CHAPTER 118 NEUROLOGY OF ENDOCRINOLOGY
CLASSIFICATION OF HORMONES
Multicellular organisms have developed complex mechanisms to ensure regulation of metabolism. Although the nervous system and endocrine system were classically considered to play crucial and largely independent roles in this process, studies over several decades demonstrated that these systems converge to maintain homeostasis and various physiological functions. In its simplest form, the endocrine system may be viewed as consisting of an endocrine gland: that is, a collection of specialized cells that synthesize and secrete a hormone and a target tissue that responds to this hormone. Hormones are chemical substances, produced by endocrine organs or cells dispersed in major organ systems, that act at distant tissues through the blood to exert their biological actions.
Steroid hormones are derived from enzymatic processing of a cholesterol precursor (Fig. 118-1). Conversion of cholesterol to pregnenolone through side-chain cleavage is the first step in the steroidogenic pathway and occurs in all steroidogenic tissues (i.e., adrenal cortex, testes, ovaries, and placenta). Further metabolism is determined by specific enzymes that mediate hydroxylation, methylation, and demethylation. Corticosteroids and progestins contain 21 carbons; androgens, 19 carbons; and estrogens, 18 carbons and aromatic A-ring. Because 11- and 21-hydroxylases are expressed only in the adrenal cortex, the production of glucocorticoids and mineralocortiocoids occurs only in this gland. Cortisol, the principal glucocorticoid, is synthesized mostly by zona fasciculata cells and hydroxylated at the 17-carbon position and exerts major effects on glucose and various metabolic processes. Aldosterone, the principal mineralocorticoid, is a 17-deoxycorticosteroid produced in the zona glomerulosa of the adrenal cortex. Progesterone is the main steroid secreted by the placenta and ovaries and also serves as a precursor for corticosteroids and other sex steroid hormones.
MECHANISMS OF HORMONE ACTION
Hormones by definition are transported in the blood, although the methods for transporting peptides, steroids, and catecholamines vary, depending on solubility. Peptide and protein hormones are hydrophilic and dissolve directly or associate with albumin in the plasma. Insulin circulates as monomeric and polymeric forms, whereas some anterior pituitary hormones circulate as dissociated subunits. Steroid and thyroid hormones, in contrast, are insoluble and bind to transport proteins (corticosteroid-binding globulin in the case of cortisol and thyroid-binding globulin and transthyretin in the case of thyroxine). The hormone bound to the carrier protein cannot interact with the receptor, but it serves as a reserve to replenish the free (bioactive) form. Nonetheless, the bound hormone exists in equilibrium with free hormone and receptor-bound fractions. The free hormone level increases as the rate of hormone degradation and clearance rises. On the other hand, conditions that increase the amount of carrier proteins, such as pregnancy and liver disease, elevate total hormone levels, although the balance between free and bound hormone is preserved.
In view of the fact that hormone concentrations are very low, in the range of 10−15 to 10−9 M, and more than 100-fold lower than levels of similar sterols, amino acids, and polypeptides, how do target cells identify hormones to initiate specific biological effects? In general, receptors for peptide, amine, and steroid hormones have a domain that recognizes and binds the hormone, with subsequent activation of a signaling mechanism that transduces the information into an intracellular action. Steroid and thyroid hormones and retinoic acid are lipophilic; associate with transport proteins in the blood, which prolongs their plasma half-life; and readily cross the plasma membrane in target tissues and bind to receptors in the nucleus or cytoplasm (Table 118-1; Fig. 118-2).1,2 The hormone-receptor complex undergoes activation, which leads to changes in chromatin, binding to specific regions of DNA, and transcription or inactivation of target genes. Studies since the 1980s have led to greater understanding of steroid-regulated genes, hormone response elements (i.e., short DNA segments that bind to specific steroid receptor-hormone complexes), and how these cis-acting DNA elements interact with transacting factors.3–5 Transacting factors include coactivator and corepressor molecules that modulate transcription.5 For example, in the absence of hormone, thyroid and retinoic acid receptors associate with NcoR, SMRT and other proteins, which results in repression of target genes. Binding by thyroxine dissociates the complex culminating in gene activation. Members of the p160 family of coactivators (e.g., SRC-1, NCoA-1, GRIP 1, TIF2, and p/CIP) have been implicated in the function of corticosteroids and other steroids.
TABLE 118-1 Classification of Hormone Receptors
Steroid Family | Seven-Transmembrane Domain | Single Transmembrane |
---|---|---|
ACTH, adrenocorticotrophic hormone; FSH, follicle-stimulating hormone; LH, luteinizing hormone; PPAR, peroxisome proliferator-activated receptor; TRH, thyrotropin-releasing hormone; TSH, thyroid-stimulating hormone.
Polypeptides, glycoprotein hormones, and catecholamines are water soluble, have no transport proteins, and possess a relatively short half-life. These hormones bind to surface receptors on the plasma membrane and generate second-messenger molecules (Fig. 118-3). Epinephrine, as well as several neuropeptides and gut and pituitary hormones, including neuropeptide Y, cholecystokinin, vasopressin, glucagon, ACTH, thyroid-stimulating hormone (TSH), and luteinizing hormone, bind to their respective membrane receptors, stimulate adenylate cyclase located on the inner plasma membrane, and catalyze the formation of cyclic adenosine monophosphate (cAMP) from adenosine triphosphate.6 Activation or inactivation of adenylate cyclase occurs through the guanosine triphosphate–dependent regulator proteins (e.g. Gs [stimulatory] and Gi [inhibitory]).7 Changes in cAMP exert diverse effects on metabolism through various substrates, such as the transcription factor cAMP response element binding protein (CREB). CREB phosphorylation by cAMP leads to interaction with the coactivator CREB-binding protein, which results in stimulation of gene transcription.8 These effects can be terminated by hydrolysis of cAMP by phosphodiesterases or dephosphorylation by phosphoprotein phosphatases.9
Some polypeptide hormones produced by vascular tissues, such as atrial natriuretic factor, stimulate guanosine triphosphate by guanylate cyclase and increase cyclic guanosine monophosphate (cGMP), which leads to activation of cGMP-dependent protein kinase, phosphorylation, and alteration in the of smooth muscle proteins.10 This effect is terminated by specific cGMP phosphodiesterase. Other hormones signal through phosphatidylinositides and calcium. The actions of ACTH in the adrenal cortex, luteinizing hormone in the ovaries and Leydig cells of the testes, and angiotensin II in vascular tissues have been associated with activation of phospholipase C, which mediates the hydrolysis of phosphatidylinositol-4,5-biphosphate (PIP2) to 1,4,5-triphosphate (PIP3) and diacylglycerol. Binding of PIP3 to organelles increases intracellular Ca2+.9 Diacylglycerol activates protein kinase C, which phosphorylates various substrates involved in metabolism.
Cytokine receptors lack intrinsic tyrosine kinase activity but associate with proteins that are tyrosine kinases.11,12 For example, growth hormone, prolactin, inflammatory cytokines, and leptin bind to their receptors, which activate cytoplasmic protein tyrosine kinases, such as Jak1 and Jak2.11–13 These then phosphorylate other proteins, such as signal transducers and activators of transcription (STAT) proteins and docking proteins containing Src homology 2 (SH2) domains, culminating in transcriptional activation of neuropeptides and various target genes. In contrast, the insulin receptor possesses intrinsic tyrosine kinase activity in the cytoplasmic domain that is activated upon binding of insulin to the extracellular domain of the receptor. This autophosphorylation of insulin receptor leads to phosphorylation of insulin receptor substrates, activation of PI-3 kinase, and a cascade of biochemical events underlying insulin’s effects on glucose uptake, lipid and protein metabolism, and growth.14
REGULATION OF HORMONE LEVELS AND RHYTHMS
Hormones generally regulate existing reactions instead of initiating de novo reactions. In contrast to the rapid time course of nervous activity, which lasts from milliseconds to seconds, the action of hormones is often prolonged and may persist for some time after the hormone is withdrawn. However, as discussed in detail later, the neurosecretory system allows the neural pathways in the hypothalamus, brainstem, and other regions of the central nervous system to interact with peripheral endocrine tissues, such as the thyroid, adrenal gland, and gonads.
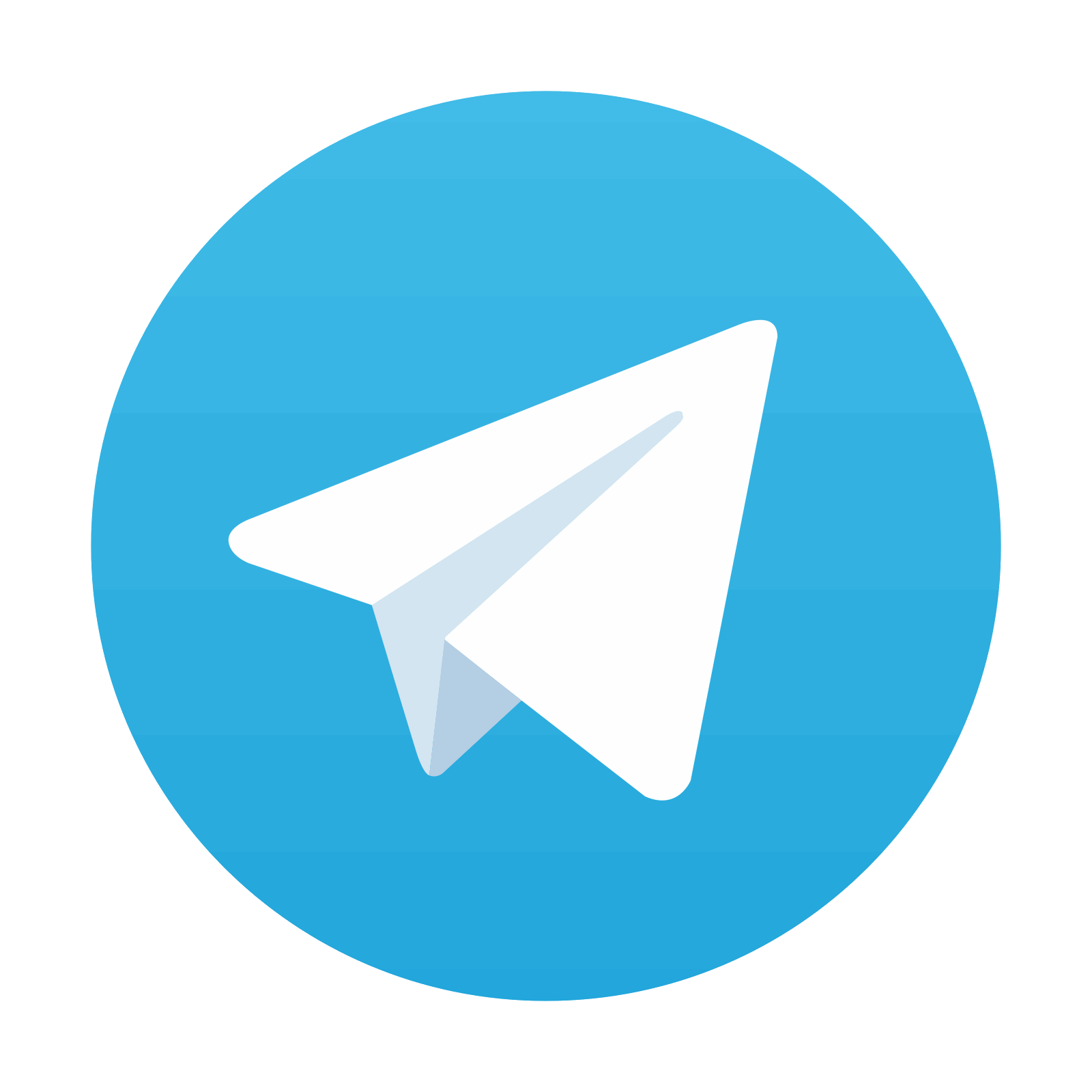