Ontogeny of the Reticular Formation: Its Possible Relation to the Myoclonic Epilepsies
Harvey B. Sarnat
Alberta Children’s Hospital, Pediatrics (Neurology) and Pathology (Neuropathy), Calgary, Alberta, Canada
Introduction
The reticular formation (RF) is a central core of polysynaptic ascending and descending circuits of interneurons, extending caudally from the thalamus and through the periaqueductal grey matter of the midbrain and the tegmentum of the pons and medulla oblongata to the spinal cord (1,2). The initial connection of the reticular formation with the forebrain is at the marginal zone of the early telencephalon and includes the Cajal–Retzius neurons that form a preplate plexus before the arrival of the first wave of radial migrations of neuroblasts from the subventricular zone. Its influence on spinal cord function is through the reticulospinal pathway and, as with other bulbospinal tracts, axons decussate before or during their longitudinal descent. The fundamental cell of the reticular formation is the interneuron. No primary afferent sensory of efferent motor neurons to or from the reticular formation exit from the central nervous system to project into the periphery.
From an anatomical viewpoint, the RF is unlike any other region of the central nervous system (CNS). It is difficult to visualize in sections of brainstem because it forms discrete anatomic nuclei with histologically defined margins only in some portions. Most of the reticular formation is a diffuse irregular longitudinal column of structures with indistinct boundaries filling the space between more distinctive nuclei of the brainstem. Both magnocellular and parvocellular regions are identified. Many of these regions at different levels of the brainstem have been assigned arbitrary names, based on position and size of the neurons, such as the nucleus paramedianus and the nucleus gigantocellularis dorsalis. In some places, such as the thalamus and midbrain, the bilaterality of the RF is obvious; but even in other regions where it may appear continuous across the midline, it remains a bilateral, symmetrical structure. The descending reticulospinal tracts decussate, as with other bulbospinal axonal projections. Despite its multisynaptic nature, in which neurons have only short axons, the synaptic organization of the RF is not random or unpredictable; rather, it is as precise as in any other part of the brain (3). The number of neuroanatomical and neuroembryological studies on the reticular formation is small, reflecting the difficulty in studying such a heterogeneous and often apparently nebulous anatomic structure. The structures included in the reticular formation vary according to different authors. Many include the locus ceruleus, median raphé nuclei, substantia nigra, and structures adjacent to more defined brainstem nuclei, such as the nucleus and fasciculus solitarius and nucleus ambiguus (3); the latter should be excluded, however, because it contains primary motor neurons. Some authors include portions of the limbic system, including the amygdala and hippocampal formation, as components of the ascending reticular formation. Reciprocal fiber connections with the majority of brainstem structures make it a very complex system and the neurotransmitters synthesized and secreted by reticular formation neurons are multiple and include serotonin, monoamines including dopamine and norepinephrine, γ-aminobutyric acid (GABA), and a variety of neuropeptides. This diversity underscores that the reticular formation is really a heterogeneous group and not a single, unitary entity.
Consistent with the diversity of anatomical structure and neurochemical projections, the functions of the reticular formation are diverse and include coordination of movements of the head and body, alternation of respiration and blood pressure, a gate to block certain sensory inputs such as pain, arousal and attention, sleep states, and influence on emotion in the limbic system (3).
Because of the implication of the RF as a possible site of origin of subcortical epilepsies, and the myoclonic epilepsies of infancy and early childhood, in particular, its ontogenetic, and indeed its phylogenetic, development should be considered in the context of the evolution of subcortical epilepsy, as well as in the context of alterations that the reticular formation may undergo in various cerebral malformations.
What is an Interneuron?
The essence of the RF is the interneuron, generally one with a long axon and many collateral branches along its length as it descends in the brainstem. An interneuron is a neuron in which afferent and efferent connections remain entirely within the central nervous system and do not project dendrites or axons peripherally. The predominance of interneurons is one of the principal differences distinguishing a ganglion and a brain (Table 2-1) (4). Even the so-called “cephalic ganglion” of very simple invertebrates, such as the flatworm, is a true brain. There is no evidence that, in the embryonic development of humans or any other animals, the initial rostral neural folds of neuroepithelium are ever a cerebral ganglion that later becomes a brain. The interneuron, therefore, is primordial in the development of a CNS in both phylogeny and ontogeny; the reticular formation is one of the fundamental and earliest structures to develop.
Table 2-1. Comparison of Brain and Gangliona | ||||||||||||||||||
---|---|---|---|---|---|---|---|---|---|---|---|---|---|---|---|---|---|---|
|
The definition of the interneuron has itself evolved because of the integration of the neurophysiologic with the initial morphologic criteria. This more modern definition began with the concept that interneurons were inhibitory cells with short axons that regulated excitability in local circuits, by contrast with excitatory principal cells with long axons projecting information to distant regions within the CNS. However, this oversimplification must be further modified, because some reticulospinal axons are long and some nonreticular long pathways within the CNS are mainly inhibitory (e.g., corticospinal tract and corpus callosum) whereas other interneurons with short axons are excitatory (e.g., cholinergic interneurons of the corpus striatum) (5). Most, but not all, interneurons are indeed inhibitory, synthesizing and secreting GABA as their neurotransmitter, but exceptions to this rule also are documented (e.g., the glycenergic inhibitory Renshaw cells of the ventral horn of the spinal cord) (6). Nearly all interneurons of the hippocampus and cerebral neocortex release GABA (5,6,7). These interneurons are electrically coupled with paravalbumen-containing basket cells that are essential for gamma and theta oscillations physiologically, and a highly modifiable syncytium of cholecystokinin-containing interneurons in the neocortex carries impulses from subcortical pathways, related to emotion and motivation; impairment of this inhibitory mechanism may result in disorders of mood, such as anxiety (8). It is evident that there is a much greater diversity in the morphology and connectivity of local circuit interneurons in the mammalian brain than of the primary neurons, including the decussating interneurons with long axons, such as the pyramidal cells of the cerebral cortex, which are more uniform and have much less plasticity (5). Synaptic plasticity, similar to that observed in primary pyramidal neurons, and the presence or absence of long-term potentiation in interneurons are important determinants of their function (7).
Phylogeny of the Reticular Formation
We may infer much from the study of comparative neuroanatomy that is relevant to understanding the relations of different structures of the central nervous system. Those structures that differ substantially in each class and species denote the evolution of the nervous system. The forebrain of reptiles and birds, compared with that of mammals, is a good example. Structures identified in all vertebrates and that have changed little except for somewhat more elaborate development in more complex brains tell not about evolution, but rather about the origin of the nervous system. The ventricular system is an example, the reticular formation is another good example.
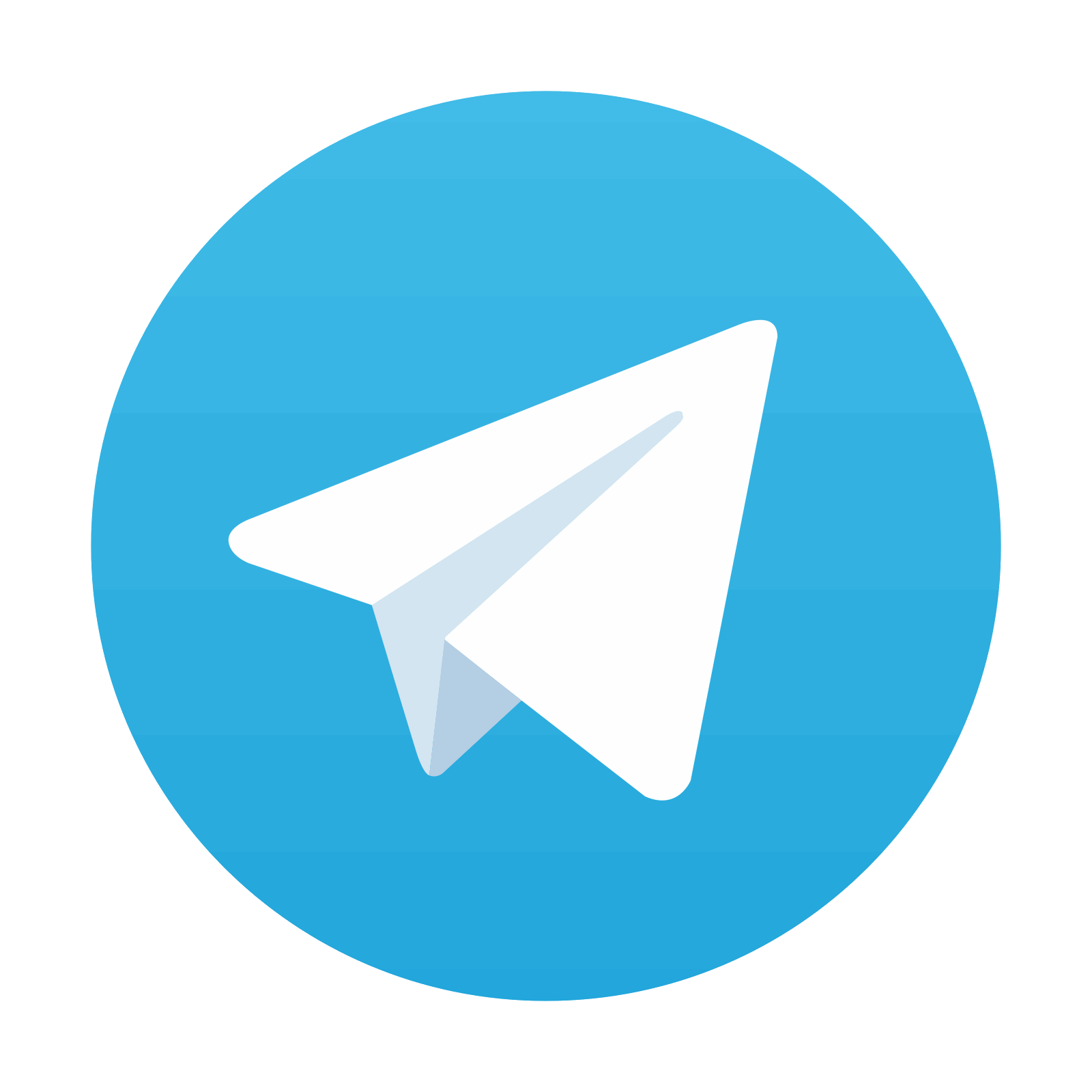
Stay updated, free articles. Join our Telegram channel

Full access? Get Clinical Tree
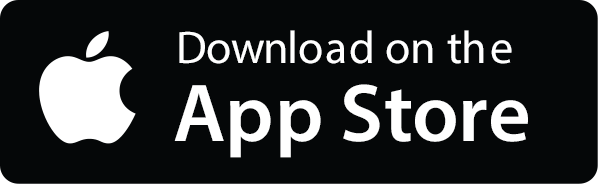
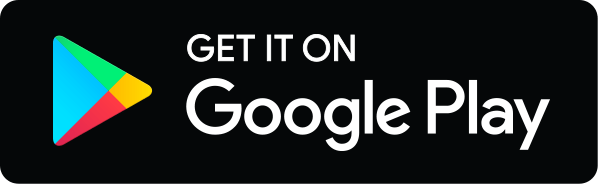