Anatomical organization of a peripheral nerve . (Reproduced with permission from Sunderland [10])
Conduction velocity in nerve fibers of different types
Fiber type | Function | Average axon diameter (mm) | Average conduction velocity (m/s) |
---|---|---|---|
Aα | Motor nerves, primary | 15 | 100 (70–120) |
Muscle-spindle afferents | |||
Aβ | Mechanoreceptor afferents | 8 | 50 (30–70) |
Aδ | Temperature and pain afferents | <3 | 15 (12–30) |
C | Pain afferents | ~1 | 1 (0.5–2) |
Sympathetic postganglionic fibers |
Peripheral Nerve Injuries
Peripheral nerve injuries can result from a variety of mechanisms. A recent retrospective review of 1019 operative brachial plexus lesions showed that the majority of lesions resulted from stretch or contusion and were in continuity. A smaller proportion resulted from tumors, gunshot wounds, and transection due to sharp laceration [6]. The management of injuries from sharp laceration and blunt transection is relatively straightforward, consisting of primary repair within 72 h and 2–3 weeks, respectively [7, 8]. However, lesions found in continuity pose several confounding variables that require extensive preoperative evaluation and planning [6, 7, 9]. Intraoperative neurophysiological assessment of peripheral nerve lesions is useful in determining continuity and establishing a treatment plan.
Classifications of Injuries
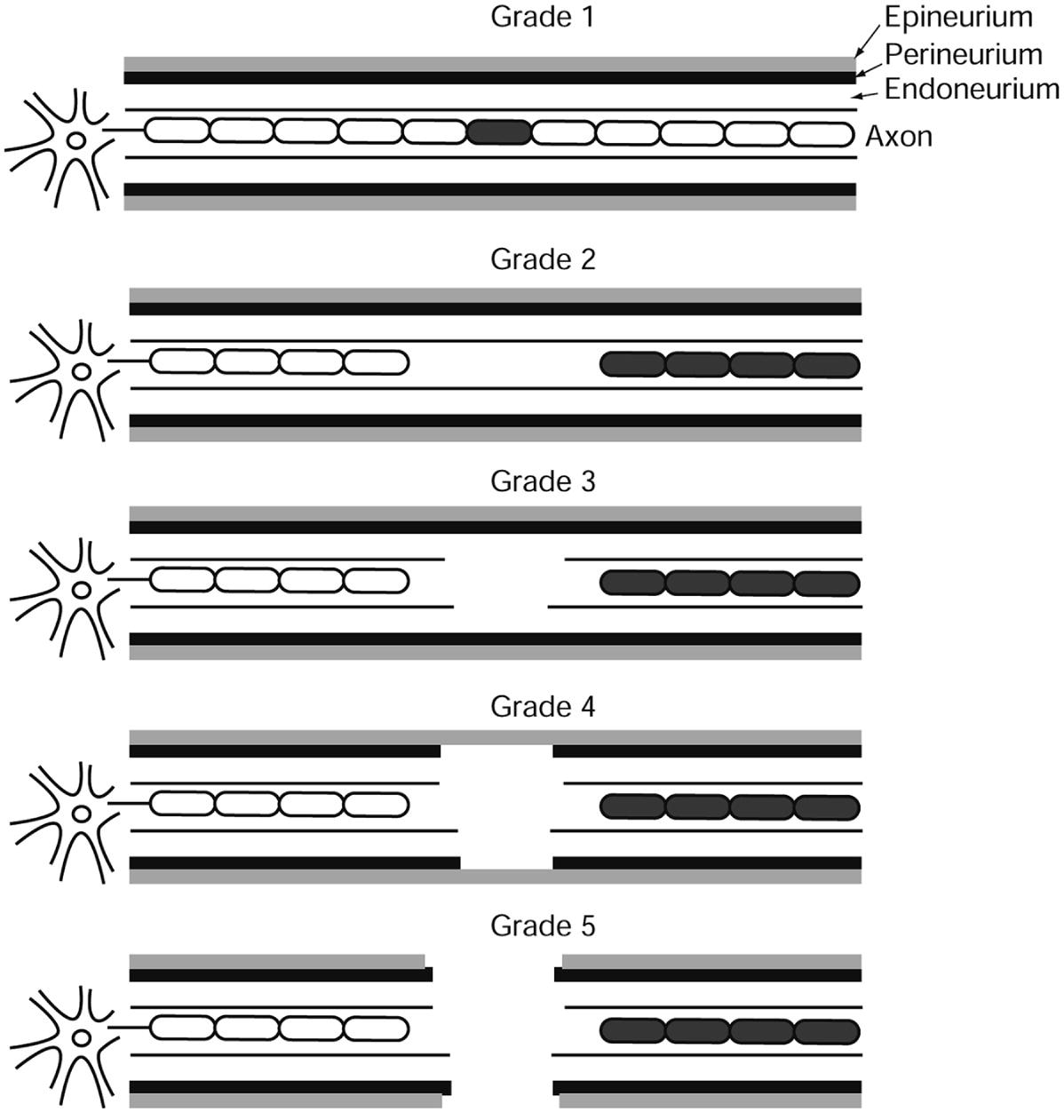
Illustration representing Sunderland grading and showing the various degrees of conduction block and support structure changes inherent to each grade of injury. (Adapted with permission from Sunderland [2])
Neurapraxia is the mildest form of injury. It is defined as partial or complete conduction failure without any structural changes in the support structures of the nerve [10]. This type of injury can also be classified as Sunderland grade 1. Etiology of this type of lesion can be due to excessive stretch, heat, or compression. The affected nerve is able to regain full function within several hours to days [1].
Axonotmesis is defined as the interruption of nerve axons without damage to its supporting connective tissue or glial structures. This is a more significant injury and can also be identified as Sunderland grade 2 [11]. Injuries such as this can also result from excessive stretch, compression, or pinching [1]. Lesions that occur distally to the cell body result in Wallerian degeneration of axonal components distal to the lesion. Wallerian degeneration is defined as the degenerative changes that occur in a segment of a nerve fiber when continuity with its cell body is lost [1]. Wallerian degeneration begins immediately after the lesion has occurred and is usually complete within 48–72 h after the injury. It is important to note that the nerve may still be able to conduct impulses within the first 24–72 h after the injury [1, 11]. Intraoperative assessment in this immediate time frame, therefore, may be inaccurate.
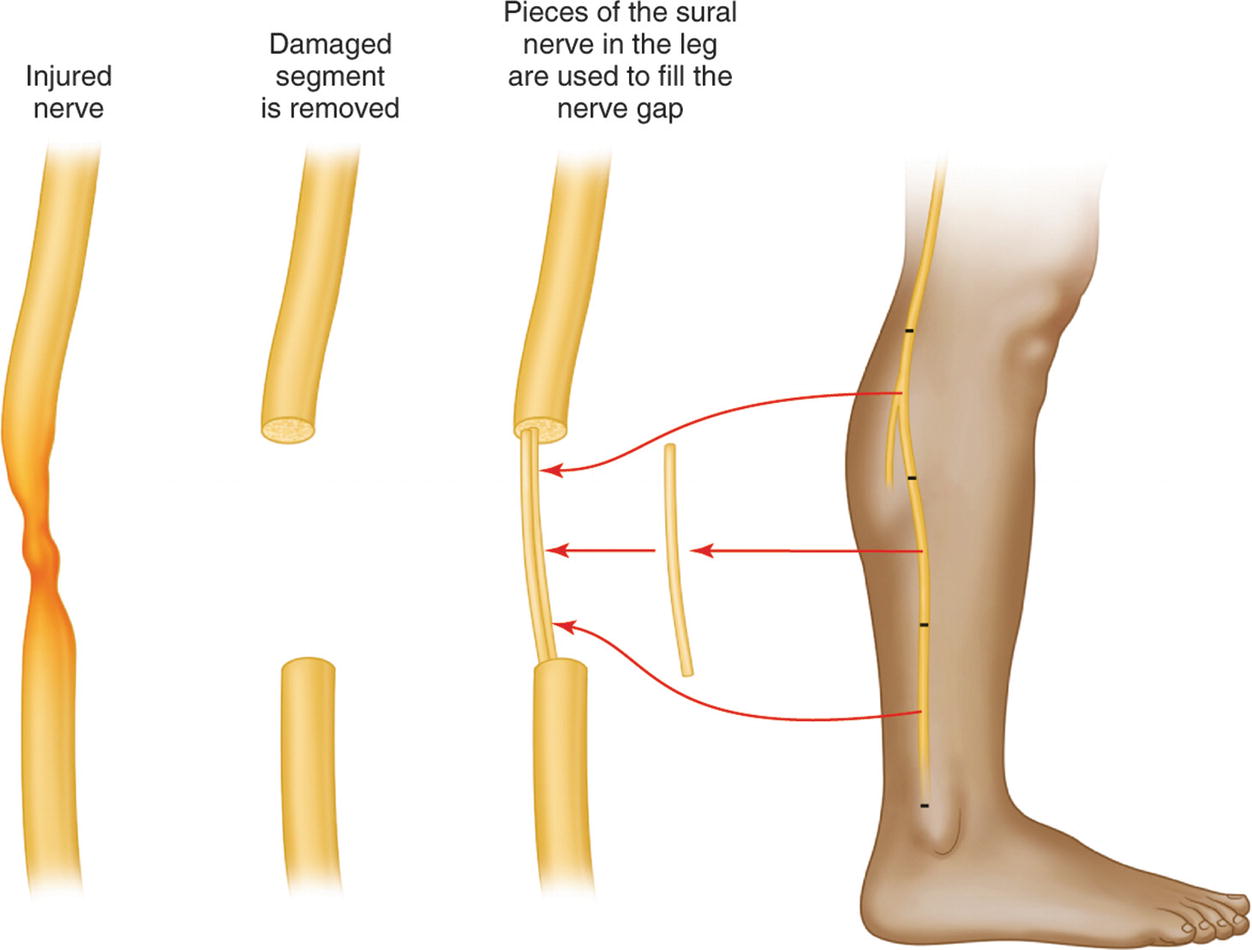
An example of using the sural nerve to graft two segments of an injured peripheral nerve
When considering the degree of neuronal injury, it is important to also understand the basic physiology of neuronal regeneration. Nerve regeneration is a complex process that is very different in humans compared to lower mammals, which have much greater regenerative capabilities [13–15]. As mentioned earlier, axonal components distal to the lesion undergo Wallerian degeneration , while the proximal part seals off at the point of division [12]. The proximal sealed portion will produce multiple sprouts of growing neurites within approximately 36 h of the injury. Regeneration occurs at a speed of approximately 1 mm/day [1]. This increase in growth can actually result in a greater concentration of axons distal to the lesion compared to proximal axon counts [12]. However, these growing axons have a much smaller diameter with distinct electrical properties [16–18]. They have much slower conduction velocities relative to normal nerves [12]. They also have significantly higher electrical thresholds relative to normal nerves. Effective regeneration is characterized by some fibers increasing in diameter, while other finer fibers regress and die. Axons must reach a critical diameter in order to produce a useful motor unit. If the developing small-caliber fibers do not successfully increase in diameter, there is low probability that they will be able to form a meaningful connection with the corresponding muscle [12, 13, 15]. Thus the presence of numerous fine fibers may be indicative of either active early-stage regeneration or later-stage failed regeneration.
Preoperative Evaluation of a Peripheral Nerve Injury
Crum et al. detail four critical questions that should be addressed when evaluating all peripheral nerve injuries [19]. First, it is obviously vital to identify whether the problem is truly neurologic. Poor patient effort, pain, and the subjective nature of sensory examinations can decrease the accuracy of clinical evaluations. Next, it is important to localize the specific nerve that is affected. This is largely dependent on clinician knowledge of peripheral nerve anatomy and dermatome distribution [19]. The clinician must then try to identify where the lesion is located along the anatomical course of the nerve. Lastly, it must be determined whether the lesion is complete or not. As discussed previously, it is possible that lesions in continuity may be undergoing axonal regrowth that is not yet detectable via clinical examination alone. Prior to the advent of electrophysiological testing, surgeons had to rely on visual inspection to determine a course of treatment. Today, real-time electrophysiological recordings can provide an accurate diagnosis of the lesion, allowing the surgeon to formulate a well-informed treatment plan. Generally, lesions found to be in continuity are observed for subsequent regeneration, while lesions in which there is no continuity are grafted [6, 20].
Recording Nerve Action Potentials
Peripheral nerve lesions that result in axonal sprouting can form an accumulation of misdirected neurites known as a neuroma. Neuromas can be small or large and cause compression of the nerve resulting in even more injury. Frequently, functionally regenerating axons may pass through the neuroma on their way to reestablish connections with their targets. These are known as neuromas in continuity. Such neuromas in continuity may need to undergo neurolysis so the regenerative process may continue but do not require grafting. Neurolysis is the surgical freeing of the nerve from inflammatory adhesions and resulting traumatic neuroma. The concept of diagnosing a neuroma in continuity is a simple one; stimulate a peripheral nerve proximal to a lesion and use electrodes to monitor for a response distal to the lesion. Intraoperative monitoring can be accomplished using relatively simple and inexpensive equipment. The majority of commercially available EMG machines are able to effectively detect compound nerve action potentials (CNAPs) . It has been estimated that a minimum of 4000 fibers are needed to produce a clear CNAP [21].
Since the size of most CNAPs is considerably larger than the typical evoked potentials recorded in operative monitoring, the process of signal averaging is not required [21]. The amount of stimulation required is dependent on the size of the nerve being studied. It is recommended that the stimulator be able to produce short pulses of 0.02–0.05 ms and intensities of up to 70 V [12]. The use of short-duration pulses helps to identify the type of fibers being stimulated. Fine fibers of regenerating axons and other small-diameter fibers are much less sensitive to short-duration impulses compared to healthy nerves [21]. Stimulation of nerves in short duration through electrodes in direct contact has been proven to be both safe and effective [21]. Longer-duration stimulation may result in electrical burns or other iatrogenic injury [21].
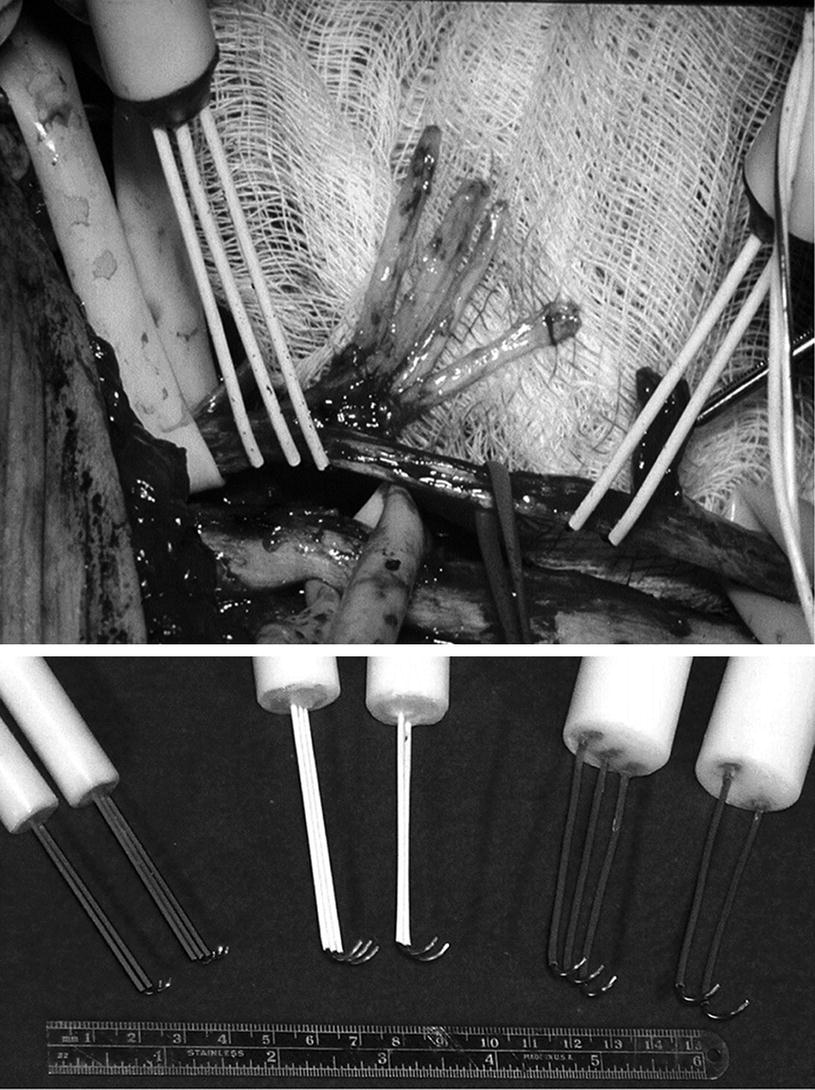
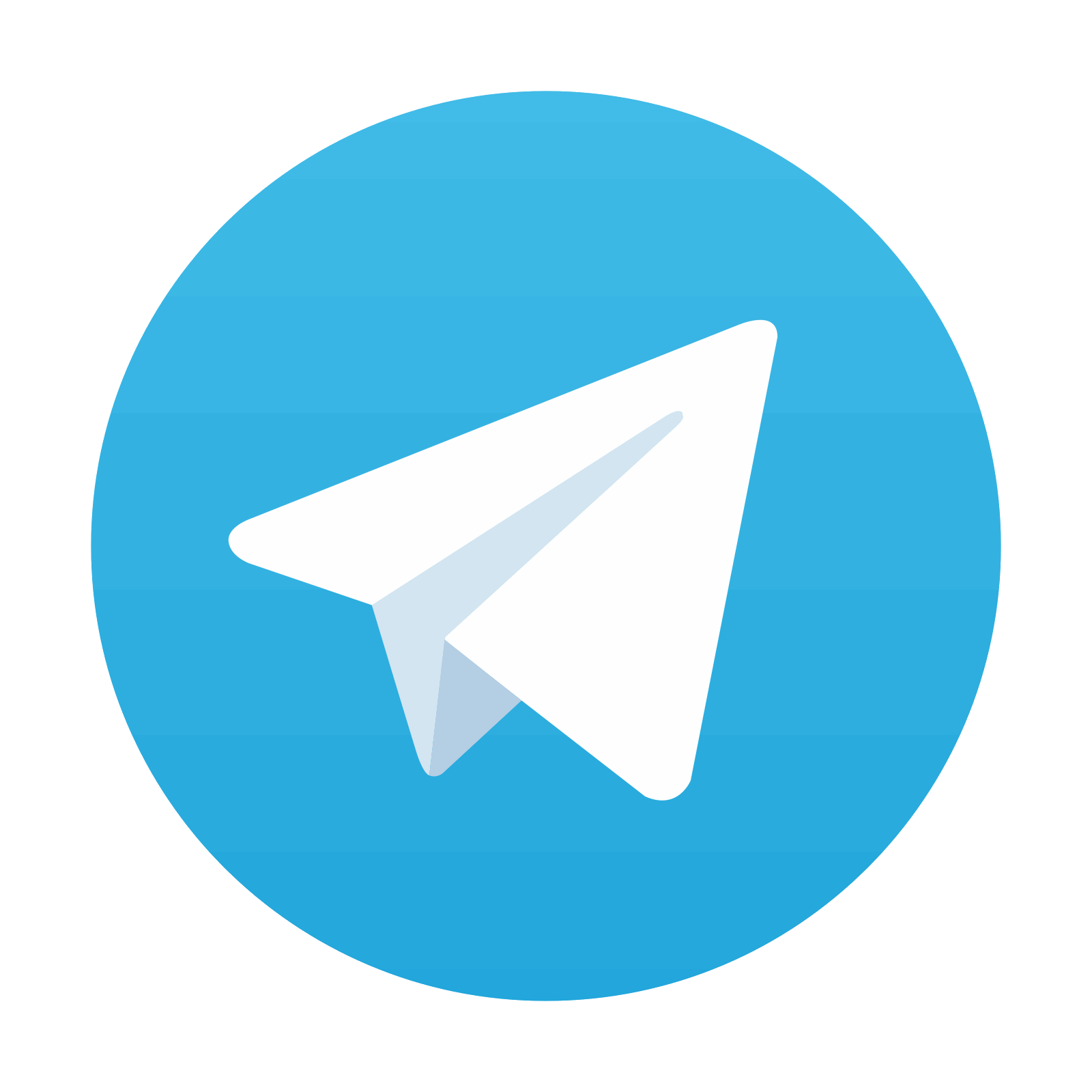
Stay updated, free articles. Join our Telegram channel

Full access? Get Clinical Tree
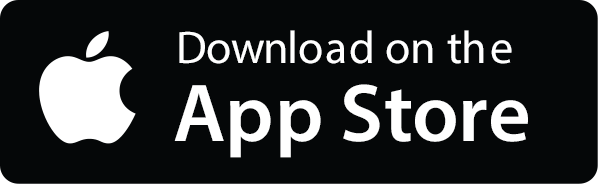
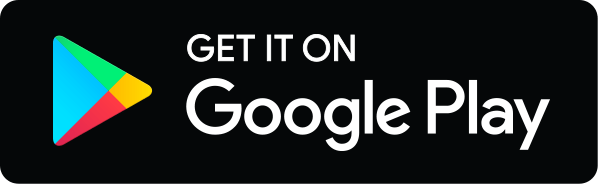