34 Nerve Repair with Conduits An 18-year-old man presented to the neurosurgery clinic ˜3 weeks after a self-inflicted laceration to the left wrist. He noticed immediate thumb weakness as well as numbness in the hand and all fingers except for the fifth digit. At the time of injury he was evaluated in a local emergency room where the wound was washed and closed. An orthopedics consultant reported no tendon injury but expressed concern for possible nerve injury. Against medical advice, the patient left the hospital prior to his evaluation by the hand surgeon. At the time of presentation to the neurosurgery clinic he noted some improvement in the numbness at the base of the palm but denied any other change in symptoms. On examination, his sensation was altered to absent in the median nerve distribution including the radial aspect of the ring finger. Motor examination revealed absent function in the left abductor pollicis brevis and opponens pollicis with preservation of the flexor pollicis longus, flexor digitorum profundus, and flexor digitorum superficialis. Electrodiagnostic examination 2 days prior to his appointment revealed a left median motor and sensory mono-neuropathy of moderate to severe degree. Figure 34–1 Intraoperative photographs of the wrist demonstrating (A) the initial laceration and (B) the initial exposure to locate the median nerve. Both the scarred median nerve in the area of the injury (arrow) and the normal median nerve in the area of the carpal tunnel (arrowhead) are demonstrated. Due to his history and physical and electrodiagnostic examination findings, he was taken to the operating room for exploration approximately 6 weeks after the injury. The distal volar forearm laceration was reopened and explored. The palmaris longus tendon was identified and appeared to be completely transected. The median nerve was not immediately identifiable due to dense scar. For this reason, a short linear incision was made in the carpal tunnel region to identify the median nerve and follow it proximally to the scarred area (Fig. 34–1). Although the nerve was found to be grossly in continuity in the area of injury, the superficial aspect of the nerve was divided. Intraoperative electrical nerve stimulation was performed. Neither compound motor action potentials (CMAPs) nor nerve action potentials (NAPs) could be identified from or across the median nerve, suggesting a functional transection. The nerve was then sharply transected with a knife in the area of injury. Pure fibrous connective tissue without fascicular architecture was seen. The nerve was sectioned serially until a healthy-appearing fascicular pattern was identified both proximally and distally (Fig. 34–2). After sectioning, the gap between the nerve ends was noted to be ˜2 cm. A 2 cm x 7 mm collagen conduit (Neuragen,Integra Neurosciences, Plainsboro, NJ) was used for repair. The nerve repair was performed with two interrupted 8–0 nylon sutures placed through the nerve guide then through the epineurium and then back through the nerve guide to allow each nerve end to be inserted ˜2 mm into the nerve tube without excessive tension (Fig. 34–3). The nerve guide was filled with saline and the wound was closed in layers. The patient was then splinted in a mildly flexed position to remove tension from the repair site. He was discharged without complication. He returned to the clinic 1 month after surgery for wound care but did not return for his long-term follow-up appointment. Figure 34–2 (A) Intraoperative photographs of the median nerve with surrounding scar on initial exposure and (B) after neurolysis and serial transection to identify healthy fascicles. Median nerve injury repaired with nerve conduit Figure 34–3 (A) Intraoperative photographs during and (B) after conduit placement (arrowhead) (C) Illustration depicting placement of the conduit with a single horizontal mattress suture on each side. The presented case demonstrates the need for suitable conduits to bridge the gap across areas of nerve damage. The first documented attempts at nerve repair using conduits date back as far as 1608. Early results were uniformly poor, despite the use of various materials to aid in repair.True strides in peripheral nerve repair were not made until the 1960s when Millessi demonstrated the importance of a tension-free repair and popularized meticulous alignment of nerve fascicles using microsurgical techniques. The logical corollary to tension-free repair is the need for material to bridge the gap between the two injured ends of a nerve that otherwise cannot be approximated without tension. Despite serving as the initial standard for repairing a nerve gap, the use of autologous nerve graft tissue is associated with significant complications, morbidities, and suboptimal results, providing the rationale for the development of other forms of conduits. The use of autologous nerve grafts is limited due to the characteristics of the donor site, the graft site, and the nerve graft itself. Donor graft harvest requires a second incision and thus surgery at an area of the body without disease. At the same time, normally innervated tissue is denervated in the process. Furthermore, surgery at this site prolongs surgical time and can lead to local infection or painful neuroma formation. At the graft site, the suture lines allow for the possibility of formation of a neuroma-in-continuity. These suture lines can also encourage intraneural fibrosis, which may impede axonal regeneration. Additionally, local scar tissue formation may lead to compression and loss of function of the graft. Nonvascularized autografts are limited by the vascular quality of the wound bed and the size of the grafted nerve because the possibility of central and segmental necrosis of the graft can limit the size of the nerve that may be used. Nerve grafts with vascular pedicles have been used, but this procedure significantly prolongs surgery and requires additional operative experience and skill. Other limitations to autologous donation include inherent restrictions on the length and diameter of donor nerves. Most donor nerves are ˜2 to 3 mm in diameter, limiting the diameter of the nerves to which they may be coapted. Furthermore, although the sural nerve can provide as much as 30 to 35 cm of donor material, complex lesions such as brachial plexus injuries may require several lengths of nerve graft, exceeding the supply of nerve that may be harvested with acceptable donor site morbidity. To address the shortcomings of autologous nerve grafts, various substances, both biological and synthetic, have been used as conduits in a process called tubulization. To understand the potential benefits and challenges of conduits in nerve regeneration it is necessary to have a basic understanding of the mechanisms of nerve growth and guidance. At the beginning of the 20th century, these mechanisms were poorly understood. In the 1920s, Ramon y Cajal suggested that a long-range, diffusible chemical signal might mediate axonal guidance. In contrast, ˜20 years later, Weiss described a series of experiments using Y-shaped arteries as nerve conduits that supported a local, contact-mediated guidance mechanism. The current view of axonal guidance as a complex process simultaneously influenced by attractive and repulsive neurotropic cues operating through both contact-mediated and long-range mechanisms only became widely accepted in the 1980s. The ideal bridge between two injured nerve ends would provide an environment in which both long-range and contact-mediated guidance cues can operate, while excluding or mitigating the risks of deleterious effects such as fibrosis, scar formation, aberrant regeneration, or neuroma formation. A suitable material for guidance channels should be readily formed into a conduit of a desired diameter, flexible while able to maintain structural integrity in vivo, easily implanted, and easily sterilized. Engineered guides are enhanced by the ability to vary their physical characteristics, such as permeability, electrical conductance, and surface texture. The physical properties of the conduit can have a profound effect on nerve regeneration. Studies on both permeability and channel volume have shown that there is an ideal degree of permeability and an ideal channel volume, and extremes of either adversely affect nerve regeneration. Engineered guides also provide the opportunity to incorporate both neurotropic and neurotrophic factors to optimize axonal growth and guidance. Whereas neurotropic factors provide guidance and direction for regenerating axons, neurotrophic factors ensure survival and growth of the axons. Insoluble extracellular matrix proteins, such as laminin, fibrin, and collagen, have been shown to promote axonal growth. These proteins have been incorporated into the lumen of nerve guides with good results. The incorporation of either laminin or collagen into guidance tubes has been shown to improve regeneration relative to saline-filled controls in a concentration-dependent fashion. Additionally, soluble factors supporting nerve growth such as nerve growth factor, basic fibroblast growth factor, and transforming growth factor ßcan be incorporated either directly into the conduit or indirectly, via the implantation of support cells that secrete these factors. A major benefit of engineered nerve conduits is the obviation of the need for a second incision. Guidance channels can also provide a barrier to the infiltration of scar tissue, which inhibits axonal regeneration and may cause nerve compression. Typically, fewer sutures are needed in a standard tubulization repair, resulting in less surgical trauma to the affected nerve as well as less exposure to foreign material that may induce an inflammatory reaction. Nerve regeneration through autografts or via end-to-end repair inevitably results in imperfect alignment of proximal and distal fascicles, which may ultimately lead to aberrant regeneration and poor recovery of function. Nerve conduits can allow for the inclusion and accumulation of both neurotrophic and neurotropic factors, which can theoretically increase both the speed and the accuracy of peripheral nerve regeneration. Such an effect has been intimated by studies on injuries with no loss of nerve substance showing better recovery of sensory function with repair via tubulization rather than primary end-to-end coaptation. Allowing regeneration with neurotropic factors concentrated in the conduit may lead to a better alignment than is possible using standard microsurgical repair. Still, one possible drawback is that these conduits may alter the gradient of neurotropic factors, which, in addition to the presence of these factors, may be necessary for precise reinnervation of distal targets. Gluck documented the first known attempt to bridge a nerve gap with a tube in 1880 using a piece of hollow bone—the first description of the use of an autologous substance other than nerve as a conduit. In 1909, Wrede described the reconstruction of a median nerve with a vein graft. Although some authors continue to advocate the use of vein grafts filled with substances such as muscle or sliced neural tissue, simple vein grafts are rarely used in clinical practice. To date, studies on simple vein grafts have demonstrated mixed results. Several recent studies have shown some return of function in gaps up to 45 mm using vein grafts, whereas other studies have shown that vein grafts are not successful guides in delayed repair when compared with direct primary repair and use of nerve autografts. These poor results could be due, in part, to the thin walls of veins that may allow for vein collapse and surrounding scar tissue to compress the regenerating nerve. Furthermore, use of a vein graft does not obviate the need for a second surgical site—one of the principal shortcomings of autologous nerve grafts. Denatured muscle alone has been used as a nerve graft with the rationale that it contains several factors known to encourage nerve growth, such as type IV collagen and laminin. Nevertheless, without a suitable conduit there is a substantial risk of nerve fibers growing out of the muscle tissue leading to neuroma formation. Other groups have used inverted veins filled with muscle tissue with good functional results. However, this approach seems to exacerbate, rather than solve, the shortcomings associated with donor site morbidity. Ideally, any new conduit ought to both improve function and decrease morbidity relative to the autograft standard. To date, no biological graft has fulfilled both of these conditions satisfactorily. Synthetic conduits, on the other hand, do appear to have advantages when compared with autografts. Both biodurable and bioabsorbable conduits are discussed in the next sections. Initial experience with biodurable synthetic conduits was hopeful. Promising early results, however, were tempered by a relatively high rate of late complications. Merle et al first reported the successful use of silicone conduits in humans in 1989, demonstrating good nerve regeneration in three patients. Similarly, in 2004, Lundborg et al demonstrated in a clinical series that silicone tubulization resulted in equivalent regeneration compared with autologous nerve grafting at 5 years, with significantly less cold intolerance in gaps up to 5 mm. However, eight of 17 implanted tubes required a second procedure to remove the tube after local irritation developed. In another study by Braga-Silva (1999), seven of 26 silicone tubes necessitated removal for local irritation or nerve compression. Polytetrafluoroethylene (PTFE), commonly known as Gore-Tex (W. L. Gore and Associates, Inc., Flagstaff, AZ), has also been used as a nerve conduit with mixed results. In 1998, Stanec and Stanec reported 78.6% recovery of nerve and motor function in gaps up to 40 mm in ulnar and median nerves, leading the authors to conclude that PTFE was superior to other synthetic tubes available at the time. Despite these encouraging results, only two of seven lingual and inferior alveolar nerve repairs in another study by Pogrel et al demonstrated recovery of function, suggesting that PTFE is not effective for gaps greater than 3 mm. In a 2001 study by Pitta et al involving patients with painful dysesthesias following inferior alveolar nerve (IAN) or lingual nerve (LN) injury, four of six patients had no appreciable decrease in pain and only three of six were able to respond to touch in the affected areas following nerve reconstruction with PTFE grafts. These results led the authors to recommend against the use of PTFE for IAN or LN defects. Although biodurable conduits have proven capable of allowing peripheral nerve regeneration, they remain in situ after nerve regeneration is complete. As such, these conduits have caused chronic foreign body reactions and excessive scar tissue formation in a substantial proportion of patients, often necessitating a second operation for removal. This problem significantly limits the clinical utility of these synthetic conduits and has led to the development of bioabsorbable conduits. Bioabsorbable synthetic conduits are currently the synthetic material of choice for most surgeons. They offer many of the advantages of biodurable conduits (i.e., a chamber to concentrate neurotropic/neurotrophic chemicals, guide nerve growth, and support against neuroma formation) without the risk of increased local irritation or delayed nerve compression. Polyglycolic acid (PGA) is a well-tolerated material that has been widely used as a suture material in the clinical setting. In vivo PGA is broken down via hydrolysis, yielding a transient decline in pH. Theoretically, use of excessive amounts of PGA could thereby result in local necrosis. Nevertheless, clinical experience with PGA tubulization has been predominantly positive. In 1990, Mackinnon and Dellon reported the first human trials of a PGA conduit showing equivalency to autologous nerve grafts at 22 months in defects up to 30 mm long. In a 2005 study of seven patients utilizing the Neurotube PGA conduit for facial nerve repair, Massimo demonstrated positive results (categorized as fairly good or excellent recovery of function) in 71% of cases with no cases of intolerance or tube removal. Poly(DL-lactide-e-caprolactone) (PLCL) is another biodegradable substance whose characteristics include a longer degradation time resulting in less acidic degradation products than PGA. A randomized, controlled study of 30 patients using a commercially available PLCL tube (Neurolac, Polyganics B.V., Groningen, the Netherlands) published in 2004 by Mariëtta demonstrated functional recovery that was at least as good as the end-to-end repair control group. Extracellular matrix proteins are one potential adjunct to conduit construction. Using a resorbable PGA tube filled with a collagen sponge, Nakamura and colleagues reported recovery of both sensory and motor electrophysiological function in canines that was equivalent to and, in some cases, superior to an autograft control group. This group used similar tubes in two patients with 20 mm and 65 mm defects in peripheral nerves and reported good functional recovery in both patients and again in patients with digital nerve gaps of 25 mm and 36 mm with full recovery of finger function. Another tube, constructed entirely of highly purified type I collagen, has become commercially available for clinical use (Neuragen, Integra Neurosciences, Plainsboro, NJ). In nonhuman primates this guide produced equivalent nerve regeneration and physiological recovery over gaps of 5 mm when compared with autografts. In further animal studies by Archibald and colleagues, functional recovery has been reported using this type of guide over gaps up to 15 mm. Yet another study by Krarup et al demonstrated that collagen nerve guides were capable of allowing regeneration across gaps of as much as 50 mm, although the time to reinnervation was significantly longer with nerve guide repairs compared with nerve graft repairs. In this same study it was shown that the timing of reinnervation was the critical element in determining ultimate functional recovery, with longer time to reinnervation associated with poorer recovery of function. In 2005, Taras reported repairing 73 peripheral nerves, including median, ulnar, radial, posterior interosseous, common digital, and proper digital nerves with the Neuragen tube. Although full results have not been published, the authors’ clinical impression was that results with Neuragen are favorable when compared with direct repair or nerve grafting Although bioabsorbable conduits have shown some success at bridging gaps less than 3 cm, longer gaps may still present a problem with collapse or resorption of the graft and scar infiltration. These larger gaps may require novel approaches, such as the incorporation of biologically active factors that encourage, direct, and enhance axonal regeneration. Regenerating axons react to a host of molecular cues in the local environment. Several attempts have been made to overcome the length restrictions inherent in other synthetic tubes by using tissue engineering to combine the advantages of neurotrophic and neurotropic substances that are known to support or enhance axonal growth with the benefits of tubulization. Combining resorbable synthetic tubes with biological factors known to encourage and hasten nerve growth is an active area of research. Because regeneration occurs at ˜1 mm per day, larger gaps can lead to distal muscle wasting and ultimately unsatisfactory results. Several neurotrophic factors, such as brain-derived neurotrophic factor, nerve-growth factor, basic fibroblast growth factors-1 and -2, neurotrophin-3, insulin-like growth factor-1, platelet-derived growth factor, ciliary neurotrophic factor, interleukin-1, and transforming growth factor ßhave demonstrated capability to promote axonal growth in vitro. Their use in vivo, however, remains limited by a short half-life. Several groups have attempted to overcome the short half-life by adding Schwann cells, a potential source for these neurotrophins, to the conduits. For example, vein grafts filled with Schwann cells allowed successful bridging of rabbit nerve defects up to 60 mm. Furthermore, in a direct comparison, Schwann cell-impregnated trimethylene-carbonate-co-epsilon-caprolactone (TMC/CL) tubes, but not empty TMC/CL tubes, allowed regeneration across a 2 cm gap in the rat median nerve. Despite their potential, at this time, implantation of Schwann cells into conduits is limited in clinical practice due to difficulty obtaining a viable, nonimmunogenic, and cost-effective source. The need to achieve a tension-free repair in peripheral nerve surgery has led to the accepted standard of nerve grafting. However, the associated morbidities and suboptimal clinical results provide a compelling reason to search for viable alternatives. Resorbable conduits avoid both the problems inherent in harvesting a nerve for autograft and the potential nerve compression and irritation found with nonresorbable synthetic grafts. Allowing axons to grow through conduits, and thus to be subject to the neurotrophic guidance cues that collect there, may ultimately allow more precise alignment of fascicles and thus better functional recovery than would be possible with primary nerve repair or nerve autograft. These conduits appear to offer significant advantages, including improved regeneration when compared with traditional repair, and are currently available for use clinically. Initial reports of incorporating neurotropic and neurotrophic factors into conduits have been encouraging and will likely play a prominent role in the future of peripheral nerve repair. Evans GR. Challenges to nerve regeneration. Semin Surg Oncol 2000;19:312–318 Taras JS, Nanavati V, Steelman P. Nerve conduits. J Hand Ther 2005;18:191–197
Case Presentation
Diagnosis
Historical Perspective
Management Options
Limitations of Autologous Nerve Grafts
Benefits of Tubulization
Biological Conduits
Biodurable Synthetic Conduits
Bioabsorbable Synthetic Conduits
Discussion
Future Directions
Conclusions
Suggested Readings
Study References
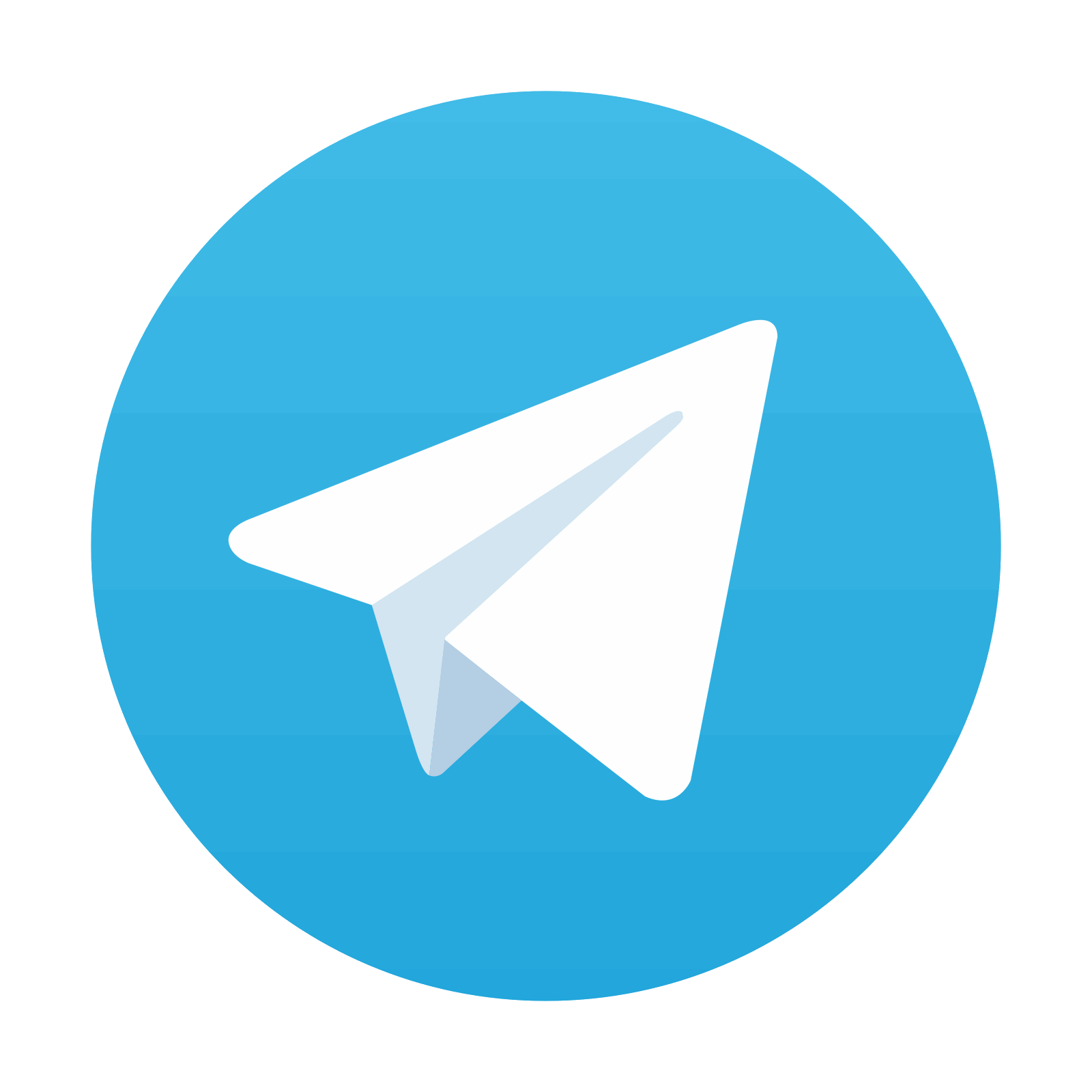
Stay updated, free articles. Join our Telegram channel

Full access? Get Clinical Tree
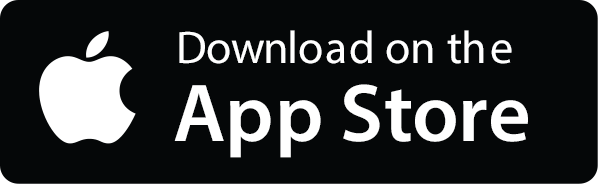
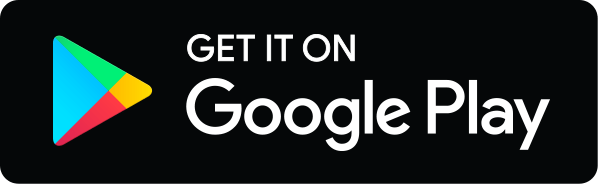