205CHAPTER 9
Nerve Conduction Studies and Needle Electromyography
Devon I. Rubin and Ruple S. Laughlin
INTRODUCTION
In clinical electromyography (EMG), electrical signals from muscle fibers or nerves are recorded and analyzed to determine the function of nerves, neuromuscular junctions, and muscle fibers. In disease states, alterations in the signals on nerve conduction studies (NCSs) and/or needle EMG indicate the presence and type of disorder. In contrast to EEG where the physiologic electrical signals originating in the central nervous system are of extremely low voltage, the signals generated from peripheral nerve axons and muscle fibers are somewhat larger. As a result, external electrical artifacts less commonly interfere with the physiologic signals during NCS and needle EMG. Furthermore, advances in modern equipment, the use of common mode rejection differential amplifiers, and appropriate grounding of equipment have significantly reduced nonphysiologic artifacts. Nonetheless, artifacts such as 50- or 60-Hz signals from nearby external sources or artifacts from sources such as pacemakers or nerve stimulators still do occur and may be recorded during needle EMG. Since some of the artifacts can impede reliable interpretation of true physiologic responses or may mimic physiologic waveforms, recognition of artifacts is critical to prevent misinterpretation of a study. This chapter will review a variety of external artifacts as well as artifactual responses due to technical errors during NCS and needle EMG.
NCS ARTIFACTS
NCSs are a component of an electrodiagnostic examination during which a nerve is stimulated with an electrical stimulator and the action potentials propagating along the nerve or a muscle innervated by that nerve are recorded, usually with surface recording electrodes. Although nonphysiologic artifacts are uncommon during the performance of NCS with modern-day equipment, “artifactual” responses are frequent. Artifactual responses refer to recorded waveforms that do not accurately represent the true or optimal physiologic response, and are frequently due to technical problems in the performance of an NCS. Artifactual responses can occur due to many factors, including problems with nerve stimulation, errors in nerve or muscle recording, physiologic conditions such as low surface temperature, or the presence of anomalous variant nerve anatomy. These artifactual responses may be more difficult to identify than external nonphysiologic artifacts, as the responses obtained may look “real” but still not be the optimal responses. As a result, the presence of these artifactual responses may result in false-positive (misdiagnosis of a disease) or false-negative interpretations of a study. This section will discuss and demonstrate artifactual responses due to technical problems as well as external, nonphysiologic artifacts in NCS.
ARTIFACTS DURING MOTOR NCS
The goal of a motor NCS is to test the integrity of an individual nerve, the neuromuscular junctions, and the muscle fibers innervated by that nerve. A motor NCS is performed by electrically stimulating (and depolarizing) all of the axons within a nerve and recording the summation of all of the muscle fiber action potentials generated in the innervated muscle. This study requires appropriate placement of the recording electrodes over 206the muscle and appropriate stimulation of the nerve. Technical problems with recording and stimulating can produce artifactual and inaccurate responses (1).
Artifactual Low Amplitude—G1 Electrode Placement
This median motor NCS was recorded from a 49-year-old man with hand pain and demonstrates low compound muscle action potential (CMAP) amplitude (Figure 9.1A). In our laboratory, the normal amplitude from the elbow stimulation site is greater than 4.0 mV and in this study it is 3.2 mV. Low CMAP amplitude would be suggestive of a process anywhere along the motor unit, including loss of anterior horn cells or motor axons, a severe neuromuscular junction defect, or severe loss of muscle fibers in a myopathy. However, technical problems such as incorrect placement of the G1 (active) recording electrode can also result in low CMAP amplitude.
The ideal placement of the G1 recording electrode during a motor NCS is directly over the endplate zone. At this site, the action potentials are initiated along the muscle fibers and propagate away from the electrode along the course of the muscle fibers. Since the extracellular electrical field generated from the inward sodium ion flow during the action potential generation is negative at the site of sodium channel opening and positive on the preceding and trailing “tails” of the moving potential, a CMAP waveform recorded directly over the endplate will have an upward deflection from the baseline and appear as a biphasic, negative (upward)–positive (downward) wave. When the G1 electrode is placed along the muscle at a site away from the endplate zone, the preceding positive electrical field from the action potential moving toward the electrode will produce an initial “positive dip” in the CMAP morphology and result in a triphasic, positive–negative–positive waveform. Additionally, since the electrical field density is lower, the further the electrode is from the endplate zone, the more gradual the negative slope and the lower the amplitude of the CMAP (1).
In most cases in which the G1 electrode is not placed directly over the endplate zone, the CMAP amplitude will be lower than it should be and an initial positive deflection will be present. However, a low CMAP can occur without the “positive dip.” Therefore, a problem with G1 electrode placement should always be considered first when an unexpectedly low CMAP amplitude is recorded. In this case, the G1 electrode was moved and the amplitudes significantly increased. After the G1 electrode was moved along the muscle belly, much higher amplitude (11.6 mV) was recorded in this patient (Figure 9.1B).
Artifactual Low Amplitude—Stimulator Off Nerve
The tibial motor response was recorded from a 57-year-old woman with foot burning and a normal neurologic examination and demonstrates a much lower CMAP amplitude at the knee (8.8 mV) than at the ankle (18.3 mV) (Figure 9.2A). During a motor NCS, when all of the axons within the nerve being tested are depolarized by electrical stimulation, all of the muscle fibers innervated by that nerve will elicit action potentials. The summation of each of the muscle fiber action potentials produces the CMAP response, and the amplitude of the CMAP directly reflects the number of muscle fibers depolarized and indirectly reflects the number of axons present within the nerve. Therefore, accurate CMAP amplitude requires depolarization of all of the axons within the nerve. When the electrical current administered through the stimulator is not sufficient to depolarize some of the axons, the recorded CMAP amplitude may be lower than the actual amplitude and does not reflect the true integrity of the nerve or muscle. Artifactual low amplitudes from inadequate nerve stimulation can result from placement of the stimulator at a distance from the nerve or may occur when certain anatomic factors are present, such as limb edema, prior nerve transposition, and in limbs with a larger amount of subcutaneous tissue (1). In the most severe situation when the stimulator is located far away from the nerve being tested, no response will be recorded. In other cases, artifactual low amplitude is obtained since only a portion of the axons are depolarized. If submaximal stimulation occurs at a proximal stimulation site but not the distal site, the artifactual low proximal CMAP amplitude may mimic a conduction block.
Techniques to ensure maximum stimulation of a nerve include sliding the stimulator parallel to the expected course of the nerve to determine the highest amplitude response at low stimulus intensity (1). A higher amplitude response at a different site (without a change in the stimulus intensity) indicates closer proximity to the nerve. The stimulator is moved several times in both directions until the site of the maximum amplitude waveform is identified. At that site, the stimulus intensity is then increased until a supramaximal response is obtained. Ensuring that the stimulus intensity is increased by 5% to 10% after the waveform appears to be maximal is important to ensure that all axons have been depolarized. In this case, after sliding the stimulator and ensuring supramaximal stimulation, the CMAP amplitude increased to 13.8 mV (Figure 9.2B).
207Artifactual High Amplitude—Overstimulation
The peroneal motor NCS was performed in a 60-year-old patient with leg pain and demonstrates larger CMAP amplitude with stimulation at the knee (3.7 mV) than at the ankle (3.1 mV) (Figure 9.3A). Since motor axons within a nerve all have approximately the same diameter and degree of myelination, they all conduct action potentials at approximately the same rate. However, given slight variations in the conduction along the motor axons, when a nerve is stimulated at two different sites (such as at the elbow and axilla), there is a slight dispersion of the CMAP waveform and slight reduction in the amplitude from the more proximal stimulation site compared to the distal site. In every normal motor nerve, the CMAP amplitude at the distal site should be either slightly higher than or equal to the amplitude at the proximal site. A higher CMAP amplitude at a proximal site than distal site indicates either a technical problem or anomalous anatomic variation.
Overstimulation is one common technical problem that can result in an artifactual high CMAP amplitude (1). When too much current is administered to a nerve, the excess current will spread through the extracellular tissue and will not only depolarize the nerve of intent, but may also spread to another nearby nerve (or portion of the brachial plexus when stimulating at Erb’s point). When another nerve is additionally depolarized and muscles supplied by that nerve are in the vicinity of the G1 recording electrode, stimulation of multiple nerves may yield artifactual large CMAP amplitude due to the summation of responses of multiple nerves and the subsequent activation of muscle fibers in the vicinity of the recording electrode. Overstimulation can also change the waveform morphology and result in inaccuracies in marking the onset latencies of the waveform, yielding inaccurate conduction velocities and latency measurements. Additionally, overstimulation may mimic anomalous innervation if the amplitude of the response is higher proximally than distally.
When performing motor NCS, careful observation of the waveform as well as the visible muscle movement elicited by stimulation is important. If the waveform changes in morphology when stimulation is increased in 5% to 10% increments, or if the muscle twitch is not one expected from stimulation of the intended nerve, it is important to reassess and reduce stimulation intensity, slide the stimulator, and begin again. In this case, overstimulation at the knee led to current spread to and depolarization of the tibial nerve, in addition to the peroneal nerve. The G1 electrode over the extensor digitorum brevis (EDB) recorded action potentials from tibial-innervated foot muscles. The waveform morphologies were different and the patient’s foot was noted to change from dorsiflexion to plantar flexion as the stimulus intensity increased. When only the peroneal nerve was stimulated, the amplitudes were similar between the ankle and knee (Figure 9.3B).
Artifactual High Amplitude—Martin-Gruber Anastomosis
The median motor study was performed in a 60-year-old woman with arm numbness, and demonstrates higher CMAP amplitude at the elbow (13.5 mV) than at the wrist (8.4 mV) (Figure 9.4). When a higher CMAP amplitude at a proximal site compared to a distal site is encountered, technical factors such as submaximal stimulation at a distal site or overstimulation at a proximal site should be considered. When these technical problems have been excluded, another possible cause is anomalous anatomy. During a median motor NCS, when the CMAP amplitude at the elbow is higher than at the wrist, a Martin-Gruber anastomosis may be present. This is a common anatomic variation that consists of a communication between the median and ulnar nerve fibers in the forearm, whereby fibers that are destined to supply ulnar-innervated muscles course through the median nerve in the upper arm and proximal forearm, and “cross over” to the ulnar nerve in the forearm before innervating the destined muscles (2–5). When the crossing-over fibers supply ulnar muscles that are located in the hand adjacent to the thenar region (such as first dorsal interosseous, adductor pollicis, or abductor pollicis brevis), an artifactual high CMAP amplitude with elbow stimulation during a routine median motor NCS is recorded. This results from the summation of the volume-conducted CMAP response from the nearby ulnar-innervated muscles that are supplied through the crossing-over fibers to the “normal” median CMAP response originating from the abductor pollicis brevis and opponens pollicis. This addition of other muscle fiber action potentials results in a higher CMAP amplitude at the elbow than at the wrist (where only the true median fibers are activated).
Artifactual High Amplitude—Accessory Peroneal Nerve
The peroneal motor NCS, with recording from the EDB, was recorded from a 49-year-old man and demonstrates a higher CMAP amplitude with stimulation at the knee (4.1 mV) than at the ankle (3.8 mV) (Figure 9.5A). Anomalous innervation in the lower extremity can produce an artifactual appearing result and mimic a technical problem. In motor NCS, all of the axons within the nerve being studied should be depolarized through electrical stimulation and reflected in the resultant muscle CMAP amplitude with appropriate stimulation intensity. The CMAP amplitudes should be approximately the same at all sites of stimulation, with the proximal stimulation sites 208often being slightly lower than distal sites due to normal minor dispersion of the response. A higher proximal than distal amplitude never occurs from a physiologic basis, and only is seen with technical problems (e.g., overstimulation at the proximal site or understimulation at the distal site) or anomalous innervation. Technical factors should always be checked first, such as ensuring that the foot is dorsiflexing upon stimulation, sliding the stimulator if necessary, and excluding understimulation at the distal stimulation site.
If technical factors are excluded and the proximal peroneal motor CMAP amplitude is still higher than the distal site, the patient likely has an “accessory peroneal nerve.” This is a normal anatomic variant, present in approximately 15% to 20% of individuals, in which the terminal branch of the superficial peroneal nerve that courses along the lateral leg and behind the lateral malleolus innervates a portion of the EDB (1). As a result, some of the axons innervating some fibers of the EDB muscle are not depolarized when the peroneal nerve is stimulated at the normal distal stimulation site at the anterior ankle. An accessory peroneal nerve can easily be confirmed by stimulation behind the lateral malleolus, in which a response will be recorded from the EDB due to this anomalous pathway. This was confirmed in the patient in Figure 9.5B.
Artifactual Slowed Conduction Velocity—Incorrect Distance Measurement
This ulnar motor NCS was performed in a 35-year-old woman with hand tingling, and demonstrates a mildly slowed conduction velocity (CV) (49 m/sec, normal >51 m/sec) across the elbow (Figure 9.6A). In motor NCS, CV between two or more sites of stimulation is calculated to assess the integrity of the axons and myelin in a specific nerve segment. The CV is calculated by determining the difference in onset latencies of the recorded waveforms between the different sites of stimulation divided into the distance between the stimulation sites, usually measured in millimeters. Accurate calculation of the CV requires precise and accurate measurement of the actual course of the nerve being studied. Measurement is made by holding a measuring tape along the skin surface of the limb between the stimulation points. If the tape measure is not accurately placed along the actual course of the nerve, the recorded distance will not reflect the actual length of the nerve. When a falsely short measurement is recorded, such as when the tape measure is placed in front of the medial epicondyle rather than behind the epicondyle through the ulnar groove, the CV will be artifactual slowed and may mimic disease (1). This may occur more commonly in the ulnar nerve around the elbow, where it is more difficult to know the actual course of the nerve. The effect of measurement error is proportional to the distance between the two stimulation sites, with a much greater error the shorter the distance. In this case, when the nerve measurement was accurate, the CV was normal (Figure 9.6B).
Artifactual Fast CV—Martin-Gruber Anastomosis
The median motor NCS was performed in a 79-year-old woman with a moderate left median neuropathy, and demonstrated a CV of 75 m/sec (Figure 9.7). A normal CV of motor axons in most nerves in most patients ranges from approximately 50 to 60 m/sec. This patient had an artifactual, excessively fast CV, which is outside of the normal range expected for a patient of this age. Artifactual fast CVs can occur as the result of technical errors, such as incorrect distance measurement or overstimulation and depolarization of adjacent nerves. In this case, technical problems were excluded. What is also noted in this case is that the waveform morphology between the wrist and elbow sites differs with the elbow waveform, demonstrating a “notch” in the waveform. This is indicative of a Martin-Gruber anastomosis in the context of a median neuropathy at the wrist (6,7). An earlier example demonstrated and discussed how a Martin-Gruber anastomosis can produce a higher CMAP amplitude at the elbow than wrist in a median motor NCS, due to the addition of muscle fibers from nearby ulnar innervated muscles supplied by the crossing-over fibers. When this is present in the context of a median neuropathy at the wrist, the slowing of the conduction across the wrist delays the latencies of the “true” median responses (the portion of the CMAP that originates from the ABP/opponens pollicis). However, the portion of the CMAP that courses through the crossing-over fibers (which are located in the ulnar nerve at the wrist and are not compressed at the wrist) is not delayed. This results in a separation between the “median” CMAP and the “crossover” CMAP. Therefore, the initial onset of the waveform at the elbow does not represent the true median fibers; however, the latency marker is usually automatically placed at the onset latency by the machine which includes the faster MGA fibers bypassing the carpal tunnel, resulting in a calculated CV that will be falsely fast. This often also results in a “notched” appearance of the proximal waveform. In severe cases, there may be a complete separation of the true median CMAP and the crossover CMAP.
ARTIFACTS DURING SENSORY NCS
Sensory NCSs are performed by electrically stimulating the axons within a nerve and recording the sensory nerve action potentials (SNAPs) at another site along the nerve. Since SNAPs are much smaller than muscle fiber action potentials (or CMAPs) recorded during motor NCS, careful attention to 209technique is critical to obtain the most accurate response. Problems with nerve identification during stimulation or placement of the recording electrodes, limb temperature, and stimulus and motor artifact can all interfere with obtaining the most accurate responses.
Artifactual Low Amplitude—G1 Placement Off Nerve
This medial antebrachial cutaneous NCS was performed in a 50-year-old man with arm paresthesia demonstrating fairly low amplitude (5 μV) (Figure 9.8A). Sensory NCS record the summated SNAPs traveling along the nerve past the recording electrodes. In order to record the maximum electrical field from the travelling volley of the action potentials, the recording electrodes are placed as close as possible to the nerve. The further the G1 recording electrode is from the action potential generator, the lower the amplitude in response. This is particularly important in sensory studies where the amplitudes are much lower than motor responses. Additionally, the G1 and G2 electrodes should be placed 3.5 to 4 cm apart to maximize the amplitude of the waveform displayed through a differential amplifier. If the two electrodes are less than 3 cm apart, the SNAP amplitude decreases. In this case, the G1 electrode was located too far lateral to the actual course of the medial antebrachial nerve. Once the electrode was moved a few centimeters medially, a higher amplitude (11 μV) was recorded (Figure 9.8B).
Artifactual Low Amplitude—Limb Edema
The sural sensory NCS was performed in a 30-year-old woman with radicular leg pain and lower limb lymphedema, and demonstrated a low-amplitude response (Figure 9.9A). In most laboratories, routine NCSs are performed using surface recording electrodes placed on the skin over the nerve being studied. Since SNAPs are very low amplitude, they are much more susceptible to the effect of distance between the nerve and recording electrodes. The recording electrodes record the electrical field generated by the action potential propagating along the nerve. This electrical field is strongest immediately adjacent to the nerve and decreases further away from the nerve. Therefore, conditions that increase the distance between the skin and nerve may have a significant effect on the recorded sensory (more than motor) amplitude, producing artifactual low amplitude.
In patients with lymphedema, fluid accumulation in the subcutaneous tissue and less commonly in the muscle increases the distance between the nerve and the skin surface. This may not only impact the ability to obtain supramaximal stimulation due to the increased distance between surface shock and nerve, but also affects the recorded amplitude. Low amplitudes on NCS should be interpreted with caution in patients with lymphedema as they may not accurately reflect the integrity of the nerve. It is important to be conscientious of these limitations to avoid erroneous conclusions regarding pathology. The effects of edema are difficult to mitigate; in these cases good electrode-to-skin contact is quite important. If concern is high for a falsely low-amplitude response, needle stimulation or needle recording may be considered. In this patient, the surface stimulator was pressed more deeply into the skin to ensure that it was as close as possible to the nerve, resulting in a slightly higher amplitude (Figure 9.9B).
Artifactual Long Distal Latency—Cool Limb Temperature
This median antidromic sensory response was recorded from a 50-year-old man with hand numbness, and demonstrates a prolonged distal latency (Figure 9.10A). Although this would suggest that the patient might have carpal tunnel syndrome, the amplitude of the response is very high and the waveform morphology appears somewhat broad for a SNAP, raising the suspicion that there may be low limb temperature.
Cool limb temperature prolongs the duration of opening of the sodium and potassium channels and slows the electrotonic spread of the action potential along the nerve. This results in slowing of the conduction time along the nerve and may be seen as an artifactual prolonged distal latency (by approximately 0.2 milliseconds/°C decrease) and slowed CV (by 1.8–2.0 milliseconds/°C decrease). Additionally, both the amplitude and duration of the waveform response will be increased. The artifactual prolongation of the distal latencies due to cool limb temperature can lead to the erroneous interpretation of a study as indicative of polyneuropathy or carpal tunnel syndrome. Limb surface temperatures should always be measured prior to the performance of an NCS. The ideal temperature for the NCS should be greater than 32°C on the hand and greater than 30°C on the foot. If the limb is too cool, it should be warmed prior to the study. When the limb was warmed in this patient, the distal latency normalized (Figure 9.10B).
Artifactual Long Distal Latency—Cathode–Anode Reversal
This median antidromic NCS was performed in a 48-year-old woman without any hand numbness, and demonstrates a prolonged distal latency (normal <3.6 milliseconds) (Figure 9.11A). This could be consistent with carpal tunnel 210syndrome. However, technical factors should always be considered when an abnormal finding is encountered. During routine NCS using a bipolar electrical stimulator, the cathode (the site under which the nerve is depolarized) is placed directly over the nerve, pointed toward the recording electrodes, with the anode approximately 2 cm distant to the cathode. If the cathode and anode are inadvertently reversed, the nerve is depolarized approximately 2 cm further away from the recording electrode. This additional 2 cm of distance that the action potentials must travel along the nerve will increase the distal latency by approximately 0.4 milliseconds. In patients being evaluated for mild carpal tunnel syndrome, where prolongation of the sensory distal latency may be the only finding, inadvertent cathode–anode reversal may produce an artifactual long distal latency (a false-positive study). An additional effect of cathode–anode reversal is that a theoretical block of conduction of some of the fibers may occur as a result of hyperpolarization of axons at the anode. Anode block can consequently require a higher stimulus intensity to depolarize the axons. In this patient, when the cathode and anode were appropriately placed, the distal latency was normal (Figure 9.11B).
Artifactual Long Distal Latency—Wrong Distal Measurement
The median sensory antidromic NCS was performed in a 50-year-old man with arm pain and demonstrated a prolonged distal latency of 3.9 milliseconds(normal <3.6 milliseconds) (Figure 9.12A). One parameter assessed in each NCS is the distal latency, or time from the stimulus to the onset or peak of the recorded waveform with stimulation at a distal site. Normative reverence data for NCS are derived using specific laboratory techniques. Distal latency reference values are derived using standard distances between the stimulator cathode and G1 recording electrode. Therefore, the distal stimulation should be performed as close as possible to the standard distance. When an incorrect and excessively long distal distance is used, the actual length of the nerve segment being tested will be longer than normal. Therefore, the time for the action potentials to reach the recording electrode will increase, producing artifactual long distal latency. This could result in a false-positive study and an incorrect diagnosis in a normal patient. Falsely long distal distances can occur with imprecise measurement technique, in situations where the stimulator was moved (sliding technique) to optimally identify the nerve, or in patients with long limbs, such as a long hand. In cases where sliding resulted in a longer distal distance, the study should be repeated at a more accurate distance. In this example, the initial distance was 15 cm and, after stimulating at 13 cm, the distal latency was 3.4 milliseconds (Figure 9.12B).
Shock/Stimulus Artifact
This medial plantar NCS was recorded from a 40-year-old patient with foot pain and demonstrated prominent shock artifact, resulting in artifactual low amplitude (Figure 9.13A). Shock artifact, also called stimulus artifact, is the artifact that occurs from the spread of the stimulating current over the skin directly to the recording electrode. While shock artifact is present on every NCS, it is much more prominent in sensory than motor NCS due to the high signal amplification needed to display sensory waveforms. The shock artifact can alter the actual recorded waveform or run into the actual waveform, particularly if the distance between the stimulator and recording electrodes is short. The most common scenario is a distortion of the baseline by the shock artifact, hindering accurate marking of waveform onset for latency measurements. Methods to reduce shock artifact include minimizing the amount of electrical conducting paste placed on the stimulator as well as reducing impedance with good skin preparation prior to electrode application. Utilizing a smaller stimulating head may also limit current spread due to the closer relationship between anode and cathode. When the shock artifact does interfere with the SNAP baseline or waveform, rotating the stimulator anode around the cathode can reduce the impact of the shock artifact on the recorded response by finding a position in which the electrical field difference is the smallest, allowing the electrical stimulus to reach both the G1 and G2 electrodes to a similar degree and cancel out. When this was performed in the same patient, the onset baseline became more accurate and the amplitude increased to 20 μV (Figure 9.13B).
Motor Artifact
This ulnar sensory antidromic NCS was performed in a 50-year-old patient with hand pain, and demonstrates prominent motor artifact (arrows) (Figure 9.14A). Some sensory NCSs are performed by stimulating a mixed nerve (with motor and sensory fibers) and recording over a site where only sensory axons are present, such as over a digit in the hand. In these studies, although the intended response to be analyzed is the SNAP response, the electrical field from the muscle fibers that are also depolarized may be picked up by the recording electrodes through volume conduction through the extracellular tissue. Since sensory axons conduct at a slightly faster rate than motor axons, the SNAP will occur earlier than the motor response. However, the motor response is much larger than the SNAP amplitude and can distort or interfere with the identification and marking of the SNAP response. In cases where the SNAP is absent, a portion of the motor artifact may be misinterpreted as the sensory response. This is most commonly seen in ulnar 211antidromic studies, where the abductor digit minimi is in close proximity to the 5th digit. Motor artifact can be reduced by moving the G1 recording electrode a short distance away from the stimulator (such as over the proximal interphalangeal joint instead of the metacarpal phalangeal joint) and shielding the recording electrodes with gauze. In this case, moving the G1 electrode slightly more distal on the digit reduced the artifact (8) (Figure 9.14B).
60-Hz Artifact
This radial sensory NCS was attempted in a 70-year-old patient in the ICU and demonstrates prominent 60-Hz artifact that interfered with the recorded response (Figure 9.15A). During routine NCS, 60-Hz artifact can interfere with the recorded waveforms. This artifact is most problematic in areas where other electrical equipment is present (e.g., intravenous [IV] pumps or ventilation devices), such as a hospital room or an ICU setting. The green ground electrode applied to the patient is a common reference point that is used in differential amplifiers in EMG machines to attempt to reduce 60-Hz artifact that is common to both the G1 and G2 electrodes. However, it does not perfectly reduce the artifact. When 60-Hz artifact is prominent, high-amplitude sinusoidal waves can interfere with the identification of the true nerve response due to their inability to obtain a clear baseline, and signal distortion. Sensory NCSs are much more affected by such artifacts due to their filter settings and low amplitude, requiring greater amplification (which also amplifies the 60-Hz artifact). Methods used to reduce the artifact include the use of a larger ground electrode and reducing the possible sources as much as possible (e.g., turning off lights, unplugging a hospital bed). When a larger ground was used and equipment was turned off, a radial response was able to be recorded (Figure 9.15B).
ARTIFACTS DURING REPETITIVE NERVE STIMULATION
Repetitive nerve stimulation (RNS) is an NCS technique that consists of administering several electrical stimuli at various rates (2–50 Hz) to assess the adequacy of neuromuscular transmission. In normal persons without impaired neuromuscular transmission, supramaximal stimulation at low rates of a nerve results in depolarization of all of the innervated muscle fibers; therefore, the CMAP amplitude and area remain the same with each stimulus. In some neuromuscular junction diseases, such as myasthenia gravis, the amplitude and area of the CMAP decrease with each stimulus, since there is a reduction in the endplate potential at some of the postsynaptic junctions, resulting in an insufficient depolarization to produce action potentials in some of the muscle fibers. The presence and degree of “decrement” in the CMAP amplitude and area helps to diagnose neuromuscular junction diseases. RNS studies are difficult and frought with technical factors that can produce artifactual false decrement (false-positive studies) or, rarely, artifactual normal (false-negative) studies. Technical problems that produce artifactual responses include submaximal stimulation, unstable baselines due to muscle contraction or recording electrode movement, and cool limb temperature (which improves decrement).
Artifactual False Decrement—Stimulator Movement
The spinal accessory RNS study was performed in a 60-year-old man with weakness and appears to demonstrate a maximum amplitude decrement of 52% between the first and fourth stimuli (Figure 9.16A). During RNS, the stimulator must remain in a completely fixed position over the nerve to ensure reliable and reproducible responses. Movement or sliding of the stimulator off the nerve during each stimulus results in a variability and possibly a reduction in the number of axons that are depolarized, thereby resulting in lower CMAP amplitude. This drop in amplitude can mimic real decrement. The pattern of true, physiologic decrement from a neuromuscular junction disorder is a maximum decrease in amplitude (or area) between the first and second stimuli, with less of a drop with each subsequent stimulus. If the pattern of amplitude reduction is not physiologic (such as the largest drop occurring between the third and fourth stimuli), technical factors are likely. The stimulator should be carefully held in a stable position on the skin and supramaximal nerve stimulation should be administered to reduce this artifactual response. In this case, when another set of four stimuli were given and careful attention was made to holding the stimulator still, no significant decrement (2%) was seen (Figure 9.16B).
Artifactual False Decrement—Muscle Contraction
This ulnar RNS study was performed in a 50-year-old man with weakness and appears to demonstrate a maximum amplitude decrement of 11% between the first and fourth stimuli (Figure 9.17A). During RNS, the recording electrodes must remain in a completely fixed position over the muscle to ensure reliable and reproducible responses. Even slight movement of the G1 electrode along the muscle may change the position of the electrode relative to the endplate 212zone, affecting the morphology and amplitude of the CMAP. This may result in CMAP variability with each stimulus and may mimic decrement. Movement of the electrode can occur when the patient tenses up or contracts the muscle during stimulation. If the morphology of the CMAP waveforms with each stimulus varies, or the pattern of apparent decrement is not physiologic, technical factors are likely. In this case, when the study was repeated and the patient remained relaxed, no decrement was seen (Figure 9.17B).
Artifactual Normal Study—Cool Limb Temperature
This ulnar RNS study was performed in a 42-year-old woman with weakness and positive acetylcholine receptor antibodies, and demonstrated no decrement (Figure 9.18A). The patient was noted to have a cool hand temperature of 27.8°C. Cool limb temperature improves neuromuscular transmission by prolonging the open time of the acetylcholine receptors as well as lowering the activity of acetylcholinesterase at the neuromuscular junction, thereby increasing the safety margin of neuromuscular transmission (9–11). Therefore, RNS performed on a cool limb in a patient with myasthenia gravis may improve the actual decremental response and result in an artifactual normal (false-negative) study. Warming the limb has been shown to increase the degree of decrement and the sensitivity of repetitive NCS in myasthenia gravis. In this patient, when the limb was warmed to 32.2°C, a reproducible 8% decrement was recorded (Figure 9.18B).
ARTIFACTS DURING NEEDLE EMG
During needle EMG, a recording needle electrode is inserted into a muscle and the electrical signals generated from muscle fibers are recorded and amplified. The presence of various abnormal signals from fibers and alterations in the motor unit potential (MUP) morphologies help to determine whether there is an underlying neuromuscular disorder. The electrical signals generated from muscle fibers are very small in amplitude, in the range of 10 μV to 1 to 2 mV, and therefore must be amplified for interpretation. Due to the small amplitude of the signals, various external artifacts and other factors can interfere with the recordings. To reduce the contribution of external artifactual signals, all electrical activity recorded from an “active” wire at the tip of the needle electrode is compared to the activity recorded at a reference site at the same point in time. Through the use of a differential amplifier in the EMG machines, the difference in the voltages between the active and reference sites is amplified and displayed. Therefore, artifactual signals that are recorded equally at the active and reference sites will essentially be cancelled out and minimized. This cancellation effect is higher when needle EMG is performed with a concentric needle electrode (in which the reference electrode is the needle shaft) compared to a monopolar needle electrode (in which the reference electrode is a separate needle or surface electrode placed at some other site on the limb). However, because the signals are recorded to a slightly different degree at the active and reference sites, artifactual signals are not completely eliminated and artifacts can be recorded with both types of electrodes. The use of a ground electrode can help to minimize, but not completely eliminate, external artifacts, by serving as a common reference for the active and reference recording sites. When the ground electrode is not well attached to the patient, external artifacts are more likely to be recorded.
In contrast to external artifacts, artifactual responses can occur from physiologic factors (such as abnormal movements or poor relaxation), which can interfere with the recordings and affect the ability to adequately assess for abnormal waveforms. Similarly, technical factors, related to equipment or technique of the needle examination can interfere with the recordings and lead to misinterpretation of the recordings. This section will provide examples of physiologic, technical, and external artifacts during needle EMG.
PHYSIOLOGIC ARTIFACTS
Tremor
This waveform was recorded from the pronator teres in a 70-year-old man with arm pain (Video 9.1). The recording demonstrated bursts of MUPs that recur repetitively in a regular firing pattern. Each burst consists of a different group of MUPs, as evident by the changing morphology of each burst, due to central activation of different anterior horn cells. This type of regular burst firing of groups of different MUPs is characteristic of a tremor discharge. In many cases, a visible tremor will be evident to the electromyographer during the study; however, sometimes subtle tremor may not be visible. Tremor bursts may appear similar to myokymic discharges or polyphasic MUPs, although the burst of a myokymic discharge consists of the same MUP firing within the group and polyphasic MUPs have spikes that are time-locked to the main spike and fire in a semi-rhythmic firing pattern. The presence of tremor discharges can interfere with the ability to assess for spontaneous discharges, such as fibrillation or fasciculation potentials.
Poor Relaxation
The recording was made from the thoracic paraspinal muscles in a 28-year-old man who was referred for a possible myopathy (Video 9.2). The recording was made with the patient in the lateral decubitus position with attempted 213relaxation of the paraspinals. During assessment for spontaneous activity, continuous activity from voluntary MUPs was recorded, despite attempts to relax the muscle. The presence of the MUPs makes it difficult to assess for fibrillation potentials. Poor relaxation can occur when patients are in discomfort or tense, have certain neurologic disorders such as spasticity or movement disorders, or in certain muscles that are difficult to relax (such as paraspinals, diaphragm, or genioglossus).
TECHNICAL ARTIFACTS
Decreased Insertion Activity
This recording was made from the anterior tibialis in a 76-year-old man with complete foot drop from an old, severe peroneal neuropathy (Video 9.3). During needle examination of each muscle, examination of a resting muscle is performed to assess for any abnormal spontaneous discharges, such as fibrillation potentials. Needle movements within a muscle typically induce a brief burst of electrical activity as the needle irritation of the fibers initiates action potentials along the fibers (insertional activity). Normal insertional activity consists of a burst of spikes that lasts only as long as the needle electrode is moved, and ceases immediately after needle movement is halted. In cases where muscle tissue has been replaced by fat or connective tissue, no action potentials can be elicited and needle movement will initiate little or no electrical activity, known as decreased insertional activity (12). Decreased insertional activity is an abnormal finding that can produce an artifactual appearing response during assessment of a resting muscle. While it is often seen in severe disease processes, it can also occur from technical factors, such as poor needle connection to the cable or when the preamplifier has not been turned on.
Low-Frequency Filter—Too High
This recording was made from the deltoid of a 35-year-old man with fatigue, and demonstrates a short duration, low appearing MUP (Video 9.4). Every EMG waveform is composed of signals of a wide range of frequencies, typically ranging from 2 Hz to 30 kHz. Various frequency signals compose different parts of the waveform, with the higher amplitude spike component composed of higher frequency waves and the lower amplitude “tails” composed of lower frequency waves. In order to minimize the effect of extraneous electrical noise that may distort the physiologic signals, amplifier filters are applied to all signals prior to signal amplification in EMG machines. During routine EMG studies, the low-frequency filter (LFF) is usually set to 20 to 30 Hz. Increasing the LFF has the effect of reducing the amplitude and contribution of the lower frequency components of the MUP, which mainly compose the preceding and trailing “tails.” Filtering these components results in artifactual short duration and lower amplitude MUPs which can mimic a myopathy. When the LFF was decreased to 20 Hz from 2,000 Hz, the MUP duration normalized (Video 9.5).
LFF—Too Low
This recording was made from the first dorsal interosseous at rest in a 52-year-old woman with hand numbness (Video 9.6). The recording demonstrates prominent instability or “swaying” of the baseline during assessment of insertion activity. During routine needle EMG recording, the default LFF is typically set at 20 to 30 Hz. Decreasing the LFF has the effect of allowing more of the lower frequency components of the potentials to contribute to the waveform and results in an increase in slow movement artifact and instability of the baseline. During MUP assessment, a lower LFF allows for incorporation of more of the distant muscle fiber action potentials in the motor unit to be recorded, resulting in an increase in the baseline–baseline duration of the MUP. When normative MUP duration data were collected in the past, the LFF was set at 2 Hz. Therefore, when quantitative EMG is performed and the results are compared to normative data, the LFF should be reduced to 2 Hz for accurate comparison. However, lowering the LFF also introduces additional low-frequency waveforms, such as sweat artifact or artifact from slight movement of the needle, which reduce the stability of the baseline. In this patient, increasing the LFF from 2 to 20 Hz resulted in a more stable baseline (Video 9.7).
Too Rapid Needle Movements
This recording was made from the biceps of a 42-year-old woman with hand numbness (Video 9.8). The recording was made during assessment of insertion and spontaneous activity in the muscle at rest, particularly to assess for fibrillation potentials. Each burst of insertion activity was elicited during brief needle movement. In this example, the needle was constantly being moved with very minimal pauses between needle movements. Although this technique does not produce true artifactual recordings, it may result in artifactual false interpretations since slowly firing spontaneous waveforms (e.g., fibrillation potentials or fasciculation potentials) may be missed. This can be corrected by ensuring pauses of at least 1 to 2 seconds after each needle movement during assessment of a resting muscle (12).
214EXTERNAL ARTIFACTS
60-Hz
This artifact was recorded from the anterior tibialis in a 65-year-old man with generalized weakness in the ICU (Video 9.9). 60-Hz artifact is the most common type of artifact recorded during needle EMG. Although this artifact does not resemble any physiologic artifact, when it is prominent it may interfere with the baseline recordings and make it more difficult to assess for other spontaneous discharges or MUP abnormalities. 60-Hz artifact is more prominent in environments with a large amount of electrical equipment (e.g., ICU), but can occur in any environment. Sometimes, just a cellphone or tablet plugged into an outlet in the EMG examination room can produce prominent 60-Hz artifact. It can be minimized by using a larger ground electrode, unplugging as much of the nearby equipment as possible, and ideally using a dedicated earth ground for the EMG equipment.
Pacemaker
This waveform was recorded from the thoracic paraspinal muscles in a 75-year-old patient with back pain (Video 9.10). This waveform is a single-spike waveform that fires repetitively in a regular pattern and has the sound of a ticking clock. This waveform is a cardiac pacemaker artifact. A pacemaker artifact typically appears as a single spike, usually firing in a regular pattern at a rate of approximately 1 Hz. The regular firing pattern of a single spike may mimic a fibrillation potential. Although a pacemaker artifact is most often recorded in muscles close to the pacemaker, such as thoracic paraspinals, it can be recorded from more distant limb muscles as well. In contrast to a true fibrillation potential, a pacemaker artifact does not change or disappear with needle movement and the same spike firing at the same rate is often found in more than one muscle. Additionally, the square wave morphology of the pacemaker artifact produces an artificial sound that is subtly different from a fibrillation potential.
Pacemaker (With Fibrillation Potentials)
This example was recorded from the thoracic paraspinals in a 69-year-old man with weakness (Video 9.11). This recording demonstrates a run of positive waveform fibrillation potentials. However, the larger amplitude single spike is a pacemaker artifact and fires at a regular rate at just under 1 Hz. This pacemaker artifact could easily be confused with a single-spike form fibrillation potential.
Transcutaneous Electrical Nerve Stimulator
This discharge was recorded from the tensor fascia lata muscle in a 50-year-old patient with leg pain (Video 9.12). The discharge is a recurrent single spike that fires at a rate of approximately 60-Hz. The interspike intervals between the spikes are completely fixed and do not vary, although occasionally a spike will drop out. This artifact was generated from a transcutaneous electrical nerve stimulator (TENS) unit. The fast, unchanging rate gives the discharge an artifactual sound. This discharge would not likely be misinterpreted as a physiologic waveform, although the fast, unchanging rate could appear somewhat similar to a complex repetitive discharge.
Radiofrequency Artifact
This artifact was recorded from the tensor fascia lata of a 30-year-old patient with leg pain (Video 9.13). Although the needle electrode was in the subcutaneous tissue, radiofrequency artifact was recorded. Visually, the artifact is very low amplitude and high frequency, and appears similar to subcutaneous tissue. However, the sound of the radio broadcast (discussing the stock market!) can be easily heard. There is also low-amplitude 60-Hz artifact as well.
Gastric Stimulator
This artifact was recorded from the iliopsoas of a 55-year-old woman with a gastric stimulator (Video 9.14). A single positive waveform fires repetitively at a rate of about 15 Hz with a very regular, unchanging pattern. The waveform then pauses briefly before firing again. This could potentially be misinterpreted as a myokymic discharge, but the unchanging rate and morphology of the single spike indicates that this is an artifact.
Deep Brain Stimulator
This artifact was recorded from the deltoid in a 68-year-old patient with Parkinson’s disease (Video 9.15). This high-frequency artifact is composed of two spikes firing at rates of approximately 200 Hz, with one spike firing slightly slower (which appears to be moving to the right) than the other. This artifact was generated from a deep brain stimulator (DBS) device. Although 215this artifact does not mimic any physiologic EMG waveform, its prominence can interfere with recording of smaller waveforms (such as fibrillation potentials) or MUP assessment.
Printer Artifact
This artifact was recorded during needle examination of the medial gastrocnemius (Video 9.16). The discharge begins with a low-amplitude spike firing at about 12 Hz followed by the addition of a very high-pitched, high-frequency background artifact firing at over 500 Hz. This artifact was recorded during the printing of a waveform from the EMG machine in the exam room.
Text Artifact
This waveform was recorded while assessing spontaneous activity in a resting muscle (the first dorsal interosseous) in a 40-year-old patient with hand numbness (Video 9.17). After a brief period of normal spontaneous activity, a repetitive spike is initiated, firing first at 60 Hz, and then suddenly shifting to a slower rate 25 Hz. The interspike intervals between the spikes remain completely fixed and stable. This could have the appearance and sound of a complex repetitive discharge. The discharge occurred at the same moment that the electromyographer received a text message on a smartphone.
CONCLUSIONS
External artifacts and artifactual responses due to technical factors are common in clinical EMG. Recognition of these artifactual responses is critical to ensuring accurate interpretation of the NCSs and needle EMG findings. When identified, the electromyographer must take the appropriate steps to reduce or resolve the artifactual response and ensure technically reliable studies.
VIDEOS
Video 9.1: Tremor producing an electrophysiologic discharge in a resting muscle.
Video 9.2: Artifactual “active” baseline in a resting muscle due to poor muscle relaxation.
Video 9.3: Decreased insertion activity due to severe muscle atrophy.
Video 9.4: Artifactual short duration, low-amplitude motor unit potentials due to low frequency filter set too high (2000 Hz).
Video 9.5: Same motor unit potentials as Video 9.4, now with normal duration and amplitude when low frequency filter set at 20 Hz.
Video 9.6: Unstable baseline sway due to low frequency filter set too low (2 Hz).
216Video 9.7: Normal baseline when low frequency filter set to 20 Hz.
Video 9.8: Rapid needle movements in a resting muscle obscure the baseline, making it difficult to assess for slow spontaneous discharges.
Video 9.9: 60-Hz artifact present during needle EMG.
Video 9.10: Pacemaker artifact recorded during needle examination of the thoracic paraspinal muscles.
Video 9.11: Fibrillation potentials present with a pacemaker artifact.
Video 9.12: TENS unit artifact recorded during needle EMG.
Video 9.13: Radiofrequency artifact recorded during needle EMG.
Video 9.14: Gastric stimulator artifact recorded during needle EMG.
Video 9.15: Deep brain stimulator artifact recorded during needle EMG.
Video 9.16: Printer artifact recorded during needle EMG.
Video 9.17: Smartphone text artifact recorded during needle EMG.
217
FIGURE 9.1: (A) Artifactual low-amplitude median motor CMAP due to G1 electrode placement away from the end plate zone. (B) Normal amplitude is noted after moving the G1 electrode over the end plate zone.
CMAP, compound muscle action potential.
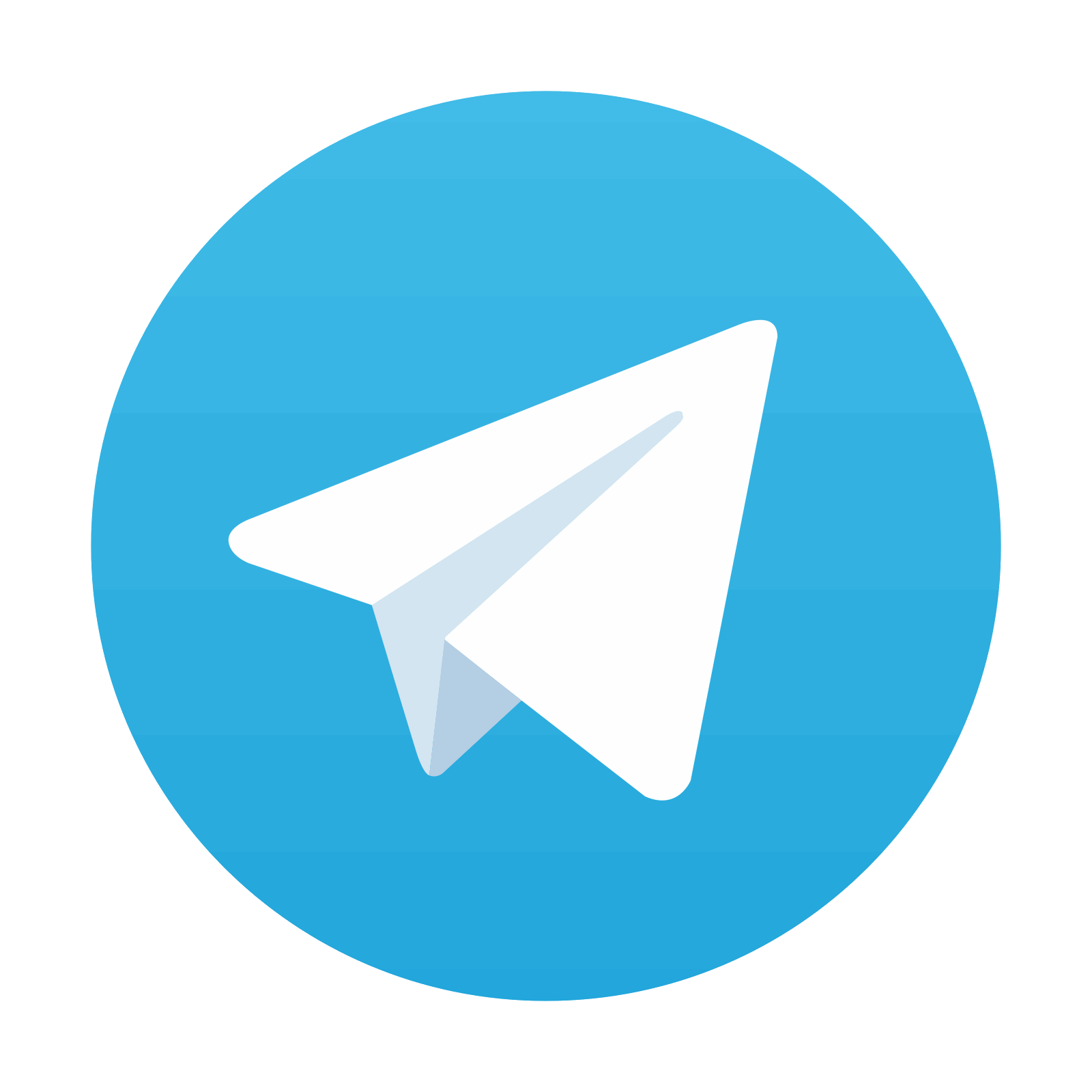
Stay updated, free articles. Join our Telegram channel

Full access? Get Clinical Tree
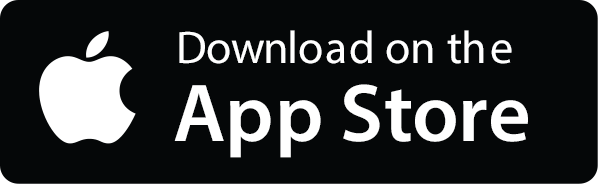
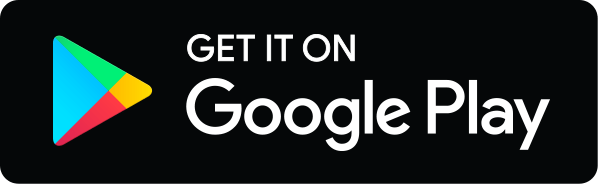