Chapter 8 Neurodegenerative Diseases and Hydrocephalus
Let us start with the basics. Atrophy reflects the loss of brain tissue, be it cortical, subcortical, or deep. With the loss of cell bodies in the cortex (gray matter), axonal wallerian degeneration occurs with white matter atrophy or demyelination. Selective atrophy of the white matter may also occur with perivascular small-vessel insults. Generally, there is no treatment for atrophy; what’s gone is gone. Therefore, beware of overcalling atrophy. Remember that certain drugs (steroids) or metabolic states (dehydration, alcoholism) may cause an appearance of increased cerebrospinal fluid (CSF) spaces, suggesting atrophy, but are potentially reversible (Box 8-1).
Until you have a good sense of what the normal brain looks like at all ages, be hesitant to label a brain “atrophic.” You should use the terms age-related involutional changes or volume loss appropriate for age. Normally functioning elderly persons may get offended (if not litigious) at the neuroradiologist who labels their brains “atrophic.” There is a spectrum of normal brain parenchymal volume for any age (Fig. 8-1). Remember also that men have more prominent sulci at most ages than women.
Computed tomography (CT) or magnetic resonance (MR) imaging findings that suggest hydrocephalus over atrophy are summarized in Table 8-1 (Fig. 8-2). At a first glance, the presence of dilation of the chiasmatic, infundibular, and suprapineal recesses of the third ventricle; rounding of the frontal horns; convexity to the third ventricle; expansion of the temporal horns; effacement of sulci; enlargement of ventricles out of proportion to sulcal dilation; periventricular smooth high signal representing transependymal CSF exudation (best seen on fluid-attenuated inversion recovery [FLAIR]); marked accentuation of the aqueductal signal void; narrowing of mamillopontine distances; and associated papilledema are indicative of hydrocephalus (Fig. 8-3).
Table 8-1 Differentiation of Hydrocephalus and Atrophy
Characteristic | Hydrocephalus | Atrophy |
---|---|---|
Temporal horns | Enlarged | Normal except in Alzheimer disease |
Third ventricle | ||
Fourth ventricle | Normal or enlarged | Normal except with cerebellar atrophy |
Ventricular angle of frontal horns on axial scan | More acute | More obtuse |
Mamillopontine distance | <1 cm | >1 cm |
Corpus callosum | ||
Transependymal migration of cerebrospinal fluid | Present acutely | Absent (rule out ischemia) |
Sulci | Flattened | Enlarged out of proportion to age |
Aqueductal flow void | Accentuated in normal-pressure hydrocephalus | Normal |
Choroidal-hippocampal fissures | Normal to mildly enlarged | Markedly enlarged in Alzheimer disease |
Sellar changes | Erosion of floor and ballooning of sella | None |
HYDROCEPHALUS
Overproduction of Cerebrospinal Fluid
Classically, it was stated that patients with choroid plexus papillomas and choroid plexus carcinomas have hydrocephalus based on the overproduction of CSF (see Fig. 3-24). Increasingly, this hypothesis has come into question because it is believed that some cases of hydrocephalus may in fact be due to obstruction of the arachnoid villi or other CSF channels secondary to adhesions from tumoral hemorrhage, high protein levels, or intraventricular debris. This is particularly true with fourth ventricular choroid plexus papillomas, which generally tend to obstruct the sites of egress of the CSF in the foramina of Luschka and Magendie. In the cases of lateral ventricle choroid plexus papillomas (particularly in the pediatric population), the overproduction of CSF may be the cause of hydrocephalus.
Noncommunicating Hydrocephalus
Obstructive hydrocephalus may be separated into noncommunicating and communicating forms. Noncommunicating forms are due to abnormalities at the ventricular outflow levels (Box 8-2). Communicating hydrocephalus is due to abnormalities at the level of the arachnoid villi or blockage at the incisura of the foramen magnum. Noncommunicating types often need brain surgery to remove offending agents; communicating types respond best to shunts.
Causes of Obstructive Hydrocephalus
Colloid Cyst
The classic cause of obstruction at the foramina of Monro is the colloid cyst (see Fig. 3-69). This is typically located in the anterior region of the third ventricle. On CT, the lesion is high in density before enhancement. MR often shows a lesion that is high intensity on T1-weighted (T1WI) and T2-weighted images (T2WI). The signal of colloid cysts is variable, depending on the protein concentration, hemorrhage, and other paramagnetic ion effects.
Congenital Aqueductal Stenosis
Sagittal MR is essential for distinguishing extrinsic mass compression from an intrinsic aqueductal abnormality (Fig. 8-4). Aqueductal stenosis may also be diagnosed on CSF flow imaging. The normal aqueductal signal in this case should be bright, signifying CSF flow. With aqueductal obstruction, a dark signal is seen on the flow scan (Fig. 8-5). Phase-contrast MR with a velocity encoding set to 10 to 15 mL/sec may be the best way to assess aqueductal patency. Interstitial edema, bright on a FLAIR scan, will also be present in the periventricular zone.
Masses or Tumors
Masses of the pineal gland are the most common causes of extrinsic obstruction of the aqueduct. These generally compress the aqueduct from posteriorly and cause dilatation of the lateral and third ventricular system. Tectal gliomas also obstruct the aqueduct early in their course (Fig. 8-6). Occult cerebrovascular malformations may occur there as well. The base of the aqueduct may be obstructed by tumors of the posterior fossa such as medulloblastomas (PNETs) or ependymomas.
Trapped Ventricles
Isolation of the fourth ventricle may occur when the aqueduct of Sylvius and the foramina of Magendie and Luschka are occluded. The fourth ventricle becomes “trapped” and will expand as CSF production by the choroid plexus continues unabated (Fig. 8-7). This expansion may compress the cerebellum and brain stem and lead to posterior fossa symptoms.
Communicating Hydrocephalus
Infection, Hemorrhage, Tumors
The arachnoid villi are sensitive, delicate structures that may get gummed up by insults of several causes, resulting in communicating hydrocephalus (Box 8-3). Think of them as the little fenestrations in your bathtub drain: The whole tub will overflow if these tiny conduits are obstructed. The most common causes of obstruction include infectious meningitis, ventriculitis, ependymitis, subarachnoid hemorrhage, and carcinomatous meningitis. As the CSF becomes more viscous with a higher protein concentration, the arachnoid villi lose their ability to reabsorb the fluid. This causes hydrocephalus with dilation of the ventricular system.
Box 8-3 Causes of Communicating Hydrocephalus
Normal-pressure Hydrocephalus /Adult Hydrocephalus
Normal-pressure hydrocephalus (NPH) has a classic triad of clinical findings: gait apraxia, dementia, and urinary incontinence (Box 8-4). We like to think of NPH as a treatable cause of a nontraumatic DAI (dementia, ataxia, and incontinence). Although NPH was initially described as being idiopathic, patients with a remote (cryptic) history of infection or hemorrhage are still lumped into this category when they have the clinical triad. This probably accounts for 50% of NPH cases. The patients have enlarged ventricles from communicating hydrocephalus with particular enlargement of the temporal horns. They may show evidence of transependymal CSF leakage on MR or CT. MR often shows accentuation of the cerebral aqueduct flow void (Fig. 8-8). These patients may respond to shunting procedures with amelioration of their clinical symptoms. The most accurate predictors of a positive response to shunting are (1) absence of central atrophy or ischemia, (2) gait apraxia as the dominant clinical symptom, (3) upward bowing of the corpus callosum with flattened gyri and ballooned third ventricular recesses, (4) prominent CSF flow void, and (5) a known history of intracranial infection or bleeding (nonidiopathic NPH). It is important to entertain this diagnosis because there is a chance at the possibility of return of function with a shunt.
In patients with suspected NPH, an indium-111 DTPA (diethylene triamine penta-acetic acid) study is sometimes ordered. The agent is instilled in the CSF through a lumbar puncture. Normally, the tracer is resorbed over the convexities without ventricular reflux within 2 to 24 hours. In cases of communicating hydrocephalus and NPH, reflux of the tracer into the ventricles is seen with lack of tracer accumulation over the convexities 24 to 48 hours after instillation (Fig. 8-9). Patients who demonstrate this scintigraphic appearance allegedly have a better response to shunting than patients with normal or equivocal indium-111 DTPA findings.
The rate of clinical improvement after shunting in patients with NPH is still only 50%. Prominence of the CSF flow void in patients with this condition has led some investigators to use phase-contrast MR techniques to measure the flow through the cerebral aqueduct. A stroke volume of greater than 42 mL was predictive of better response to shunting. The specific parameters inherent in this measurement are related to scanner field strength and pulse sequences, so they are not necessarily transferrable to your own scanner, but the point made is that greater flow through the aqueduct means a better chance for shunt improvement. The rationale for this is that the brain is pushed centrifugally in NPH from enlarged ventricles (Fig. 8-10). As systole occurs, the blood being pumped into the closed space of the cranium forces CSF out of the lateral ventricles and into the aqueduct. Expansion of the lateral ventricles leads to shearing strains on the white matter and clinical symptoms.
External Hydrocephalus
Another benign cause of hydrocephalus from arachnoid villi malfunction is “external hydrocephalus,” also referred to as benign enlargement of the subarachnoid spaces in infants, benign extra-axial collections of infancy, extraventricular obstructive hydrocephalus, benign subdural effusions of infancy, and benign macrocephaly of infancy. This may be due to immaturity of the arachnoid villi with a decreased capacity to absorb CSF. External hydrocephalus is typically seen in children younger than age 2 years who have a rapidly enlarging head circumference, going off the scale on the pediatrician’s graph. Neurologically, these children are normal. Prematurity (immaturity), a history of intraventricular hemorrhage, and some genetic syndromes predispose to external hydrocephalus. CT or MR shows dilation of the CSF spaces over the frontal lobes and along the interhemispheric fissure but with relatively normal-sized ventricles (Fig. 8-11). The disorder usually resolves by the time the child is 3 to 4 years old and the head circumference returns to normal. The ventricles are usually normal in size (64% of cases)—thus the term external hydrocephalus. It is the CSF spaces external to the ventricles that are dilated. Why do the ventricles fail to dilate? Presumably because the cranial sutures are open, so the head enlarges instead. The differential diagnosis includes chronic subdural hygromas and atrophy caused by previous injury. When one sees sulcal dilatation and vessels coursing through the CSF collection, chronic subdural hygromas are much less likely (see Fig. 6-1). Atrophy is not usually associated with an enlarged head circumference.
ATROPHY
Cerebral Atrophy
The disorders that demonstrate gross supratentorial atrophy are often associated with dementia (Table 8-2).
Alzheimer Disease
Of the disorders in Table 8-2, Alzheimer disease (dementia Alzheimer type, or DAT) is one of the most notorious and common, accounting for 60% to 90% of the dementing disorders with progressive memory loss. DAT affects 2 to 4 million Americans; 8% of the population older than age 65 years and 30% of those older than age 85 years. Women are more commonly affected by a 2:1 margin. With DAT, life can be fascinating or petrifying, because memory is severely affected. DAT usually occurs in the late middle-aged adult, with the major dysfunction noted in memory, personality, and thought. Olfaction is one of the first senses to show some effects of the disease, but this is rarely tested. The patient’s social skills, personality, and speech pattern are also affected early. Depression often coexists. Late in the course the patient becomes severely impaired, myoclonic, vegetative, and weak. Current treatments are variably effective.
One study found that the measure with the best sensitivity in discriminating DAT patients from control subjects was the width of the temporal horn. If one combines the measure of width of the temporal horn, width of the choroid fissure, height of the hippocampus, and the interuncal distance into a compound factor, one can discriminate patients with mild DAT from control subjects with 86% sensitivity (Fig. 8-12). There may, in fact, be a continuum of progressive hippocampal atrophy between normal elderly, those with mild cognitive impairment (MCI), and those with DAT. Yet another volumetric study showed that the best correlation with progression to Alzheimer disease is the change in volume of the entorhinal cortex and inferior parietal lobule over time (even more so than the hippocampus).
< div class='tao-gold-member'>
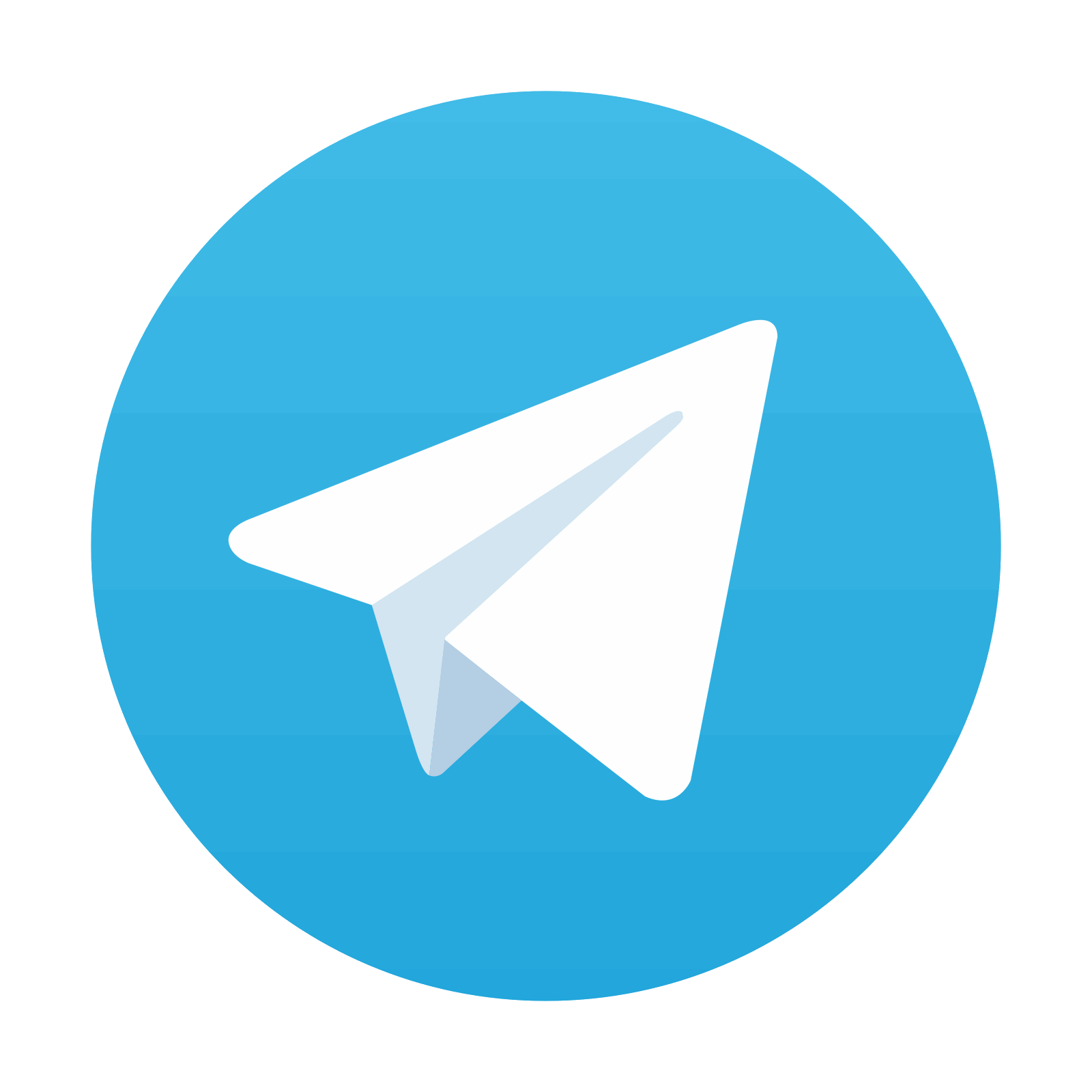
Stay updated, free articles. Join our Telegram channel

Full access? Get Clinical Tree
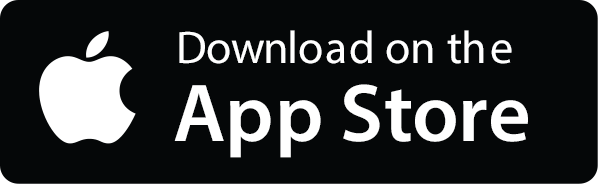
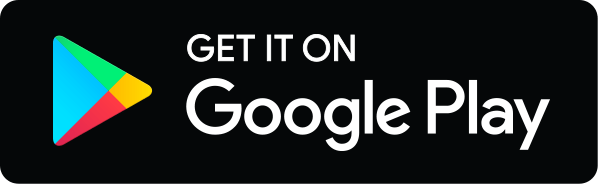