I. Neurotransmitters (NTs) and Receptors A. Miscellaneous 1. Three major categories of NTs a. Amino acids i. Glutamate ii. γ-Aminobutyric acid (GABA) iii. Aspartic acid iv. Glycine b. Peptides i. Vasopressin ii. Somatostatin iii. Neurotensin c. Monoamines i. Norepinephrine (NE) ii. Dopamine (DA) iii. Serotonin (5-hydroxytryptamine [5-HT]) iv. Acetylcholine (ACh) 2. Monoamine NTs are nearly always (with a few exceptions) inhibitory. 3. ACh is the major NT in the peripheral nervous system (the only other peripheral NT being NE). 4. Major NTs of the brain are glutamate and GABA. 5. Peptides perform specialized functions in the hypothalamus and other regions. 6. Peripheral nervous system has only two NTs: a. ACh b. NE 7. Excitatory NTs a. Glutamate b. Aspartate c. Cystic acid d. Homocystic acid 8. Inhibitory NTs a. GABA b. Glycine c. Taurine d. β-Alanine 9. Excitatory/inhibitory pairs a. Glutamate (+): GABA (–) in the brain b. Aspartate (+): glycine (–) in the ventral spinal cord B. ACh 1. Miscellaneous a. First NT discovered b. The major NT in the peripheral nervous system i. Provides direct innervation of skeletal muscles ii. Provides innervation of smooth muscles of the parasympathetic nervous system c. Major locations of ACh i. Autonomic ganglia ii. Parasympathetic postganglionic synapses iii. Neuromuscular junction (NMJ) iv. Renshaw cells of spinal cord d. Roles of ACh i. Thermal receptors ii. Chemoreceptors iii. Taste iv. Pain perception (possibly) e. Primarily (but not always) an excitatory NT f. Main effect of ACh on pyramidal cells is via muscarinic receptor-mediated depletion of K+ currents, which results in hyperexcitability g. Most dietary choline comes from phosphatidyl choline found in the membranes of plants and animals. h. Phosphatidyl choline is converted to choline, which is then transported across the blood-brain barrier. i. Acetylcoenzyme A and choline are independently synthesized in the neuronal cell body and independently transported along the axon to the synapse in which they are conjugated into Ach. 2. Synthesis: Rate limiting: supply of choline 3. Release a. Voltage-gated calcium channel is open as the action potential (AP) reaches the terminal button of the presynaptic neuron, producing an influx of calcium ions that allows exocytosis of presynaptic vesicles containing ACh into the synaptic cleft. b. The activation of postsynaptic ACh receptors results in an influx of Na+ into the cell and an efflux of K+, which depolarizes the postsynaptic neuron, propagating a new AP. 4. Receptors a. Muscarinic receptors i. Subtypes (A) M1, 3, 5: activate phosphatidyl inositide hydroxylase (B) M2, 4: inhibit adenyl cyclase ii. Agonists (A) Bethanecol (B) Carbachol (C) Pilocarpine (D) Methacholine (E) Muscarine (from Amanita mushroom) iii. Antagonists (A) Atropine (B) Scopolamine (C) Trihexyphenidyl b. Nicotinic receptors i. Antagonists (nondepolarizing) (A) Tubocurarine (B) Atracurium (C) a-Neurotoxin of sea snakes (D) Procainamide (E) Aminoglycoside antibiotics ii. Antagonists (depolarizing) (A) Succinylcholine iii. Receptor inactivation (A) Myasthenia gravis iv. Receptor deficiency (A) Congenital myasthenia gravis v. ACh release augmentation (A) Black widow spider latrotoxin vi. ACh release blockade (A) Botulism (B) Lambert-Eaton syndrome (C) Tick paralysis (D) β-Neurotoxin of sea snakes c. Specific locations of muscarinic and nicotinic receptors i. Both nicotinic and muscarinic (A) Central nervous system (CNS) (muscarinic > nicotinic receptor concentrations) (B) All sympathetic and parasympathetic preganglionic synapses ii. Muscarinic only (A) All postganglionic parasympathetic terminals (B) Postganglionic sympathetic sweat glands iii. Nicotinic only (A) NMJ (B) Adrenal medulla iv. In brain: muscarinic > nicotinic 5. Vesicle transport a. SNARE proteins: i. Mediate docking of synaptic vesicles with the presynaptic membrane ii. Targets of the bacterial neurotoxins responsible for botulism and tetanus b. SNAP-25: i. Synaptosomal-associated protein 25 (SNAP-25) accounts for the membrane fusion (bringing the synaptic vesicle and plasma membranes together). ii. Botulinum toxins A, C, and E cleave SNAP-25, leading to muscle paralysis as intended in clinically induced botulism. 6. Inactivation a. Metabolism i. Within synaptic cleft by acetylcholinesterase ii. Acetylcholinesterase found at nerve endings is anchored to the plasma membrane through a glycolipid. 7. Cholinergic agonists AGONISTS SOURCE MODE OF ACTION Nicotine Alkaloid prevalent in the tobacco plant Activates nicotinic class of ACh receptors, locks the channel open Muscarine Alkaloid produced by Amanita muscaria mushrooms Activates muscarinic class of ACh receptors a-Latrotoxin Protein produced by the black widow spider Induces massive ACh release, possibly by acting as a Ca2+ ionophore 8. Cholinergic antagonists ANTAGONISTS SOURCE MODE OF ACTION Atropine/scopolamine Alkaloid produced by the deadly nightshade, Atropa belladonna Blocks ACh actions only at muscarinic receptors Botulinum toxin Eight proteins produced by Clostridium botulinum Inhibits the release of ACh β-Bungarotoxin Protein produced by Bungarus genus of snakes Prevents ACh receptor channel opening d-Tubocurarine Active ingredient of curare Prevents ACh receptor channel opening at motor end plate 9. Specific agonist/antagonist action a. Presynaptic NMJ release blockade i. Botulinum toxin: block presynaptic vesicle mobility (see Sections 5.a and b) ii. Lambert-Eaton syndrome: block presynaptic Ca2+ channels iii. Sea snake venom b. Postsynaptic NMJ receptor blockade i. Myasthenia gravis: ACh receptor antibody ii. Succinylcholine: depolarizing blockade iii. Curare: nondepolarizing blockade iv. α-Bungarotoxin: irreversible ACh receptor blockade 10. Anticholinesterases a. Reversible i. Neostigmine ii. Pyridostigmine iii. Physostigmine iv. Donepezil, galantamine, rivastigmine, tacrine b. Irreversible i. With irreversible anticholinesterases, receptors can be regenerated with pralidoxime (peripherally) and atropine (centrally). ii. Agents (A) Organophosphates (B) Carbamates (C) Nerve gas 11. Conditions/medications that increase ACh concentration a. Acetylcholinesterase inhibitors i. Pyridostigmine ii. Physostigmine iii. Edrophonium iv. Donepezil, galantamine, rivastigmine, tacrine v. Organophosphates vi. Black widow venom vii. β-Bungarotoxin b. Enhances of neurotransmission i. Pyridostigmine ii. 3,4-diaminopyridine C. Catecholamines 1. Miscellaneous a. Principal catecholamines i. NE ii. Epinephrine iii. DA b. Synthesis c. Tyrosine (TYR) transported to catecholamine-secreting neurons in which it is converted into DA, NE, and epinephrine d. Direct innervation of the sympathetic nervous system (except for sweat glands) due to NE e. β-Noradrenergic receptors inhibit feeding, whereas α receptors stimulate feeding. 2. DA a. Miscellaneous i. 3 to 4 times more dopaminergic cells in the CNS than adrenergic cells ii. DA made in the substantia nigra: neurons in the pars compacta of the substantia nigra account for 80% of DA in the brain; neuromelanin is a DA polymer that makes the substantia nigra appear dark. iii. Highest concentration of DA: striatum (caudate and putamen)—although made in the substantia nigra, is transported to the striatum from the substantia nigra in vesicles iv. Two primary DA-receptor types found in striatum: D1 (stimulatory) and D2 (inhibitory) v. D2 receptors are found predominantly on dopaminergic neurons functioning primarily as autoreceptors to inhibit DA synthesis and release. vi. Four main dopaminergic tracts (A) The nigrostriatal tract accounts for most of the brain’s DA. (B) The tuberoinfundibular tract controls release of prolactin via D2 receptors. (C) The mesolimbic tract (D) The mesocortical tract vii. Parkinson’s disease develops when striatal DA is depleted by >80% (<20% of original concentration remaining) b. Synthesis i. Rate-limiting step: TYR hydroxylase conversion to L-dopa ii. DA is feedback inhibitor iii. TYR (A) Not an essential amino acid because it can be synthesized in the liver from phenylalanine (B) Cannot be synthesized in the brain (C) Must enter the brain by the large neutral amino acid transporter, which transports TYR, phenylalanine, tryptophan, methionine, and the branch-chained amino acids iv. L-TYR converted to L-dopa within the brain v. DA is synthesized in the cytoplasm. c. Receptors i. The receptor that determines whether the transmitter is excitatory or inhibitory ii. D1 receptor (subtypes D1 and D5) (A) Postsynaptic receptors (1) Excitatory (2) Stimulates cyclic adenosine monophosphate (cAMP) (B) D1 receptor: ↑ adenylate cyclase (C) D1-receptor activation is required for full postsynaptic expression of D2 effects iii. D2 receptor (subtypes D2, D3, and D4) (A) Presynaptic receptor: inhibitory (high affinity) (B) Postsynaptic receptor (1) Inhibitory (low affinity) (2) Genetic polymorphisms exist for the D4 receptor that may provide basis for genetic-based schizophrenia iv. Tardive dyskinesia may be due to supersensitivity of DA receptors that have been chronically clocked (i.e., psychotropic agents) v. Tuberoinfundibular DA system: regulated by prolactin d. Inactivation i. Reuptake (A) Presynaptic intraneuronal monoamine oxidase (MAO) converts DA → 3,4-dihydroxyphenylacetic acid (DOPAC) (B) Extraneuronal MAO and catechol-O-methyltransferase convert DA → homovanillic acid; CNS DA metabolite: homovanillic acid 3. NE a. Miscellaneous i. Neuropeptide Y: co-localized with NE in sympathetic nerve terminals, innervating blood vessels ii. Most concentrated in CNS within locus ceruleus of the pons followed by lateral tegmental area iii. Electrical stimulation of the locus ceruleus produces arousal. iv. Benzodiazepines decrease firing in the locus ceruleus, which reduces release of NE to rest of brain, causing relaxation and sedation. v. Antidepressant effect of MAO inhibitors (MAOIs) is more related to NE than to DA. b. Synthesis i. Rate-limiting step (A) TYR hydroxylase (B) NE is feedback inhibitor. ii. NE is synthesized in the storage vesicles. iii. TYR hydroxylase is inhibited by a-methyl-p-TYR. c. Release and vesicle storage i. Calcium influx with depolarization ii. Amphetamines increase release. iii. Inhibition of transport (A) Reserpine (B) Tetrabenazine iv. NE is displaced from vesicles by: (A) Amphetamine (B) Ephedrine d. Receptors i. α-1 (A) Postsynaptic (B) Most sensitive to epinephrine (C) Blocked by prazosin and clonidine ii. α-2 (A) Presynaptic (B) Inhibits adenyl cyclase via G-protein effects (C) Inhibited by yohimbine and clonidine e. Inactivation i. Metabolism (A) Catechol-O-methyltransferase in synaptic cleft (B) Reuptake (1) Primary mode of NE termination (2) Reuptake inhibited by: (a) Cocaine (b) Tricyclic antidepressants (TCAs) (desipramine) (c) Tetracyclic antidepressant (maprotiline) (d) Selective serotonin reuptake inhibitors (SSRIs) f. Other medication effects i. Lithium (A) Decreases NE release (B) Increases NE reuptake 4. Epinephrine a. Miscellaneous i. Epinephrine is found with NE in: (A) Lateral tegmental system (B) Dorsal medulla (C) Dorsal motor nucleus (D) Locus ceruleus b. Synthesis: epinephrine synthesis occurs only in adrenal medulla via phenylethanolamine N-methyltransferase. 5. Medications a. Catecholamine agonists/antagonists i. Neuroleptics (A) Based on D2- and D4-receptor antagonism in the mesolimbic and mesocortical pathways (B) Antagonism of nigrostriatal pathways produces extrapyramidal side effects. (C) Antagonism in the chemoreceptor trigger zone produces antiemetic effect. (D) Older neuroleptics mainly block D2 receptor but can block multiple DA receptors. (E) D2 affinity correlates to efficacy. (F) Clozapine (1) Neuroleptic that is more selective for the D1 and D4 receptors; also binds to: 5-HT2 receptor, a1-adrenergic receptor, muscarinic receptor, histamine (histamine1) receptor (2) DA neurons in ventral tegmentum develop depolarization inactivation, but neurons in the substantia nigra do not have this effect (i.e., minimal parkinsonism). ii. Amphetamines (A) Increase release of DA and NE centrally and peripherally (B) Decrease reuptake of DA iii. MAOIs: decrease metabolism of DA iv. Cocaine: blocks reuptake of DA and NE v. TCAs: block reuptake of DA vi. Reserpine and tetrabenazine: prevent vesicle storage of DA, epinephrine, and 5-HT, both centrally and peripherally vii. Selegiline and rasagiline: MAOB inhibitor, increasing DA stores D. 5-HT 1. Miscellaneous a. An indolamine b. Most prominent effects on cardiovascular system, with additional effects in the respiratory system and the intestines c. Vasoconstriction is a classic response to the administration of 5-HT. d. Only 1% to 2% of 5-HT in the body is in the brain; widely distributed in platelets, mast cells, etc.; greatest concentration of 5-HT (90%) is found in the enterochromaffin cells of the gastrointestinal tract. e. High concentration in CNS found in: i. Raphe nuclei that project to the limbic system ii. Pons/upper brainstem iii. Area postrema iv. Caudal locus ceruleus v. Interpeduncular nucleus vi. Facial (cranial nerve VII) nucleus f. Raphe nuclei i. 5-HT neurons are located in the CNS. ii. Projects caudally mainly to the medulla and spinal cord for the regulation of pain iii. Projects rostrally to the limbic structures and the cerebral cortex iv. Stimulation produces similar effects as lysergic acid diethylamine (LSD) g. 5-HT and NE regulate arousal. h. Low 5-HT associated with anxiety and impulsive behavior i. 5-HT syndrome ii. SSRI + MAOI iii. Clinical: restlessness, tremor, myoclonus, hyperreflexia, diarrhea, diaphoresis, confusion, and possible death iv. Must wait 2 to 3 weeks after stopping MAOI before initiating SSRI v. Must wait 5 weeks after stopping SSRI before initiating MAOI 2. Synthesis a. Rate-limiting step: tryptophan hydroxylase b. 5-HT in the brain is independently synthesized from tryptophan transported across the blood-brain barrier. 3. Receptors a. Most receptors are coupled to G proteins that affect the activities of adenylate cyclase or phospholipase C. b. 5-HT1 receptor function i. Thermoregulation ii. Sexual behavior iii. Hypotension c. 5-HT2 receptor function i. Vascular contraction ii. Platelet aggregation d. 5-HT3 receptor function: ion channels 4. Inactivation a. Metabolism b. Reuptake i. Primary mode of inactivation ii. Mechanism similar to NE c. 5-HT also converted to melatonin (only in pineal gland) 5. Agonists/antagonists a. Storage i. Disrupted by reserpine and tetrabenazine (A) Reserpine (an extract of the Rauwolfia plant) prevents the transport of all the monoamines and ACh into storage vesicles in the presynaptic membrane, allowing MAO metabolism to occur. b. Release i. Increased release of 5-HT (A) Amphetamine (B) Fenfluramine ii. Increased release and blocked reuptake of 5-HT (A) Clomipramine (B) Amitriptyline c. Reuptake i. Blocked by: (A) TCAs: inhibit NE and 5-HT reuptake by presynaptic nerve terminals (B) SSRIs (fluoxetine, sertraline): selectively prevent the reuptake of 5-HT (C) Clomipramine: although a TCA, it is an SSRI. d. LSD i. Acts most strongly on the 5-HT2 receptors (and some effect on NE receptors) ii. Small doses potentiate 5-HT activity. iii. High doses inhibit 5-HT activity, leading to psychedelic action. e. 5-HT agonists i. Sumatriptan: potent 5-HT2 agonist ii. Methysergide iii. Cyproheptadine f. 5-HT antagonist: clozapine E. Glutamate 1. Miscellaneous a. Excitatory NT b. Glutamate is NT of corticostriate fibers. c. Most common NT in the brain d. High concentration in dorsal spinal cord and dentate nucleus e. Aspartic acid and glutamate have the capacity for neuronal damage via excitotoxicity 2. Receptors a. N-methyl-D-aspartate i. Only known receptor that is regulated both by a ligand (glutamate) and by voltage ii. Mainly activate Ca2+ channels iii. N-methyl-D-aspartate receptor locations (A) Cortex (B) Hippocampal neurons, particularly the CA1 region (C) Amygdala (D) Basal ganglia iv. Five binding sites alter channel opening (A) Glutamate (increase) (B) Glycine (increase) (C) Polyamine (increase): binds the hallucinogenic substance phencyclidine (D) Magnesium (decrease) (E) Zinc (decrease) v. Glycine binding is required for activation. vi. Voltage-dependent blockers (A) Phencyclidine (B) Ketamine (C) Magnesium vii. Voltage-independent blocker: zinc viii. Associated with long-term potentiation and long-term depression, which are integral for learning and memory b. AMPA i. Mainly activate sodium channel ii. Major source of excitatory postsynaptic potentials (EPSPs) iii. Receptor affinity: AMPA > glutamate > kainate iv. GluR3 receptor: implicated in Rasmussen’s encephalitis c. Kainate i. Receptor affinity: kainate > glutamate > AMP ii. No specific antagonists iii. Derived commercially from seaweed d. 1-amino-1,3-cyclopentone dicarboxylic acid (ACPD): G-coupled formation of IP3 e. L-AP4 i. G-coupled formation of AMP ii. Inhibitory autoreceptor 3. Inactivation 4. Other a. Caffeine: increases alertness and possibly produces anxiety by blocking adenosine receptors that normally inhibit glutamate release b. Mercury poisoning: damage to astrocytes prevents resorption of glutamate, resulting in excitotoxicity. c. Lamotrigine inhibits release of excitatory NTs glutamate and aspartate. F. GABA 1. Miscellaneous a. Inhibitory NT: inhibitor of presynaptic transmission in the CNS and retina b. 30% to 40% of all synapses (second only to glutamate as a major brain NT) c. Most highly concentrated in the basal ganglia (with projections to the thalamus); also concentrated in the hypothalamus, periaqueductal gray, and hippocampus 2. Synthesis: glutamate decarboxylase decreased in striatum of Huntington’s disease 3. Receptors a. Connected to a chloride ion channel, allowing chloride to enter the cell and increasing the threshold for depolarization b. GABA-A i. Fast inhibitory postsynaptic potentials (IPSPs) ii. Increase chloride conductance iii. Five binding sites (A) Benzodiazepine: increases chloride conductance of presynaptic neurons (B) Barbiturate: prolongs duration of chloride channel opening (C) Steroid site (D) Picrotoxin site (E) GABA site iv. CNS locations (A) Cerebellum: highest concentration in granule cell layer (B) Cortex (C) Hippocampus (D) Basal ganglia v. GABA-A receptor binds (A) GABA (B) Benzodiazepine (C) β-Carbolines (D) Picrotoxin-like convulsant drugs: noncompetitive antagonist (E) Bicuculline: competitive antagonist (F) Barbiturates c. GABA-B i. Slow IPSPs ii. Increased K+ conductance via K+ channels iii. Coupled to G-protein that uses adenyl cyclase as a second messenger iv. Agonist: baclofen v. Antagonist: phaclofen vi. CNS locations (A) Cerebellum (B) Cord 4. Inactivation a. Reuptake b. Enzyme metabolism 5. Agonists/antagonists a. Inhibitors of GABA transaminase i. Valproic acid ii. Vigabatrin 6. Other a. Benzodiazepines i. Increase the frequency of chloride channel opening ii. Enhance the effect of GABA on GABA-A receptors b. Caffeine: neutralizes the effects of benzodiazepines by inhibiting GABA release c. Barbiturates: prolong the duration of opening G. MAO 1. Miscellaneous a. Antidepressant effect of MAOIs is more related to NE than DA. b. MAOA i. MAOA inhibitors have proven to be better antidepressants because MAOA metabolizes NE and 5-HT; therefore, inhibition increases NE and 5-HT levels. ii. MAOA-inhibiting drugs given for depression have critically elevated blood pressure in patients eating tyramine-containing foods (e.g., cheese). c. MAOB i. Alcohol also selectively inhibits MAOB. ii. MAOB is the most common form in the striatum. iii. MAOB metabolizes the neurotoxin 1-methyl-4-phenyl-1,2,3,6-tetrahydropyridine (MPTP). iv. Selegiline, rasagiline: specific MAOB inhibitor d. Mitochondrial MAO degrades intraneuronal DA, NE, and 5-HT that is not protected within storage vesicles. e. Hypertensive crisis i. MAO in the gastrointestinal system usually prevents entrance of large amounts of ingested tyramine (or other pressor amines). ii. If MAOI is used, then ingested tyramine can be absorbed and produce sympathetic response. iii. Clinical: sudden occipital or temporoparietal headache, sweating, fever, stiff neck, photophobia (can be mistaken for meningitis) iv. Foods to avoid with MAOIs (A) Aged cheese (B) Smoked or pickled meats, fish, or poultry (C) Caviar (D) Non-fresh meat (E) Liver (F) Nondistilled alcohol (G) Broad beans (fava, Italian green, Chinese pea pods) (H) Banana peel (I) Sausage (J) Corned beef (K) Sauerkraut v. Medications/drugs to avoid with MAOIs (A) Amphetamines (B) Cocaine (C) Anorectics/dietary agents (D) Catecholamines (E) Sympathomimetic precursors (DA, levodopa) (F) Sympathomimetic (ephedrine, phenylephrine, phenylpropanolamine, pseudoephedrine) (G) Meperidine vi. Treatment of hypertensive crisis: phentolamine, 5 mg intravenously, or nifedipine, 10 mg sublingually 2. Location a. Outer surface of presynaptic mitochondria b. Postsynaptic cell membrane 3. Inhibitors a. MAOA: clorgyline b. MAOB: selegiline, pargyline, rasagiline c. Nonspecific MAOIs: phenelzine, isocarboxazid, tranylcypromine H. Glycine 1. Miscellaneous a. Inhibitory NT of cord for inhibitory interneurons (Renshaw cells), which inhibit anterior motor neurons of the spinal cord b. Glycine binds to a receptor that makes the postsynaptic membrane more permeable to Cl2 ion, which hyperpolarizes the membrane, making it less likely to depolarize (inhibitory NT). c. Opposite function of aspartate in the spinal cord d. Anoxia results in loss of inhibitory neurons and decreased glycine. 2. Synthesis 3. Inactivation: deactivated in the synapse by active transport back into the presynaptic membrane 4. Agonists/antagonists a. Antagonist i. Strychnine (A) Antagonist (B) Noncompetitively blocks glycine > GABA receptors by inhibiting opening of the chloride channel, which subsequently results in hyperexcitability ii. Tetanus toxin: blocks release of glycine and GABA b. Agonist: glycine > β-alanine > taurine >> alanine/serine I. Aspartate 1. Miscellaneous a. Primarily localized to the ventral spinal cord b. Opens an ion channel c. Excitatory NT, which increases the likelihood of depolarization in the postsynaptic membrane d. Opposite function of glycine in the spinal cord e. Aspartate (+) and glycine (2) form an excitatory/inhibitory pair in the ventral spinal cord. f. Nonessential amino acid found particularly in sugar 2. Inactivation: reabsorption into the presynaptic membrane J. Histamine 1. Miscellaneous a. Histamine acts as an NT and is found in mast cells (but histamine of mast cells is not an NT). b. Highest concentration within hypothalamus 2. Synthesis 3. Receptors a. Histamine1 receptor b. Histamine2 receptor c. Histamine3 receptor: functions in autoregulation 4. Agonists/antagonists a. Histamine1-receptor antagonists i. Diphenhydramine ii. Chlorpheniramine iii. Promethazine b. Histamine2-receptor antagonists: cimetidine c. α-Fluoromethylhistidine: selective inhibitor of histamine decarboxylase K. Neuropeptides 1. Miscellaneous a. Most common NTs in the hypothalamus b. Very potent compared to other NTs c. May modulate postsynaptic effects of NTs by prolonging effect via second messengers d. Neuropeptides coexist with other NTs NT NEUROPEPTIDE GABA Somatostatin Cholecystokinin Ach Vasoinhibitory peptide (VIP) Substance P NE Somatostatin Enkephalin Neuropeptide Y DA Cholecystokinin Neurotensin Epinephrine Neuropeptide Y Neurotensin 5-HT Substance P Enkephalin Vasopressin Cholecystokinin Dynorphin Oxytocin Enkephalin 2. Synthesis: ribosomal synthesis 3. Inactivation: extracellular action is terminated via hydrolysis by proteases and diffusion; not inactivated by reuptake. 4. Subtypes a. Enkephalins i. Enkephalin receptor (A) Opiates and enkephalins bind to the receptor. (B) Highest concentration found in the sensory system, limbic system, hypothalamic region, amygdala, and periaqueductal gray (C) Located on presynaptic synapses ii. Opiates and enkephalins inhibit the firing of locus ceruleus neurons. L. Opioids 1. Receptors a. k Receptor differs from µ and δ receptors because it cannot reverse morphine withdrawal. M. Substance P 1. Release a. Ca2+ dependent b. Inhibited by morphine 2. Agonists/antagonists a. Capsaicin: depletes substance P (analgesic effect) N. Quick reference for NTs NT SYNTHESIZED FROM SITE OF SYNTHESIS ACh Choline CNS, parasympathetic nerves 5-HT Tryptophan CNS, chromaffin cells of gut, enteric cells GABA Glutamate CNS Glutamate — CNS Aspartate — CNS Glycine — Spinal cord Histamine Histidine Hypothalamus Adenosine ATP CNS, peripheral nerves Adenosine triphosphate — Sympathetic, sensory, and enteric nerves Nitric oxide Arginine CNS, gastrointestinal O. Other 1. Quisqualate-type receptor is coupled to phospholipase C. 2. CNS sites of high neurochemical concentrations a. NE: locus ceruleus b. 5-HT: median and dorsal raphe c. DA: substantia nigra d. GABA: cerebellum e. Cholinergic: substantia innominata and nucleus basalis of Meynert f. Histamine: hypothalamus 3. Calmodulin: prominent calcium-binding protein in the CNS 4. Ascending pathways mediating arousal II. Neurochemistry A. Electrolyte concentrations ION INTRACELLULAR CONCENTRATION (mEq/L) EXTRACELLULAR CONCENTRATION (mEq/L) Na+ 15 140 K+ 135 4 Ca2+ 2 3 10−4 4 Mg2+ 40 2 Cl− 4 120 HC03− 10 24 B. Basic neurophysiology 1. Action potential (AP) a. Definition: a self-propagating regenerative change in membrane potential b. An AP only develops if the depolarization reaches the threshold determined by the voltage-dependent properties of the sodium channels; sodium channels are also time dependent, staying open for only a limited period. c. Ion fluxes and membrane potentials i. Most of the charge movement in biological tissue is attributed to passive properties of the membrane or changes in ion conductance. ii. Important cations: K+, Na+, Ca2+ iii. Important anions: Cl2, proteins d. Three phases i. Resting membrane potential (A) Potential = 270 mV (B) Due to difference in permeability of ions and sodium-potassium pump forcing K+ in and Na+ out (C) Resting membrane potential based on outward K+ current through passive leakage channels (D) If resting membrane potential is diminished and threshold is surpassed and the AP is generated ii. Depolarization (A) Potential = +40 mV (B) Dependent on sodium permeability (1) Voltage-gated opening of sodium channels (a) Sodium permeability increases as membrane potential decreases from the resting membrane potential (–70 mV) toward 0. (b) When the membrane potential reaches approximately –55 mV, sodium channels open dramatically. (c) The transient increase in sodium permeability allows results in membrane potential of +40 mV. (d) Voltage-dependent potassium channels will also open in conjunction with sodium channels. iii. Repolarization: closure of voltage-gated sodium channels reestablishes potassium as the determining ion of the membrane potential. e. Myelinated are faster than unmyelinated nerves i. Myelin decreases membrane capacitance and conductance and the time constant. ii. Increases the space constant of the segment of axon between the nodes of Ranvier iii. Velocity is proportional to axon radius. 2. Neuromuscular junction (NMJ) a. Presynaptic components i. Motor neuron ii. Axon iii. Terminal bouton (A) Synaptic vesicles: contain 5,000 to 10,000 molecules (1 quanta) of ACh (B) Release based on voltage-gated calcium channels b. Synaptic cleft: 200 to 500 µm c. Postsynaptic components i. Motor end plate ii. ACh receptors iii. Voltage-gated sodium channels 3. Synaptic transmission a. AP is based on sodium inward current and potassium outward current through voltage-dependent channels. b. When AP reaches presynaptic region, it causes release of NT. c. NTs bind to postsynaptic receptors, opening postsynaptic membrane channels. d. Depending on the ionic currents flowing through the transmitter (ligand)-operated channels, two types of postsynaptic potentials are generated. i. EPSPs (A) Occur when sodium inward current prevails (B) Increase the probability that AP will be propagated ii. IPSPs (A) Occur when potassium outward current or chloride inward current prevails (B) Cause hyperpolarization of the postsynaptic membrane, making it more difficult to reach the threshold potential e. Summation i. EPSPs and IPSPs interact to determine whether AP is propagated postsynaptically. ii. Temporal summation: EPSPs/IPSPs sequentially summate at a monosynaptic site. iii. Spatial summation: EPSPs/IPSPs simultaneously evoke an end-plate potential polysynaptically. f. Depolarization of the nerve terminal results in opening of all ionic channels, including those for calcium; calcium entry causes release of NT from the presynaptic terminal, which binds to postsynaptic receptor sites. g. Chemical transmission is the main mode of neuronal communication and can be excitatory or inhibitory (if postsynaptic binding opens sodium channels and/or calcium channels → EPSP; if it opens potassium channels and/or Cl channels → IPSP); most common excitatory NT is glutamate; common inhibitory NTs are GABA and glycine. C. Membrane channel dysfunction 1. Sodium channel a. Sodium channel inhibitors i. Tetrodotoxin (puffer fish) ii. Saxitoxin (dinoflagellate, shellfish) b. Sodium channel potentiators i. Batrachotoxin (arrow poisoning) ii. Grayanotoxin (Amazon amphibians) c. Sodium channel closure inhibitors i. Scorpion toxin ii. Sea anemone toxin d. Mutational disorders i. Failure of sodium channel to inactivate ii. Disorders (A) Hyperkalemic periodic paralysis (B) Paramyotonia congenita 2. Potassium channel a. Antagonists i. Tetraethyl ammonium chloride: voltage-gated potassium channels ii. 4-Aminopyridine: antagonizes fast voltage-gated potassium channels b. Mutational disorders: hypokalemic periodic paralysis 3. Calcium channel disorders a. Absence seizures: thalamic calcium channels b. Hypokalemic periodic paralysis
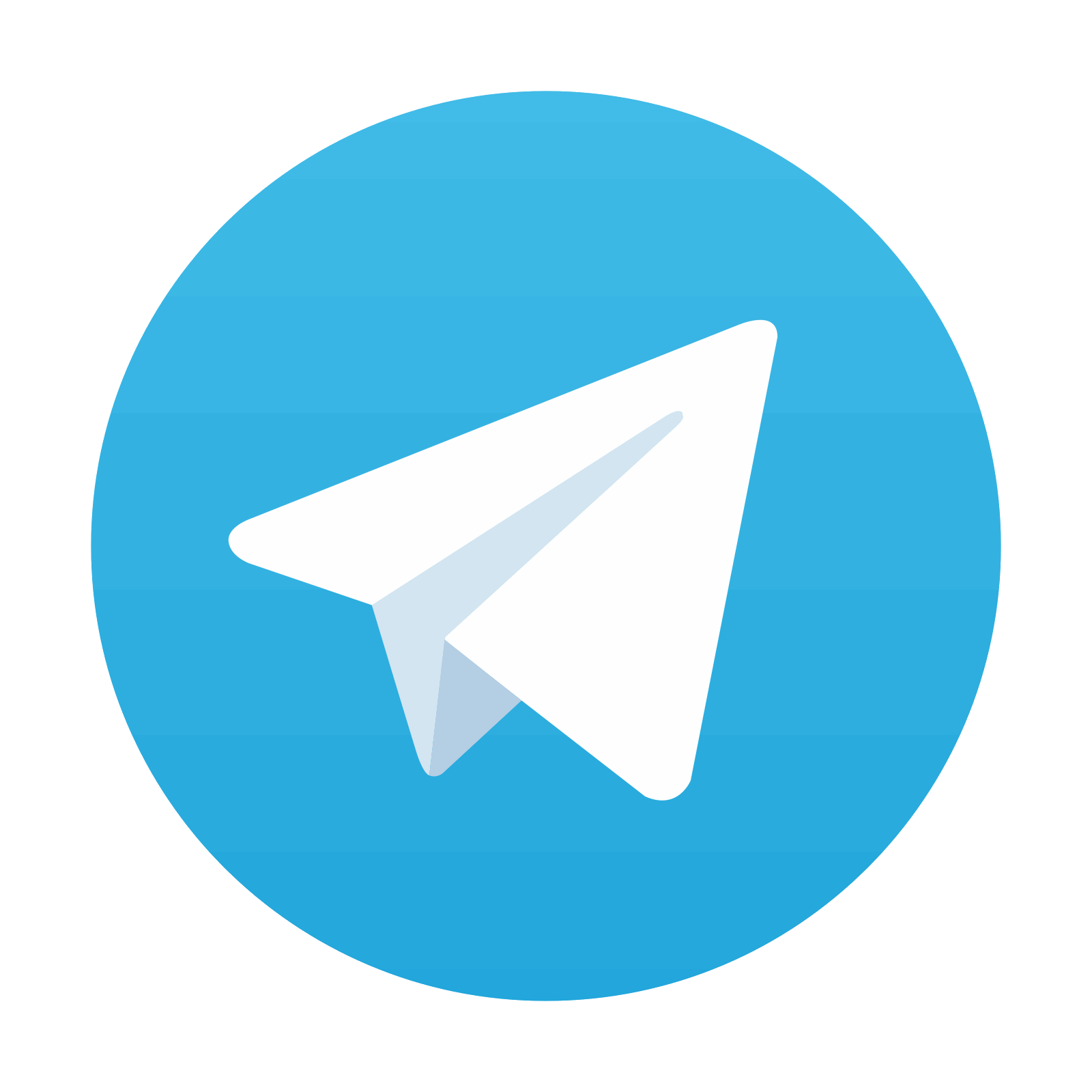
Stay updated, free articles. Join our Telegram channel

Full access? Get Clinical Tree
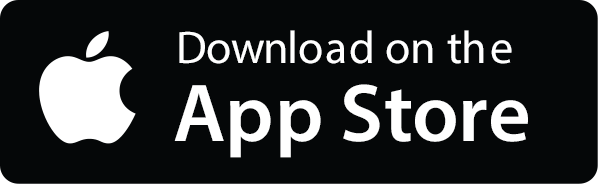
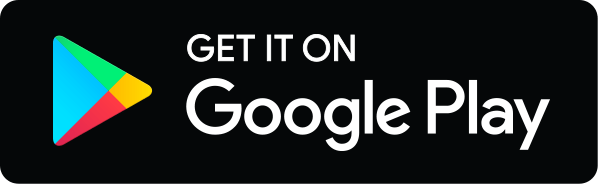