Neuroanatomical and Neurophysiologic Basis of EEG
Thoru Yamada
Elizabeth Meng
To understand the electroencephalogram (EEG) and its related field of clinical neurophysiology, it is important to know basic neuroanatomy and neurophysiology. In this chapter, neuroanatomical and neurophysiologic aspects of the nervous system are presented.
Basic Anatomical Structures of the Nervous System
The nervous system is divided into two basic parts, the central nervous system (CNS) and the peripheral nervous system (PNS). The CNS consists of the brain (cerebrum), brainstem, cerebellum, and spinal cord. The brainstem is divided into three parts, midbrain, pons, and medulla oblongata. The spinal cord is divided into cervical, thoracic, lumbar, and sacral cord (Fig. 5-1).
BRAIN (CEREBRUM)
The brain lies in the cranial cavity and is protected by the scalp and skull. Both the brain and the spinal cord are covered by three membranes: dura mater, arachnoid mater, and pia mater (Fig. 5-2). The dura mater is a thick membrane that lies just beneath the skull (cf: subdural hematoma is the collection of blood underneath the dura, and epidural hematoma is the collection of blood outside the dura, i.e., between the dura and the skull). The pia mater directly covers the entire brain surface including the cortical convolutions. Between the dura mater and pia mater, there is the arachnoid mater (cf: subarachnoid hemorrhage is bleeding underneath the arachnoid) and the cerebrospinal fluid (CSF), which is a clear and colorless fluid filling the space between the arachnoid and pia mater (the subarachnoid space).
The CNS is composed of gray matter and white matter. The gray matter, which appears grayish in color, consists of billions of nerve cells called neurons. The white matter consists of bundles of nerve fibers arising from neurons. The cerebral cortex that covers the brain surface, thalamus, and basal ganglia (such as the caudate nucleus, putamen, and globus pallidus occupying the deeper portion of the brain) is the gray matter (Fig. 5-3). The white matter of the brain is located underneath the cerebral cortex and outside the thalamus and basal ganglia.
The cerebrum is the largest part of the brain. It consists of two cerebral hemispheres, which are connected by a mass of white matter called the corpus callosum (Fig. 5-3). The cerebral cortex has multiple folds separating multiple gyri (gyrus in the singular term). The major folds are called sulci (sulcus in the singular term) or fissures. Several large fissures or sulci separate the lateral surface of the cortex into four major lobes: frontal lobe, parietal lobe, temporal lobe, and occipital lobe (Fig. 5-4). The frontal and parietal lobes are separated by the central sulcus or Rolandic fissure. The temporal lobe is separated from the frontal and parietal lobes by the lateral sulcus or sylvian fissure. Parietal and occipital lobes are separated by the parieto-occipital fissure.
![]() FIGURE 5-2 | Protective coverings of the brain. (Modified from Snell RS. Clinical Neurophysiology for Medical Students. 5th Ed. Baltimore, MD: Lippincott Williams & Wilkins, 2001, with permission.) |
Each lobe has specific, elaborate, and extremely complicated brain functions. Details of those functions are beyond the scope of this chapter. Very basic and primary functions are described here. The precentral gyrus in the frontal lobe, situated just anterior to the central sulcus, controls motor functions (primary motor cortex). The superior-medial part of the precentral gyrus represents motor function for the lower extremities, and more lateral and inferior portions of the gyrus represent motor functions for the trunk and upper extremities. The lowest portion of the precentral gyrus, that is, closest portion to the temporal lobe, controls face and tongue movements (Fig. 5-5). Since movements of the face, tongue, mouth, and fingers require much more delicate and elaborate motions than the trunk or lower extremity, these occupy much larger areas of cortex than that for the trunk or lower extremity. Damage, such as a stroke or brain tumor, or excitation, such as a seizure, in the motor cortex area would result in motor paralysis or muscle movement/twitches, respectively, in the body part corresponding to the damaged or excited area of motor cortex (see motor evoked potential section in Volume II for further detail; see also Video 14-8).
The postcentral gyrus in the parietal lobe is situated just posterior to the central sulcus (Rolandic fissure) and receives sensory input (primary sensory cortex) from the entire body (Fig. 5-4). The area corresponding to each body part is arranged in the same way as that for the motor cortex, that is, an upside-down representation with the lower extremities at the top of the gyrus and the face at the bottom of the gyrus (Fig. 5-5). Damage or excitation of an area of sensory cortex would result in sensory loss or sensory hallucination, such as numbness or tingling (see somatosensory evoked potential section of Volume II for further detail). Primary visual cortex is situated in the tip and mesial aspect of the occipital cortex (Fig. 5-4). Damage causes a visual field defect, and excitation causes visual hallucinations (see visual evoked potentials in Volume II for further detail; see Video 10-5A). In the superior gyrus of the temporal lobe, there is the primary auditory cortex, which receives auditory information. Due to extensive bilateral representation (hearing input from one ear reaches the temporal lobe in both hemispheres), damage to the auditory cortex of one side does not cause deafness in either ear, unlike other sensory or motor functions (see brainstem auditory evoked potential section in Volume II for further detail).
Most brain functions are in a mirror-imaged arrangement between the left and right hemispheres; left hemisphere receives information from or controls the right side of the body and vice versa. Some functions, especially language, have dominance in only one hemisphere. In most individuals and especially in right-handed people, the language or speech center resides in the left hemisphere, often referred to as the dominant hemisphere. Speech may be divided into separate motor and sensory functions. In the motor function, elaborate and coordinated movements of the mouth, tongue, larynx, soft palate, and respiratory muscles are required to articulate words and to speak. The motor cortex of speech is called Broca’s area and is strategically located in the inferior part of the frontal gyrus, just anterior to the primary motor cortex controlling muscles for speech, that is, mouth, lips, tongue, etc. (Figs. 5-4 and 5-5). When Broca’s area is damaged, a person can understand the spoken language but is unable to articulate words or sentences. This is called motor aphasia. In the sensory function of speech, information received through the ears reaches the primary auditory cortex located in the superior gyrus of the temporal lobe. This information needs to be interpreted to understand what is spoken. Just posterior to the primary auditory cortex, there is an area for interpretation of spoken words called Wernicke’s area (Fig. 5-4). When the Wernicke’s area is damaged, a person can speak but is unable to understand spoken language. This is called sensory or receptive aphasia.
Other elaborate cortical functional areas are also conveniently located near the primary sensory areas; just posterior to the primary sensory cortex in the postcentral gyrus, there is an area which interprets the information received in the primary sensory cortex. If this area is damaged, a person can feel an object with the hand but is unable to interpret or identify that object. This is called sensory agnosia. Similarly, there is an interpretation center for visual information just anterior to the visual cortex in the occipital lobe. Damaging this area causes visual agnosia. This is when an object can be seen but not recognized.
Other cortical dysfunctions include (i) alexia (unable to read) and agraphia (unable to write) due to a lesion at the angular gyrus in the posterior parietal lobe, (ii) astereognosis (unable to appreciate texture, size, and form by touching objects) due to a lesion at superior parietal lobe, (iii) apraxia (unable to perform purposeful and learned act such as driving a car, playing a piano, etc.) due to a lesion of various association cortex, especially in the dominant hemisphere, and (iv) amnesia (loss of memory) due to a lesion in the hippocampus.
BRAINSTEM
The brainstem lies between the brain and spinal cord and consists of the midbrain, pons, and medulla oblongata (Figs. 5-1 and 5-6). The brainstem has three major functions: (i) it functions as a conduit gathering and transmitting multiple ascending and descending tracts between the spinal cord and brain; (ii) it controls elementary life-sustaining functions such as respiration, the cardiovascular system, and level of consciousness; and (iii) it contains multiple nuclei of the cranial nerves (CN) (CN III to XII).
The details of brainstem anatomy and functions are beyond the scope of this chapter, and only basic anatomy and function will be described here.
The midbrain has four colliculi (corpora quadrigemina), which are rounded eminences that are divided into superior and inferior parts. The superior colliculi (colliculus in the singular term) are gray matter with collections of various nuclei. They relate to a part of the visual reflex system and have multiple motor and sensory traits. They connect to the lateral geniculate body (see visual evoked potential section of Volume II for further details). Inferior colliculi also have multiple nuclei and relate to the auditory pathway, having multiple sensory and motor pathways. They connect to the medial geniculate body (see brainstem auditory evoked potential section in Volume II for further details). The oculomotor nerve (CN III) and trochlear nerve (CN IV) exit from the midbrain. Both of these cranial nerves control eye movement. CN III also functions in the pupillary reflex.
The pons connects the medulla oblongata to the midbrain. The posterior portion of the pons, called the tegmentum, extends from the midbrain. The pons contains numerous nuclei and fiber tracts including the medial lemniscus, which carries the ascending sensory fibers for proprioceptive (position sense) and vibratory sensation. The pons also functions as a bridge connecting the left and right cerebellar hemispheres. The trigeminal nerve (CN V), which receives facial sensation and controls masticatory muscles, enters and exits from midpons. The medulla connects the pons superiorly and spinal cord inferiorly. On each side of the median fissure, there is an elongated eminence called the pyramid. The pyramids are composed of bundles of nerve fibers originating from the motor cortex (precentral gyrus) descending as the corticospinal tract. The majority of descending fibers cross over to the opposite side, forming the pyramidal decussation. The abducens nerve (CN VI, controls eye movements), facial nerve (CN VII, controls facial muscles), and acoustic or vestibulocochlear nerve (CN VIII, receives hearing input) emerge from the border of the pons and medulla. The remaining cranial nerves (CN IX, glossopharyngeal nerve controls oropharyngeal muscles; CN X, vagus nerve controls autonomic function; CN XI, accessory nerve controls sternocleidomastoid and trapezius muscles; and CN XII, hypoglossal nerve controls tongue movement) exit from the medulla oblongata (cf: CN I is the olfactory nerve and arises from the base of the brain. CN II is the optic nerve and passes between the base of the brain and brainstem).
CEREBELLUM
The cerebellum is located in the posterior cranial fossa and lies posterior to the fourth ventricle, pons, and the medulla oblongata. It consists of two cerebellar hemispheres joined by a narrow median vermis. The cerebellum receives afferent information concerning voluntary movement from the cerebral cortex and also from muscles, tendons, and joints. Cerebellar output is conducted to the sites that influence motor activity at the segmental spinal level. The cerebellum functions as a coordinator for precise voluntary movement, which requires a continuous balance between output from the cerebral motor cortex and feedback of proprioceptive information from the muscles. This then allows the degree of muscle contraction necessary for a precise voluntary movement to be adjusted.
Damage to one cerebellar hemisphere causes a disturbance of voluntary movement called ataxia on the same side of the body; the muscle group fails to work harmoniously, and the patient may have difficulty in picking up an object, writing, or shaving (dysmetria). The patient may have difficulty walking and may tend to fall to the same side as the lesion (ataxia). The patient may have difficulty articulating words, called dysarthria, due to a failure of coordinated movements of the tongue, mouth, and larynx (cf: this is different from motor aphasia resulting from damage to the cerebral cortex or Broca’s area). Damage in the midline of the cerebellum (vermis) causes a disturbance in the midline body parts, such as the head and trunk; the patient may have difficulty in holding the trunk straight (truncal ataxia).
Despite the important and fundamental functions of the cerebellum for daily living activities, there is no specific neurophysiologic test that directly measures cerebellar function.
SPINAL CORD
The spinal cord begins at the foramen magnum in the skull base where it connects to the medulla oblongata rostrally.
It is protected by multiple vertebrae that allow for flexible movement. The spinal cord terminates inferiorly at the level of the lower border of the first lumbar vertebra in an adult. The spinal cord in children ends at the upper border of the third lumbar vertebra. Like the brain, the spinal cord is also surrounded by pia mater, arachnoid mater, and dura mater, and there is CSF in the subarachnoid space.
It is protected by multiple vertebrae that allow for flexible movement. The spinal cord terminates inferiorly at the level of the lower border of the first lumbar vertebra in an adult. The spinal cord in children ends at the upper border of the third lumbar vertebra. Like the brain, the spinal cord is also surrounded by pia mater, arachnoid mater, and dura mater, and there is CSF in the subarachnoid space.
The spinal cord is enlarged (see Fig. 5-1) at the cervical level (cervical enlargement) to allow abundant nerves to exit and enter the brachial plexus, connecting peripheral nerves of the upper limbs (Fig. 5-7). Similarly, at the lumbar level, there is a lumbar enlargement (see Fig. 5-1) allowing abundant nerves to exit and enter the sacral plexus, connecting the nerves of the lower limb. The spinal cord tapers into the conus medullaris and progresses to the filum terminale, which ends at the coccyx (see Fig. 5-1). There are abundant peripheral nerves descending from the first lumbar vertebrae to the coccyx, which are called the cauda equina (because it looks like a horse’s tail).
Along the entire length of the spinal cord, 31 pairs of spinal nerves (8 cervical, 12 thoracic, 5 lumbar, 5 sacral, and 1 coccygeal) are attached. The spinal nerves consist of two nerve roots, one posterior and another anterior. The posterior root carries sensory information and enters the posterior part of spinal cord. Each posterior root has a posterior (or dorsal) root ganglion containing the cell body that gives rise to the peripheral and central portions of the nerve fiber. The anterior root carries motor information and exits from the anterior part of the spinal cord. The peripheral nerves may contain sensory and motor fibers (mixed nerve) or either sensory or motor fibers alone. The median and ulnar nerves are mixed nerves at the wrist but contain sensory fibers only in the fingers. The posterior tibial nerve is a mixed nerve, and the sural nerve is a sensory nerve (see somatosensory evoked potential in Volume II for further details of peripheral nerve innervations).
THE AFFERENT AND EFFERENT SYSTEMS
Both the CNS and the PNS comprise afferent and efferent pathways. The typical example of an afferent pathway is the sensory system in which sensory signals originate from the peripheral receptors (such as muscle spindles in the muscle tissue, joint receptors from the joint, etc.) and travel to the CNS via the PNS.
The peripheral nerve fibers from pain, temperature, and touch receptors traveling through small myelinated or unmyelinated fibers (Fig. 5-8A) enter the posterior portion of the spinal cord. The fibers then cross over to the opposite side of the spinal cord after synaptic connection at the substantia gelatinosa and ascend as the spinothalamic (lateral or anterior) tract (Fig. 5-8A). The fibers then ascend as spinal lemniscus at the brainstem and proceed to the ventroposterolateral (VPL) nucleus of the thalamus, finally reaching the postcentral gyrus.
The peripheral nerve fibers from muscle spindles and joint receptors carrying proprioceptive sense and discriminative touch sense consist of large myelinated fibers. They enter the posterior part of the spinal cord, the same way as the fibers of pain/temperature senses. But unlike pain/temperature pathways crossing to the opposite side of spinal cord, these ascend to the brainstem via the dorsal column on the same side as they entered the fasciculus cuneatus (from upper extremity) and the fasciculus gracilis (from lower extremity) (Fig. 5-8B). At the brainstem, synaptic connections occur in the cuneate nucleus (for nerve fibers from the upper extremity) and in the gracile nucleus (for nerve fibers from the lower extremity). After synaptic connections in the cuneate or gracile nucleus, the fibers then cross to the opposite side and ascend the brainstem as the medial lemniscus to the VPL nucleus of the thalamus. After the thalamus, the sensory fibers reach the sensory cortex (postcentral gyrus) via the internal capsule.
A typical efferent pathway is the motor system in which signals originating from the motor cortex (precentral gyrus) travel through the internal capsule down to the brainstem where the majority of fibers cross to the opposite side (pyramidal decussation) at the level of the medulla (Fig. 5-9; see also Fig. 5-6A). The fibers then descend to the anterior horn cells of the spinal cord and exit from the anterior portion of the spinal cord. They finally reach their designated muscles via the PNS.
Vascular System of the Brain and Spinal Cord
ARTERIES OF THE BRAIN
The brain is supplied by the two internal carotid arteries and two vertebral arteries.
Internal Carotid Artery
The internal carotid artery starts at the bifurcation of the common carotid artery, where the external carotid artery also gives rise. The right common carotid artery arises from the brachiocephalic artery branching from the aortic arch, which directly connects to the heart (Fig. 5-10). The left common carotid artery directly arises from aortic arch. The internal carotid artery divides into the anterior and middle cerebral arteries after entering the cranium (Figs. 5-10 and 5-11). The anterior cerebral artery runs forward and medially and supplies a large portion of medial surface of the brain including the frontal and parietal lobes. Left and right anterior cerebral arteries are connected via the anterior communicating artery (as a part of Circle of Willis). The middle cerebral artery runs laterally and supplies a large portion of the lateral hemisphere including the frontal, parietal lobes, and superior and middle temporal gyri. The corpus striatum and the internal capsule are also supplied by the middle cerebral artery.
Vertebral Artery
The vertebral artery arises from the subclavian artery (a part of the aortic arch) and ascends the neck by passing through the foramina of the transverse process of the cervical vertebra. It enters the skull through the foramen magnum. The vertebral artery gives rise to the posterior inferior cerebellar artery, supplying a large portion of the cerebellum. Left and right vertebral arteries then join at the lower border of the pons and form the basilar artery. The basilar artery splits into left and right posterior cerebral arteries at the midbrain level. The posterior cerebral artery supplies the posterior portion of medial hemisphere and inferior and medial temporal gyri. The posterior cerebral artery also sends a branch to the posterior communicating artery, which connects to the internal carotid artery (Figs. 5-10 and 5-11).
Circle of Willis
The anterior communicating, anterior cerebral, internal carotid, posterior communicating, posterior cerebral, and basilar arteries form the Circle of Willis by multiple anastomoses. This allows blood to be distributed to any part of the cerebral hemisphere by either the internal carotid or the vertebral/basilar arteries should either artery fail (Fig. 5-11).
VENOUS DRAINAGE OF THE BRAIN
There are six major sinuses that collect venous drainage from various veins. They are the superior sagittal sinus, inferior sagittal sinus, straight sinus, occipital sinus, transverse sinus, and sigmoid sinus. All sinuses join together at the sigmoid sinus, which connects to the internal jugular vein, returning venous blood to the heart (Fig. 5-12).
ARTERIES OF THE SPINAL CORD
The spinal cord receives arterial supply from two (left and right) posterior spinal arteries and one anterior spinal artery. All spinal arteries arise from the left and right vertebral arteries (Fig. 5-13). At each intervertebral foramen, the posterior spinal artery sends a segmental spinal artery. The lower two thirds of the spinal cord is mainly supplied by one large, important
feeder artery called the great anterior medullary artery of Adamkiewicz, which arises from the abdominal aorta at the lower thoracic or upper lumbar vertebral levels. (See section of intraoperative monitoring in volume II for further details.)
feeder artery called the great anterior medullary artery of Adamkiewicz, which arises from the abdominal aorta at the lower thoracic or upper lumbar vertebral levels. (See section of intraoperative monitoring in volume II for further details.)
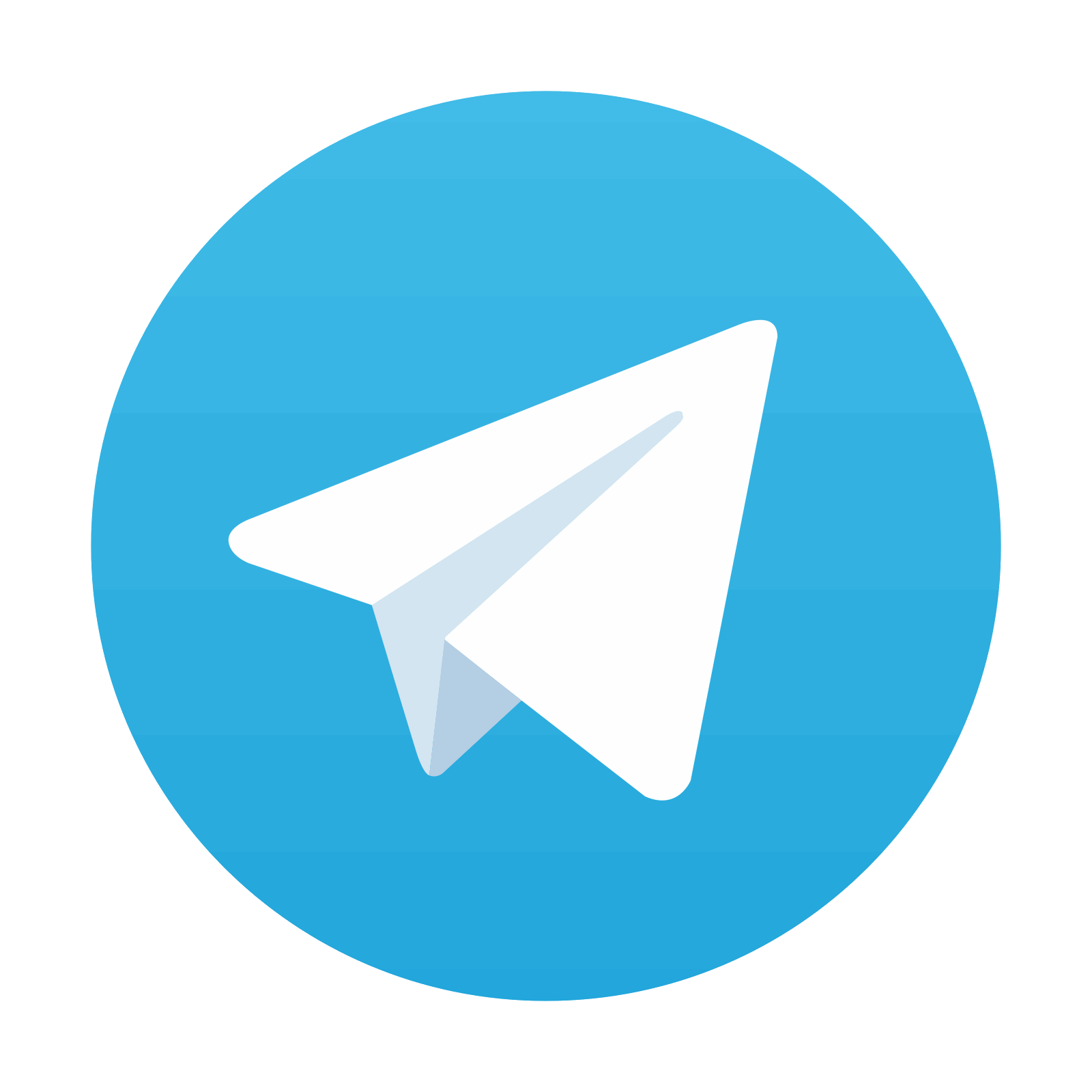
Stay updated, free articles. Join our Telegram channel

Full access? Get Clinical Tree
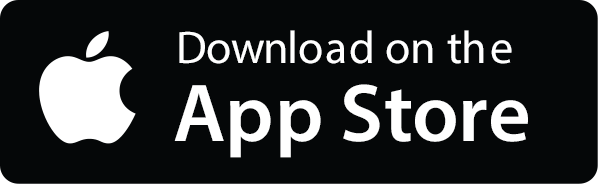
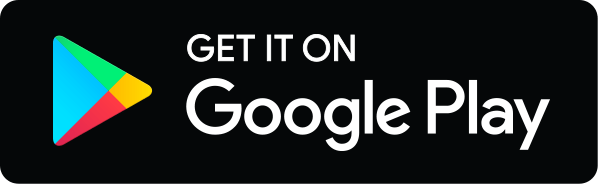