Neuroendoscopy
The field of neuroendoscopy has undergone an evolution in the past several decades, with increased attention given to minimally invasive approaches to various intracranial and spinal pathologies. With improved instrumentation, neurodiagnostic imaging, and surgical experience, pediatric neurosurgeons are now aware of the benefits and limitations of neuroendoscopy. Accompanying the improvement in surgical techniques and equipment is an ever-expanding base of scientific literature to help define patient selection, determine surgical outcomes, and describe potential complications and their management. Recent publications utilizing neuroendoscopic procedures have grown exponentially in the last decade and continue to expand in their diverse central nervous system (CNS) applications. Neuroendoscopy has been transformed as a field and is now part of a pediatric neurosurgeon’s armamentarium, with neuroendoscopy incorporated into cerebrospinal fluid (CSF) diversion, tumor resections, intracranial cyst fenestrations, skull base approaches, and spinal surgeries. There is also a growing role for endoscopically assisted microneurosurgery in these aforementioned surgeries.
This chapter focuses primarily on intracranial approaches with neuroendoscopy, and there is some mention of its use in spinal surgery. The current instruments frequently employed in endoscopic approaches are discussed, as well as the orienting anatomy that is identified throughout the neuroendoscopic procedures. The current applications of endoscopy are also described, along with surgical decision making regarding the limitations of endoscopy. Finally, potential complications arising during surgery and strategies for their management are discussed.
11.1 History
The roots of neuroendoscopy actually begin within the field of urology. Victor Darwin Lespinasse, a urologist in Chicago, utilized a rigid cystoscope to fulgurate the choroid plexus in two infants with hydrocephalus. Walter Dandy, considered to be the father of neuroendoscopy, began utilizing this technique to extirpate the choroid plexus in cases of congenital hydrocephalus.1 During his procedures, Dandy initially used a small nasal speculum to gain to access to the posterior horns of the lateral ventricles and drain the CSF for access to the choroid plexus. Later, Dandy developed long-handled surgical instruments to accompany electrocautery in his endoscopic transventricular surgeries. Dandy developed an open form of third ventriculostomy for the diversion of CSF. The first endoscopic third ventriculostomy (ETV), however, was performed by W. Jason Mixter.2 He developed the procedure in the cadaver of a child with hydrocephalus and translated his approach to patients. Around this time, in 1923, endoscopic photography was developed at the University of Pennsylvania by Fay and Grant, who captured the first endoscopic images of the choroid plexus.2,3
These early innovations paved the way for more advanced optics and instrumentation, which were subsequently developed for cauterizing the choroid plexus in infants with hydrocephalus. Scarff at the Neurological Institute of New York at the Columbia University College of Physicians and Surgeons pioneered angled lenses4 to improve the field of view and utilized a monopolar electrode that could be advanced and retracted in a working channel of the endoscopic sheath.5 Scarff was able to irrigate the field and perform surgery at a fluid-filled interface, a technique that had been developed earlier by Putnam1 to improve the primitive optics available at the time.6 Despite these adaptations, neuroendoscopy had a limited role in the treatment of hydrocephalus and was hindered by the considerable morbidity and mortality associated with these cases.7 With the introduction of the valved ventricular shunt in 1949 to treat hydrocephalus, the use of endoscopy declined.8
Unexpectedly, the same development that helped endoscopy fade from mainstream neurosurgery also helped it return to a critical role in pediatric neurosurgery.9 The complications of infections and shunt malfunctions associated with CSF shunt systems remain a persistent source of morbidity and mortality in children, and in an effort to reduce the shunt burden in children, neuroendoscopy10–13 has seen a resurgence in its use in the past two decades.7 In addition, instrumentation and optics have improved significantly and are now tailored to intracranial applications. With renewed interest, neurosurgeons employing neuroendoscopy14 are now exploring the boundaries of minimally invasive applications in various neurosurgical settings.
11.2 Instruments
11.2.1 Flexible and Rigid Endoscopes
The selection of endoscopes is critical for determining the types of surgical procedures that pediatric neurosurgeons undertake. The selection of available endoscopes is more diverse than the early cystoscopes, and innovation has been largely driven by technological improvements in digital imaging, optics, and minimally invasive surgical techniques. The types of endoscopes available can be generally classified as rigid and flexible, each having its advantages and disadvantages. Rigid endoscopes have traditionally had superior optics, relying on the segmental optics first pioneered by Harold Hopkins,15,16 an English physicist, and the optics are now coupled to a high-intensity light source, such as xenon. The rigid endoscope is introduced through a rigid sheath that can be equipped with several working channels to facilitate the introduction of microsurgical instrumentation as well as the ingress and egress of CSF and saline to flush debris and improve visualization. Adding these channels allows a fluid interface to be maintained throughout the surgery and ensures that the intracranial pressure (ICP) is kept at an atmospheric level, thereby preventing the accumulation of intracranial fluid and the resulting increase in ICP. Rigid endoscopes17 can also be outfitted with various angled lenses to widen the field of view around certain structures. Care must be taken when changing these lenses and steering through the ventricles and intracranial contents as orientation can be changed depending upon the lens angle selected.18 Additionally, rigid endoscopes and their sheaths are now available in a variety of sizes to accommodate neonatal pathologies.19
Rigid endoscopes, however, lack the maneuverability of their flexible counterparts. Flexible endoscopes can be used to peer around structures20 with little movement at the cranial surface or translational movement through the cortex. Flexible endoscopes utilize fiberoptic technology to permit maneuverability, but this currently limits the amount of transmitted light. Flexible endoscopes21 require a different steering technique from rigid endoscopes to ensure that anatomical orientation22 is preserved throughout the procedure so as not to damage adjacent neural structures. Takanori Fukushima23,24 in Tokyo, Japan, is credited with pioneering the use of the flexible endoscope, primarily for biopsies of intraventricular lesions.15 Flexible endoscopes can now be used together with rigid endoscopes25 in a scope-within-scope setup26 in which the neurosurgeon can capitalize on the benefits of each scope.27
11.2.2 Endoscope Holders; Digital and Three-Dimensional Imaging
A frequently overlooked aspect of neuroendoscopy is that a team,28 in which an additional surgeon or a trained assistant is needed to manipulate the scope with an extra set of hands, often performs the surgery. It is advantageous to have one surgeon hold and manipulate the endoscope while another works with the instruments. To support the endoscopes and minimize translational movement through the exposed cortical surface, endoscopic holding arms have been invented that allow the operating surgeon to work alone (▶ Fig. 11.1). Pneumatically operated arms29,30 can be sterilely draped in the operative field and fixed in a locale that allows delicate microsurgical manipulation with a decreased risk for awkward movements or inadvertent changes in depth or direction throughout a procedure.31 In addition to rigid fixation of the endoscope, recent technology has made possible three-dimensional neuroendoscopy,2 and improved digital imaging has become available and is moving two-dimensional images closer toward those seen during open microsurgery.32 Digital imaging ensures high-quality reproduction of the represented anatomy for the surgical team, and the addition of three-dimensional imaging allows an improved appreciation of the relationships between neural structures. Three-dimensional imaging relies on two parallel cameras in the endoscope to display the digital images for three-dimensional reproduction.31 Regardless of the dimensionality used, digital images and movies allow better patient education regarding planned surgery and surgeon training in preparation for the encountered anatomy.
Fig. 11.1 Intraoperative picture of a pneumatic endoscope holder that has been sterilely draped and utilized to hold the neuroendoscope.
11.2.3 Surgical Instruments and Lasers
To accompany the scopes and digital imaging, surgeons have designed microinstruments33 to facilitate dexterity during neuroendoscopic procedures.26 These instruments are introduced through the working channels of the sheath and are separate from the irrigation ports for CSF and saline ingress and egress. The tools available to the neuroendoscopist continue to expand yearly, and they are designed for grasping, cutting, and sampling tissue.6 Rigid and flexible probes allow penetration through cyst walls,34 whereas balloon catheters35 allow the controlled dilation of openings made for ETVs,36,37 cyst fenestrations,38,39 septostomies,40 and aqueductoplasties.20,21,41 Electrocautery with monopolar42,43 or bipolar current facilitates hemostasis and makes possible the manipulation of vascularized tissues, such as tumors, cyst walls, and choroid plexus.29,44 Multiple working channels on the sheath facilitate the use of two instruments concurrently to manipulate tissue.
Endoscopes may be outfitted with lasers45 to permit cutting through tissues. The lasers most commonly utilized in neuroendoscopy are the neodymium-doped yttrium–aluminum–garnet (Nd:YAG) laser, the argon laser, and the potassium titanyl phosphate (KTP) laser.4,6,46 The Nd:YAG laser emits light with a wavelength of 1.064 µm, which is in the near-infrared spectrum, and the light is aimed with a visible helium–neon pilot beam. Nd:YAG lasers are used to cut or coagulate pigmented tissues, as laser absorption is preferential in these tissues.47 KTP lasers48 emit light with a wavelength of 0.532 µm, which is within the visible spectrum.49 This laser can be used for dissecting because less thermal injury is produced.2
11.2.4 Stereotactic Endoscopy
Stereotactic navigation50 and intraoperative real-time anatomical localization continue to improve neuroendoscopic surgeries. Preoperative high-resolution magnetic resonance (MR) imaging and computed tomography (CT) can be fused to allow pediatric neurosurgeons to plan surgical approaches and identify critical vascular structures that might otherwise limit their resections or exposures.51 During endoscopic surgery, there is always the potential for disorientation and potential injury to uninvolved anatomical structures. Multiloculate hydrocephalus52,53 requiring multiple cyst fenestrations for the communication of fluid spaces is a condition in which intracranial structures are difficult to differentiate from one another. Stereotactic localization54,55 helps orient the surgeon to the proper cyst wall and potential underlying structures. In addition, in transnasal surgeries,56 preoperative CT of the skull base and maxillofacial region allow intraoperative midline orientation57,58 during attempts to identify the floor of the sella turcica and differentiate between anatomical variants with septate bone. Midline cranial approaches, such as transcallosal59,60 surgery, may also benefit from stereotactic preoperative localization, in which venous lakes that communicate with the superior sagittal sinus are identified and intracranial entry can be planned away from these large cortex-draining venous structures.61,62 In addition, pediatric neurosurgeons, in an effort to reduce the amount of cortical injury in children, can plan approaches to minimize the length of the endoscopic trajectories used to reach a targeted region.
Intraoperative fluoroscopy30,63 and ultrasound may also be used if guidance systems are not available or become dysfunctional during surgery. In children with open fontanels, ultrasound53,64,65 over the anterior fontanel can be used to visualize the endoscope in relation to the underlying pathology.66 One potential limitation of neuronavigation is brain shift and the collapse of fluid cysts or other fluid spaces once the endoscope has been introduced. Surgeons must be careful to correct for these changes during endoscopic procedures. Like all technology, stereotactic localization must be coupled with a working knowledge of anatomy to ensure that the information being displayed fits the anatomical endoscopic picture.
11.3 Endoscopic Anatomy
The following section focuses primarily on the intracranial anatomy encountered during transventricular approaches with the endoscope.20,67,68 There are key structures that need to be identified when the endoscope is introduced into the ventricles, and they serve as beacons throughout the case, reminding the surgeon of adjacent neural and vascular structures. Caution must be taken, however, as the normal anatomy may be obscured or distorted by the pathology of interest. If this occurs, neuronavigation with stereotactic localization may be utilized to verify the anatomy. In general, cranial endoscopy is safest if performed in the presence of ventriculomegaly, which allows the surgeon adequate visualization of the anatomical structures and room to maneuver the endoscope. If small ventricles are present, stereotactic localization may aid in cannulation of the ventricles.69,70 Before cannulation, proper scope orientation must be verified to ensure that the lens is not mistakenly inverted from a desired orientation.63 This allows proper orientation throughout the case and prevents the inadvertent manipulation of tissue. In addition, when a transventricular approach to a lesion is used, a small needle with a stylet may be introduced into the cortex and ventricle to ensure that the planned trajectory and orientation traverse the ventricle.71 With the stylet72 removed and CSF appreciated, the endoscope can then be introduced along the trajectory of the needle as it is removed.
In general, the structures viewed with an endoscope look different from those visualized with a stereoscopic operating microscope.73 The location of structures is relatively constant intracranially, but care must be taken for structures that lie outside the limited field of view while the endoscope is maneuvered. This becomes particularly important in depth perception, in which other signals, such as brightness, shadowing, and response to infusion of saline, will help the surgeon determine a structure and estimate its depth.2
The first structure that the endoscope encounters after penetrating the cortical surface and ventricular walls is often the floor of the lateral ventricle, which has a smooth, white ependymal surface. Here, the frondlike vascularized choroid plexus can be appreciated, lying in the choroidal fissure and leading anteriorly to the medial floor of the lateral ventricle. The choroid plexus then dives into the foramen of Monro and covers the roof the third ventricle posteriorly.67,74 The foramen of Monro serves as a landmark for endoscopists as it often guides surgeons to the proper location for an ETV or to the inspection of third ventricular lesions or the cerebral aqueduct (▶ Fig. 11.2). The foramen of Monro may be obscured, however, by intraventricular pathology, such as tumors, infections, or cysts. Identifying the choroid plexus overlying the thalamus in the choroidal fissure and leading to the foramen of Monro can help verify its location. An additional landmark that may be seen medially is the septum pellucidum, which may be patent or intact depending on the particular case. The head of the caudate nucleus may be seen lateral to the foramen of Monro. Neurovascular structures also serve as a roadmap intraventricularly, where the thalamostriate vein, coursing alongside the choroid plexus, joins the anterior septal vein at the foramen of Monro to form the internal cerebral vein.
Fig. 11.2 Intraoperative anatomy seen during neuroendoscopy after the lateral ventricles have been entered. The foramen of Monro can be seen at the center of the image with the choroid plexus entering the third ventricle. The thalamostriate vein can be seen surrounding the posterior opening to the foramen of Monro, while the roof of the foramen is composed of fibers from the fornix. Damage to these fibers can be responsible for postoperative memory impairment.
Structures surrounding the foramen of Monro include the c-shaped fornices arching over the foramen. These fiber bundles contain efferent projections from the hippocampus and subiculum and define the anterior and medial borders of the foramen of Monro. The fibers terminate in the mammillary bodies on the floor of the third ventricle, where they appear as white prominences. Great care must be taken not to disturb the fornices when the third ventricle is entered and the endoscope is manipulated.75 As the third ventricle is entered, the anterior portion is appreciated, with the infundibular recess and anterior to it the optic recess. The tuber cinereum, the portion of the third ventricle between the mammillary bodies and the infundibular recess, is seen along with a vascular blush along the third ventricular floor. The tuber cinereum can be thinned out and stretched in the presence of hydrocephalus and may permit a view of the apex of the basilar artery in the interpeduncular cistern. The posterior third ventricle contains the aqueduct of Sylvius and posterior commissure, observation of which may be obstructed by a large massa intermedia. Visualization of these structures often requires changing the lens from a 0-degree scope to a 30-degree angled lens and turning a rigid endoscope around to look posteriorly.
11.4 Endoscopic Procedures
Intracranial endoscopic procedures can largely be classified as intraventricular and extraventricular applications. Conventional intracranial approaches utilizing the endoscope employ a bur hole or small craniotomy access through either one or several openings. The applications of neuroendoscopy continue to expand76 in pediatric neurosurgery as investigators push the boundaries to define optimal patient populations.77 Patient selection78–80 is clearly correlated with outcomes in a variety of neuroendoscopic procedures and ultimately determines their success in addressing pathologies.7,36 These approaches are largely driven by efforts to reduce manipulation of the normal cortex and minimize injury to surrounding neural5 and vascular structures with a minimally invasive approach.81 Endoscopy also seeks to reduce the postoperative length of stay, the length of surgery, and the morbidities and blood loss associated with open techniques.82 In some areas of pediatric neurosurgery, there continues to be debate83 about the optimal approach and the role of neuroendoscopy.1 In the following sections, a variety of neuroendoscopic procedures are discussed, along with the indications for surgical intervention and the subset of children who are potentially best suited for minimally invasive surgery.
The operating room setup for each endoscopic case depends on the approach being used. In general, however, the surgeons manipulating the endoscope and instruments should have the monitor placed in front of them so that their gaze is fixed on the screen and their access to surgical instruments is not obstructed (▶ Fig. 11.3). The surgeon holding the endoscope should have adequate room to avoid movement or disruption of the endoscope, with one hand firmly grasping the scope and the other on or near the cranium, ensuring that inadvertent plunging of the endoscope does not occur while instruments are introduced into the working channel.66 When instruments are placed into the working channel, it is necessary to temporarily back out the endoscope a small distance to allow proper visualization of the instrument as it makes its way into the field of view. This prevents instruments from puncturing and damaging neural tissue outside the surgical field.66 Additionally, the operating surgeons should verify the orientation of the lens if it is changed or added to the endoscope and should ensure that CSF has a proper egress from the brain by opening a working channel or appropriate port in the endoscope.84 Finally, irrigation should be attached to the endoscope before the CNS is entered in order to avoid unnecessary twisting or manipulation of the endoscope. These small steps in endoscope setup help limit small-vessel or cortical hemorrhages throughout the endoscopic procedure.75
Fig. 11.3 The endoscope and viewing screens are set up in a way that allows the surgeon to have maximal visualization of the ongoing operative procedure while also having access to the surgical instruments. The surgeon maintains fixation on the screen to ensure that no intracranial structures are damaged during endoscopic manipulation.
11.4.1 Intraventricular Applications
Third Ventriculostomy
The history of endoscopic surgery1 to treat pathologies of the CNS is based upon the treatment of hydrocephalus with ETV.85 Since its first use in 1922 by Dandy86 as an open procedure, third ventriculostomy has undergone modification and is now incorporated into the realm of endoscopy as a standardly performed procedure.87 The indications88 for performing ETV normally include noncommunicating hydrocephalus with an open subarachnoid space for CSF uptake, although quantification of CSF absorption is difficult. Preoperative imaging64 with MR imaging or CT reveals the presence of hydrocephalus and can help to determine if aqueductal stenosis or additional pathology is responsible for the obstruction in CSF flow.89 MR imaging may reveal anatomical clues,90 such as inferior bowing of the third ventricular floor, and can identify if the prepontine space91 is adequate for passing a blunt probe through the floor of the third ventricle. The location of the basilar artery can also be appreciated on sagittal MR imaging.92 Additionally, preoperative cine phase-contrast MR imaging gated to the cardiac cycle and performed in a midsagittal plane can qualitatively measure CSF velocity and flow and further identify the source and degree of CSF obstruction.93,94
It was previously thought that in patients less than 1 year of age,16 myelomeningocele95 (MMC)–related hydrocephalus, small ventricles, and infection of the CNS were contraindications to ETV.96 However, studies97 in each of these patient populations have shown success98 in reducing the proportion of patients who are shunt-dependent, suggesting that patient selection may not be as straightforward as one would hope.99 Still, these more difficult scenarios must be taken into account when children and their parents are counseled as to the postoperative expectations with ETV.100
ETV is performed under general anesthesia, and the patient is positioned supine with the head placed on a headrest elevated to about 30 degrees. The surgeon and assistant stand at the patient’s head and look straight ahead at the digital monitor. If image guidance is being used, the authors prefer the head fixed in pin stabilization. The surgical site is prepared, and a small area in the midpupillary line just anterior to the coronal suture is marked about the coronal suture and make a bur hole or small craniotomy. The location of the bur hole may be adjusted by stereotactic localization in order to optimize the trajectory for making the third ventriculostomy and to observe any additional pathology that may be present.51 The authors prefer a more medial trajectory for ETV because it allows optimal visualization of the foramen of Monro and placement of the fenestration. The dura mater is opened, and the pia mater is coagulated and incised. The frontal horn of the lateral ventricle may be cannulated with a small needle or sheath of the endoscope to ensure that CSF is present.33 CSF can then be collected for marker studies and histology if needed. The obturator in the sheath is removed, the endoscope is outfitted with a camera, and a light source is introduced. Irrigation tubing connected to the endoscope port allows lactated Ringer solution to enter the CNS, and an open channel allows CSF and fluid to exit.66
The floor of the lateral ventricle and its ependymal surface are first appreciated along with the choroid plexus and foramen of Monro, which serve as landmarks for proceeding to the third ventricle (see ▶ Fig. 11.1).67 The fornix is identified, and care is taken to avoid injuring this structure with instruments or the manipulated endoscope, because patients with forniceal damage may present with retrograde amnesia and verbal memory impairment postoperatively.44 As the third ventricle is entered, the mammillary bodies are seen at the posterior aspect of the anterior floor along with the optic and infundibular recesses more anteriorly. The thin, translucent tuber cinereum contains only glial tissue and may be pulsatile from the underlying or adjacent basilar artery.101 A site of fenestration is chosen that is slightly more anterior14,102 than halfway between the mammillary bodies and the infundibular recess. It is imperative to avoid vascular injury to the underlying basilar artery.
A 4F Fogarty balloon catheter with stylet or rigid probe is then passed down the working channel of the endoscope so that the end of the catheter can be seen in the operative field of view before it impacts any nearby structures on the floor of the third ventricle. The 4F balloon catheter is used to augment the opening (▶ Fig. 11.4a).82,103 The floor is then punctured at the desired site, with care taken not to plunge beyond the area of visualization. The opening is widened with balloon insufflation (▶ Fig. 11.4b). The endoscope can then be used to inspect the opening to identify the Liliequist membrane,104 an arachnoid plane containing the basilar artery complex and separating the posterior fossa cisterns from the suprasellar cisterns (▶ Fig. 11.4c).68 This membrane must be opened to ensure proper connection between the cisterns and the flow of CSF through the ETV. When the basilar artery complex and perforators are seen clearly, the pulsatile flow of CSF can often be observed through the opening in the third ventricular floor.101 Care must be taken around the opening of the floor of the third ventricle as excessive stretch may damage nearby basilar artery perforators or the hypothalamus, creating subarachnoid hemorrhage or autonomic disturbances, such as bradycardia.75,81,105 Laser perforation106 has been utilized to open the floor of the third ventricle, but this may place adjacent vascular structures at increased risk for thermal injury during the procedure.45 The authors advise against the use of laser perforation of the third ventricular floor.
Fig. 11.4 Endoscopic third ventriculostomy. (a) A Fogarty balloon catheter has been introduced through the working channel of the neuroendoscope and is seen in the floor of the third ventricle. The opening for the ETV is made just superior to the paired mammillary bodies in the tuber cinereum of the floor of the third ventricle. The balloon catheter is dilated until the ETV opening is made. (b) The opening in the tuber cinereum in the floor of the third ventricle can be visualized and is superior to the paired mammillary bodies. The blush of the infundibulum can be seen superior to this opening. (c) An endoscopic view through the ETV opening; the basilar artery can be seen, along with the remnants of the Liliequist membrane.
The ventricles are inspected for any areas of hemorrhage and are copiously irrigated before removal of the endoscope. The patient is observed for 24 hours to ensure that flow through the ETV is adequate.101,107,108 Postoperative MR imaging109 can determine the site and patency of the ETV, especially when it is combined with cine flow studies.94 These images may be important to establish a new baseline for the affected child during subsequent evaluations. The ETV may not immediately affect ventricular size, and follow-up examination 6 weeks to 2 months later may reveal resolution of the ventriculomegaly.37 Children may be followed intermittently110 in the postoperative period with MR imaging to ensure that ETV failure has not occurred. Long-term follow-up100 may be required in certain cases as stomas in the floor of the third ventricle may lose patency, causing recurring symptoms and signs of elevated ICP.11 In the setting of ETV failure, reinspection with an endoscope is warranted to determine the patency of the previous ETV and possible reopening of the closure. Occasionally, the stoma may be closed from gliosis111 or infection, or plugged with cellular or proteinaceous debris, necessitating opening and exploration of the floor of the third ventricle. If the ETV is found to be patent, additional procedures, such as shunt insertion, may be required for poor CSF absorption in the subarachnoid space of the intracranial venous system.112
Outcomes113 of ETV vary depending on the definition of ETV success used by reports in the literature.114 ETV outcome studies describe primarily age and pathology as factors determining long-term outcome in children.14,103,115 ETV has also become a promoted therapeutic strategy for patients in underdeveloped countries82,116 to avoid the complications associated with shunts. The greatest success of ETV11,117 is seen in children with acquired, late-onset noncommunicating hydrocephalus resulting from tumor blockage118 of the aqueduct or fourth ventricle.119 The success rate for children meeting these criteria is as high as 80 to 90%.82,120–122 Lower success rates are reported in children less than 1 year of age110,117,123 or with a history that includes CNS infection, MMC, communicating hydrocephalus, the presence of a preoperative shunt system, or congenital aqueductal stenosis.14,115,123 The presence of a shunt system124 is not an absolute contraindication to attempting an ETV108; ETV may allow the child to become shunt-independent. Also, approximately 50% of neonates with an MMC95,125 may respond to ETV, so that they are liberated from shunt placement in a disease that was once thought to be synonymous with shuntable hydrocephalus.122 ETV for children with an MMC81,95 may be complicated by the abnormal intraventricular anatomy that is frequently encountered, necessitating conversion to placement of a ventriculoperitoneal shunt system. Such anatomical limitations include abnormalities in the skull base limiting the prepontine space91 and abnormal ventricular anatomy. The long-term cost-effectiveness10 of ETV in comparison to shunts, however, is an area of considerable debate, and additional studies are warranted83 to determine its use in various populations of children.10
Complications arising from ETV37,45,117,123 include hemorrhage126 from damage to the basilar artery, infection, midbrain injury, third nerve palsy, hemiparesis, neuroendocrine deficits from damage to the hypothalamic nuclei, subdural hematoma, fever unrelated to infection, and damage to the fornix resulting in memory impairment.12,81 Many complications127 are transient in nature and resolve in the immediate postoperative period on follow-up examinations.37,78,79,93,97,108
Multiloculate Hydrocephalus
Multiloculate hydrocephalus and isolated ventricles can result from any inflammatory126 or infectious128 process within the cerebral ventricles and can lead to trapped CSF spaces that complicate the clinical presentation and treatment of a child with hydrocephalus. Isolated ventricles may result from overdrainage of CSF in infants, leading to slit-ventricle syndrome and isolation of a lateral ventricle from the rest of the ventricular system.129 Equilibration of the ventricular system may not occur after shunt placement, and overdrainage of the ipsilateral ventricle may cause the contralateral ventricle to dilate. Similarly, if the aqueduct of Sylvius collapses, a dilated fourth ventricle may develop, compressing the adjacent brainstem. Postinflammatory hydrocephalus results from infection or hemorrhage within the CNS and can lead to scarring of the subarachnoid spaces, decreased CSF absorption, ependymal cyst formation, and obstruction of CSF flow.130 With its narrow gauge, the aqueduct of Sylvius is particularly vulnerable to occlusion from inflammation. Gram-negative ventriculitis and perinatal intraventricular hemorrhage may result in subependymal cyst formation that may later exert mass effect on the surrounding ventricles, impeding and ultimately obstructing CSF egress.48,131
Patients with numerous cysts are more complex cases than those with uniloculate cysts as these children may have undergone numerous shunt revision surgeries necessitating multiple catheter placements to control expanding cysts. The ultimate goals in treatment, however, are similar in both cases; the objective is to provide communication and CSF flow between all isolated compartments with the ventricular system. Shunt placement in these patients is fraught with challenges132 as collapse of the cyst around the shunt catheter or scarring at its tip may obstruct CSF flow.129 Balancing CSF pressures to prevent collapse and maintain flow is also difficult to determine with a shunt and its valve, leaving only trial and error and multiple surgeries as the answer to this dynamic problem.
In order to treat children with multiloculate or uniloculate hydrocephalus more effectively, pediatric neurosurgeons are turning increasingly to the use of endoscopy to reduce the need for shunts or to decrease the overall shunt burden in these patients. Endoscopic cyst fenestration aims at making sufficiently large holes (> 1 cm) in each cyst wall and having the cysts communicate with either the native ventricular drainage systems, if they are present, or with a shunt catheter.133 Cyst walls are also devascularized to help reduce the potential for closure of the stoma. The fenestrations of multiple compartments in the ventricular system are not, however, straightforward, and surgeons employ additional technology to improve fenestrations into known compartments, avoiding unnecessary manipulation of nearby neural structures. One technology that has been utilized to guide cyst fenestrations is stereotactic management, in which each cyst wall can be verified before marsupialization. Intraoperative MR imaging51,130,134 may also be used in conjunction with endoscopy to reduce the number of isolated cysts present in the CNS and to ensure maximal communication between the multiloculate locations. If the child has open fontanels, intraoperative ultrasound may also be used to verify the collapse of cysts throughout the brain.15
Before surgical intervention, preoperative MRI may improve the anatomical localization of each cyst wall in relation to the ventricular landmarks.51 The surgeon can plan multiple trajectories to determine the entry point in order optimize the number of cysts fenestrated and identify an area where these can be made to communicate with the native ventricular system or with a shunt catheter to be left in place. Entry points can also be selected to avoid eloquent cortex and neurovascular structures, which would otherwise limit the fenestration attempts. Surgical access also depends largely on the location of the cysts; those located in the anterior or body of the lateral ventricle require a precoronal bur hole, whereas those in the temporal or occipital horns may require a bur hole in the occipital region.133 After the optimal trajectory has been planned, the patient is placed under general anesthesia and prepared, and the planned incision is made. Using a combination of blunt probes, forceps, scissors, and electrocautery, the surgeon creates cyst fenestrations through the involved areas. Landmarks on the floor of the lateral ventricle are identified but may initially be obscured by cyst walls. Verifying location throughout the process with stereotaxis53 is essential to avoid collateral injury to nearby vital structures. At the end of the procedure, the ventricles are inspected for evidence of communication and for lack of hemorrhage, and if needed, a shunt catheter or ventriculostomy catheter is placed.129
Endoscopy has been shown to significantly lower the shunt revision rate in multiloculate hydrocephalus,133 and postoperative imaging may confirm the fenestrations made. Children should be followed carefully during the first several months after surgery to ensure that closure of the stomas does not occur.129 Long-term follow-up studies with MR imaging may also indicate increased growth of particular cysts and track the equilibration process. Patients with multiple cysts may be up to seven times130 more likely to need a repeated endoscopic procedure than patients who underwent endoscopic fenestration before shunt placement. Repeated endoscopic procedures for multiloculate cysts are required in up to 33% of cases; however, complex shunt systems for these patients could be simplified in 75% of patients.135 Endoscopy reduced the need for cystoperitoneal shunt revision from 2.9 revisions per year to 0.2 revisions per year.20,41
Alternative treatment strategies exist for an isolated fourth ventricle22 an aqueductoplasty with or without the use of stent assistance aims to introduce normal CSF flow through the aqueduct of Sylvius. A short segment of aqueductal stenosis is preferable119 when an aqueductoplasty is performed, and the surgical approach may originate through an anterior bur hole with access through the third ventricle. Alternatively, a suboccipital approach21 through the cerbellomedullary cistern may be used to access the foramen of Magendie.119 Stents placed in the aqueduct consist of normal ventricular shunt tubing that may be connected to subcutaneous reservoirs or existing shunt systems. Stents can be deployed with rigid, flexible, or flexible-in-rigid25 endoscope arrangements.22 Care must be taken to avoid injury to adjacent structures in the midbrain20 and to avoid prolonged occlusion of the cerebral aqueduct because it may result in bradycardia or neurologic deficits.100
Arachnoid Cysts
Arachnoid cysts account for 1% of intracranial lesions, and with improved neuroimaging techniques in children, their diagnosis has increased. Arachnoid cysts are thought to be congenital, and their etiology and pathogenesis are unclear.28,39,51,136 These intracranial lesions may result from a one-way CSF valve, osmotic gradients within the cyst, or increased fluid synthesis from the cyst wall. Fifty percent of arachnoid cysts are located in the middle fossa; 10% arise in the cerebral convexity, 10% in the midline posterior fossa, 10% in the suprasellar cistern,137 10% in the cerebellopontine angle, and 10% in the quadrigeminal cistern.138 Children may present with headaches, ataxia, seizures, or hemiparesis,139 depending upon the size and location of the cyst, and they are at increased risk for spontaneous or posttraumatic hemorrhage into the cyst or into the subdural space.140,141
The management of arachnoid cysts is controversial, and surgeons familiar with a particular approach frequently advocate endoscopy or microsurgical approaches.38,139,140,142,143 A review of arachnoid cysts treated with endoscopic techniques revealed that clinical resolution of symptoms was achieved in 90% following fenestration, and a decrease in cyst size was seen in 75% of patients. Postoperative complications were found in 16% of cases,38 with the most common middle fossa cysts having the highest recurrence and complication rates as well as the lowest clinical response rate.39,138 After fenestration (▶ Fig. 11.5), children with arachnoid cysts should be followed over several years for delayed recurrences. Similar success rates have been reported for cystocisternostomy and ventriculocystostomy28 as for ventriculocystocisternostomy, in which communication is made between the basal floor of the cyst wall and the underlying cisterns.141 Quadrigeminal cysts136 may compress the aqueduct of Sylvius and are amenable to endoscopic treatment because they are adjacent to the third ventricle. Patients with these cysts frequently present with macrocephaly, headaches, and ataxia. Fenestration options include ventriculocystostomy and ventriculocystocisternostomy, and success rates range from 70 to 90%, depending upon the series and surgical methods utilized.136
Fig. 11.5 Suprasellar arachnoid cyst. (a) Sagittal T1-weighted noncontrast magnetic resonance (MR) image of a large suprasellar arachnoid cyst with compression on the ventral pons. (b) Axial T1-weighted noncontrast MR image of the same suprasellar arachnoid cyst with compression on the cerebral peduncle. (c) Endoscopic view of the arachnoid cyst with obstruction of the foramen of Monro. The choroid plexus can be seen adjacent to the cyst along with the septal vein that runs along the left side of the image. (d) Endoscopic view of the arachnoid cyst with yttrium–aluminum–garnet (YAG) laser used to fenestrate the cyst wall.
Shunting is also a therapeutic option, with cystoperitoneal shunt placement, but it may be associated with subdural hematoma formation from a rapid decrease in cyst size. Open surgery for cysts is frequently employed, and success rates vary from 54 to 96%. Open surgery is also associated with a 7 to 12% reoperation rate.28 The advantage of using the endoscope in the treatment of arachnoid cysts is the minimally invasive approach, which avoids a larger craniotomy. Lower risk for hemorrhage and for altering the resorptive capacities of the subarachnoid space has been reported with the use of endoscopes. The success rate of neuroendoscopy in arachnoid cyst fenestration varies from 66 to 96% in the resolution of clinical symptoms and is 80% in reduction of cyst size.28 One area in which endoscopic surgery has supplanted open microsurgical technique is in the treatment of pediatric suprasellar arachnoid cysts; success rates in the reduction of cyst size have improved while the comorbidities associated with an open procedure have been limited.137 Failure rates for endoscopic cyst fenestration are similar to those of open microsurgical approaches, with rates ranging from 3 to 17%.39 The optimal treatment for symptomatic and asymptomatic arachnoid cysts remains unclear, and it is hoped that future studies will continue to clarify the use of neuroendoscopy in children with these lesions.144
Parasitic Cysts
Neurocysticercosis (NCC), resulting from infection with Taenia solium, is the most common parasitic infection in the CNS and can lead to hydrocephalus in approximately 30% of affected patients.145 NCC is estimated to cause 50,000 deaths each year in Latin America, and parasitic cysts are often seen in the lateral and fourth ventricles in children, occluding the foramen of Monro or the cerebral aqueduct.26 Noncommunicating hydrocephalus can result from the obstruction of CSF flow. Treatment for NCC infection includes antihelminthic medications such as albendazole, microsurgical removal of cysts, treatment with steroids and antiepileptic medication, ventriculoperitoneal shunting, and endoscopic retrieval of cysts.146 Endoscopy is the favored treatment modality for patients with obstructive hydrocephalus, and various endoscope setups may be utilized to retrieve parasitic cysts in the fourth ventricle. Flexible endoscopes placed in rigid endoscopes utilize the advantages of each technology, with a flexible endoscope navigating the challenging anatomy of the fourth ventricle.25 Aspiration devices in rigid scopes or flexible biopsy forceps to retrieve offending cysts are alternative setups in cases of NCC. Shunts have an estimated complication rate as high as 88%146 in NCC, and the use of neuroendoscopy avoids these comorbidities. In addition, 84% of patients whose NCC is treated with endoscopy require only one surgery to address their hydrocephalus, thereby reducing the need for additional shunt procedures.147
Intraventricular Tumors
Intraventricular tumors comprise an estimated 2% of all primary CNS tumors, and neuroendoscopy is increasingly used in biopsy for diagnosis and in resections. Intraventricular tumors in children include the following: astrocytoma, meningioma, craniopharyngioma, ependymoma, giant cell tumor, choroid plexus papilloma or carcinoma, subependymoma, germ cell tumor, teratoma, lymphoma, primitive neuroectodermal tumor, and central neurocytoma.148–150 Endoscopes can be used in a variety of ways to address intraventricular tumors; biopsies can be performed alone or in concert with ETV CSF fluid diversion151 or tumors may be resected with the endoscope, and a variety of microinstruments have been developed to work within the ports of the scope.24,55,118,152
The mainstay of neuroendoscopy in the treatment of intraventricular tumors is centered upon treating hydrocephalus, and this can be paired with biopsies153 of the lesion. The alternative to endoscopic biopsies in children is the use of stereotactic biopsy.50,154 Large series of patients undergoing stereotactic biopsy report mortality rates of 1 to 4%, morbidity of 4%, and diagnostic accuracy of 91%.154 Neuroendoscopy has lower mortality rates, variable morbidity rates ranging from 0 to 17%, and diagnostic accuracy ranging from 70 to 100%, depending upon the series.23,24,55,118,152,155 Rigid endoscopes are correlated with higher diagnostic rates than are flexible endoscopes.73,118,156 Endoscopic series of tumor biopsies may have higher morbidity rates because there is a larger opening affecting nearby neural structures, including the fornices, and because it is difficult to control the most common complications of CSF leakage and intraventricular hemorrhage. The advantage of endoscopy is direct visualization of the tumor in relation to other adjacent structures, allowing a full inspection of the anatomy before biopsy, whereas a stereotactic approach is essentially a blinded procedure guided by computer representations of the anatomy. Endoscopic biopsy has the added benefit of coupling tissue sampling with concurrent CSF diversion or tumor debulking.157,158 Recent studies on the administration of fluorescent dye to visualize tumors and improve the results of diagnostic biopsy are promising.159
Frameless stereotactic systems can be combined with microsurgical and endoscope-assisted resections153 of intraventricular lesions to improve localization and the amount of tumor resected.160 Intraventricular tumors are frequently approached through an ipsilateral bur hole, with the tumor accessed through a dilated lateral ventricle.155,161 With technical innovations in surgical instrumentation, devices can now aspirate and evacuate tumor. Oscillating microdebriders,162 ultrasonic aspirators, and variable-aspiration resection devices can be deployed through the working channel to improve tumor manipulation and extirpation. The improvements in these devices continue to advance the surgeon’s ability to remove tumors through the minimally invasive approach. Alternatively, endoscopy following the microsurgical resection of an intraventricular tumor allows a surgeon to inspect the resection bed for residual tumor. The variably angled lenses161 of the endoscope allow a wider field of view than that of an operating microscope and make it possible for the surgeon to confirm that a gross total resection has been achieved by peering around corners.31
Proponents of using the endoscope for the gross total resection of lesions have advised that lesions with moderate to low vascularity, soft consistency, size of less than 2 to 3 cm, low histologic grade, and location in the lateral ventricle are most amenable to resection via an endoscope.163 The absence of hydrocephalus is not an absolute limitation to resection or an endoscopic approach to intraventricular lesions.70 Hence, decisions to attempt gross total resection of an intraventricular tumor must take into account the lesion‘s histology, size, location, and degree of vascularity. Outcome results for patients who underwent endoscopically assisted resection of pineal lesions have revealed shorter hospital stays and shorter surgical times, suggesting a potential advantage and future role for endoscopy in treating pediatric intraventricular lesions.164
The most common locations for intraventricular tumor biopsies are lesions of the lateral and third ventricles as well as lesions surrounding the foramen of Monro.27,37,44,149,165,166 Exophytic tumors arising from the thalamus, mesencephalon, or basal ganglia may also warrant biopsy sampling; however, caution should be exercised because hemorrhagic complications during surgery may prompt conversion to an unplanned open procedure.155,167–169 Biopsies for intraventricular lesions are particularly useful when both CSF and tissue are needed for histologic analysis to exclude pathologies that may be amenable to radiotherapy or chemotherapy. CSF tumor markers for alpha fetoprotein and human chorionic gonadotropin may also be obtained.23,24 Endoscopic diagnosis of tumors like germinomas and lymphomas can alter the treatment course of children and ultimately lower the morbidity and mortality related to the surgical intervention.158
Pineal Region Tumors
Pineal region tumors are one type of pediatric CNS tumor for which endoscopic biopsy is routinely used in tissue diagnosis.30,155,169 Endoscopic approaches to pineal tumors have greatly enhanced the minimally invasive options for treating children with these lesions. Diagnostic biopsies with the endoscope are increasingly utilized to determine if chemotherapy, radiation, and microsurgery are warranted for a particular child’s anticipated clinical course.158 Pineal tumors have an incidence of 3% in the general population and comprise 11% of all pediatric tumors.118 Germ cell tumors are the most common pineal tumors, comprising 50 to 80% of lesions, and more than 50% of germ cell tumors are found to be germinomas. Pineal parenchymal lesions, including pineocytoma161 and pineoblastoma, occur in 7 to 14% of patients, and neuroepithelial lesions, such as gliomas, are the next most common diagnosis, occurring in 2 to 8% of patients. In children, 50 to 60% of tumors diagnosed with pineal tumor biopsy were found to be sensitive to chemotherapy or radiation therapy.55
Diagnosis with this minimally invasive approach avoids the permanent morbidity of 10% associated with the open microsurgical resection of pineal lesions and the estimated 2% complication rate reported with stereotactic biopsy. In a review of the literature, endoscopy yielded a definitive diagnosis in 89% of cases, a number that may depend upon expertise among endoscopists.55,118 Neuroendoscopy for pineal region tumors also allows ETV for CSF diversion in the same surgical setting because 90% of patients with a pineal tumor present with hydrocephalus.155 The ETV and biopsy procedure may be performed through a single bur hole, splitting the distance between a precoronal bur hole,153 the site commonly used for an ETV, and a bur hole at the hairline, the site commonly used for pineal region biopsy.170 A single bur hole is optimal when the lesion is anterior to a small massa intermedia and when the ventricles are dilated.
Colloid Cysts
Colloid cysts can sometimes be managed endoscopically. Colloid cysts are benign intracranial lesions and account for 1% of all intracranial neoplasms.47 They can present with symptoms and neurologic deficits consistent with elevated ICP, and sudden death in patients, presumably from acute hydrocephalus and obstruction of the foramen of Monro, has been reported. With the increasing use of cranial MR imaging, colloid cysts are found incidentally, leaving an ongoing discussion about the parameters that necessitate operative intervention.34 Traditionally, these lesions have been resected with microsurgical techniques through a transcortical and transventricular approach or a transcallosal approach.62 The authors prefer a bur hole that is more anterior and lateral than the midpupillary line in the endoscopic treatment of colloid cysts because this allows optimal visualization of the colloid cyst. A more medial approach may limit the resection or obscure portions of the cyst wall. Endoscopic resections have emerged as a minimally invasive treatment option for these lesions (▶ Fig. 11.6a,b). Endoscopic approaches are advocated because of the proposed decreases in risk of postoperative seizures from cortical manipulation, in operative time, and in hospital stay.26 Open mircosurgical techniques are associated with a 5% risk of seizures, whereas endoscopically guided colloid cyst removal has approximately a 1% risk for postoperative seizures. One of the most common complications of endoscopic resection that has been reported is impaired memory in 5% of cases171 and is attributable to manipulation of the adjacent fornices, prompting a critique as to whether the cyst capsule should be completely removed.172 Studies have also been performed on the imaging characteristics of colloid cysts and whether they dictate surgical management decisions, with hypointensity on T2-weighted MR images predicting recurrence of endoscopically resected lesions.171
Fig. 11.6 Colloid cyst resection. (a) Axial T2-weighted magnetic resonance (MR) image of a colloid cyst with obstruction at the foramen of Monro and resulting ventriculomegaly in the lateral ventricles. (b) Axial T2-weighted MR image following endoscopic resection of the colloid cyst with a patent foramen of Monro and resolution of the ventriculomegaly.
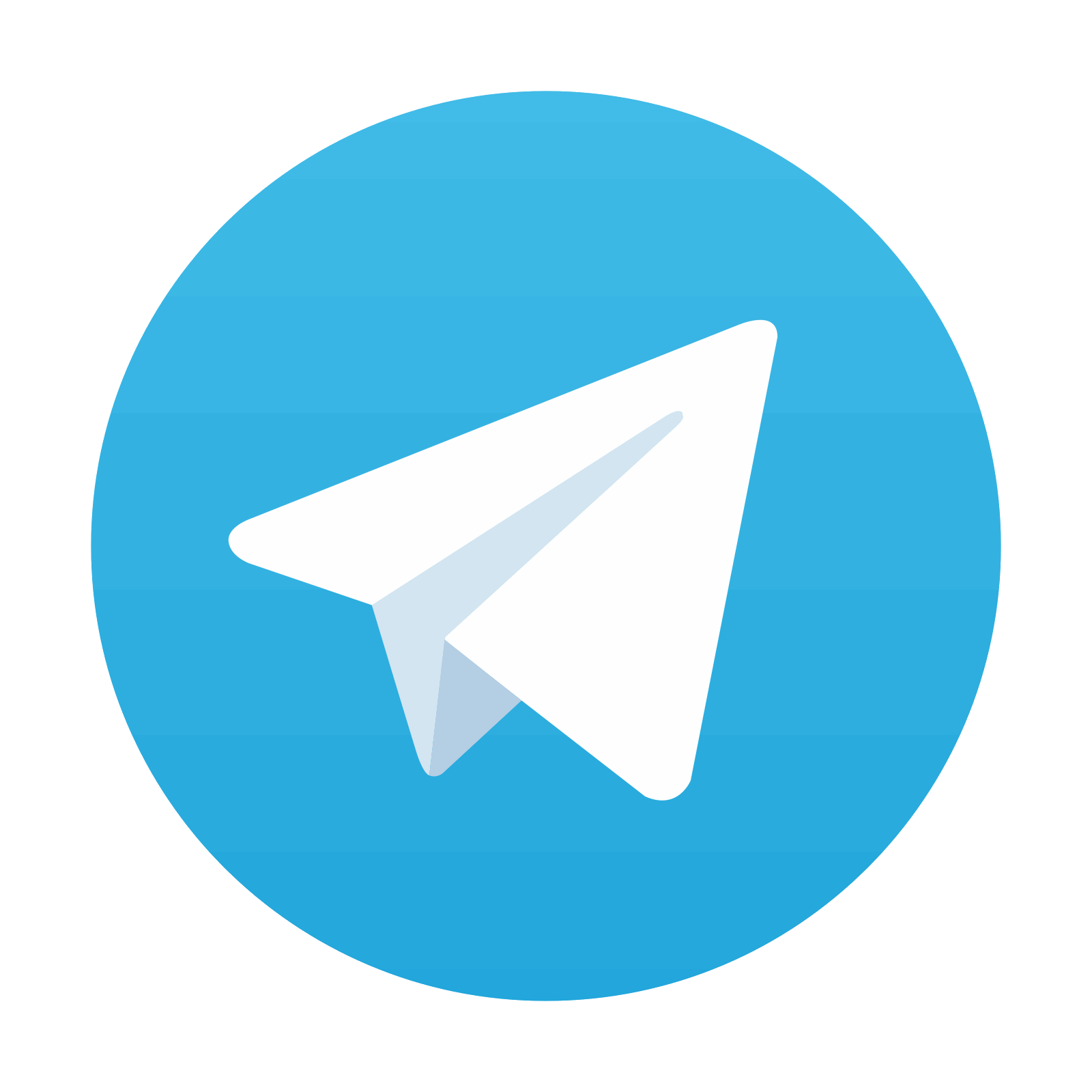