Open Fetal Surgery for Myelomeningocele
Fetal surgery arose in an effort to improve the outcome of patients with specific developmental anomalies, such as congenital diaphragmatic hernia and bladder outlet obstruction, that were often fatal, either in utero or immediately after delivery.1 Because the pathologic effects of these disorders appeared to worsen during gestation, pediatric surgeons began to realize that early intervention, preceding irreversible physiologic sequelae, could improve survival, and perhaps functional outcomes. The technical steps that were required to achieve the goal of successful fetal surgery with acceptable maternal morbidity took decades to develop, initially in a nonhuman primate model.2,3
The primary difference between fetuses with open neural tube defects (NTDs) and those with other diseases is that the anomaly is not fatal if untreated, gestation is usually carried to full term, and NTDs are not usually associated with other major organ defects.4 This is an ethical challenge for treating physicians because the potential benefits of prenatal intervention must be balanced by well-defined risks to the fetus and the mother, who is a “bystander” with respect to the perceived benefits—that is, she undergoes significant surgical risk but accrues no direct medical benefit. The ethical considerations of fetal surgery are most analogous to living related organ transplantation.
Fetal surgery requires the active participation and interaction of several clinical teams. Each group has a specific role, with overlap often required at different points of the evaluation and treatment plan. A multidisciplinary approach is mandatory in order to accomplish the surgical goals with acceptable clinical outcomes. Regular case discussions allow a joint review of prospective patients and their preoperative considerations, as well as ongoing follow-up of postoperative patients. Extensive counseling with the patient and family is critical before the patient can be expected to reach a carefully considered decision and before informed consent can be obtained. The risks and benefits of fetal surgery, as demonstrated by the recently published results of the Management of Myelomeningocele Study (MOMS), are described later in this chapter.5
22.1 Rationale for Fetal Repair
Although myelomeningocele is a defect of central nervous system (CNS) development, the impact upon other organ systems contributes to a substantial burden of disease.6 Despite aggressive postnatal intervention, nearly 14% of children with NTDs do not survive past 5 years of age, and the mortality rises to 35% among those with symptoms of brainstem dysfunction secondary to the Chiari 2 malformation.7 Although 70% of affected individuals have an IQ above 80, only 37% are able to live independently as adults.8
Although there is a broad spectrum of severity, it is useful to separate the neurologic deficits into two groups: primary and secondary. The primary neurologic deficits are those directly caused by the arrested development of the neural placode, which usually occurs in the lumbosacral region.9,10 Because neural tube closure occurs during the third and fourth weeks of gestation, the spinal cord in this region is very immature at the stage when a myelomeningocele develops. Although the structure of the spinal cord is severely disrupted at the involved level, it is unknown whether the placode is capable of further development.11 The functional neurologic level is either at the same level as the vertebral anomaly or actually higher, resulting in worse neurologic function in more than 80% of patients with open NTDs.12
The secondary neurologic deficits in patients with spina bifida include delayed loss of motor function, worsening bowel and bladder control, and scoliosis. Magnetic resonance (MR) imaging studies of most myelomeningocele lesions following repair show a dysplastic spinal cord terminating in the overlying soft tissues at the site of the repaired defect. Because the normal dura and other dorsal tissues are absent, the surgically separated placode is almost always attached to the overlying by scar tissue. At least by radiologic and anatomical features, virtually all patients with a true myelomeningocele have a tethered spinal cord. It is unclear what factors lead to the development of new symptoms attributable to the tethered spinal cord in some patients and not in others. Another source of confusion is that the symptoms and signs typically attributed to a tethered spinal cord can be caused by other conditions, such as a Chiari 2 malformation, a spinal cord syrinx, or hydrocephalus.
22.2 Preclinical Research
Fetal surgery for a variety of conditions was systematically developed in a series of consistent steps, beginning with proof of concept in an animal model, definition of the natural history in utero, refinement of surgical techniques in test cases, and finally evaluation of efficacy in a prospective randomized clinical trial.
The theoretical advantage of fetal repair for myelomeningocele is that the neural tube is covered and protected for many months before the expected delivery date. The basis for anticipating improved neurologic function is that restoration of the dysplastic neural placode within the spinal canal isolates it from the amniotic fluid and prevents ongoing injury.13,14 An ideal animal model that would occur as a spontaneous lesion and allow surgical access with measurement of outcome does not exist. In order to test fetal intervention, Meuli et al surgically created a spinal cord lesion in fetal sheep at 75 days of gestation that simulated a spina bifida lesion.3,15,16 A laminectomy was performed to expose the fetal spinal cord at 75 days of gestation, and the pregnancy was allowed to continue. The lambs were delivered by cesarean section at 140 days of gestation. After delivery at term, the gross and microscopic appearance of the exposed spinal cord resembled a human spina bifida lesion, and the animals were incontinent and had loss of sensation and motor function below the lesion level. One group of animals with surgically created spina bifida lesions were then treated with a myocutaneous flap at 100 days of gestation. These animals were then carried to full term and had nearly normal motor function and normal bowel and bladder control. The results of these experiments suggest that early repair of an exposed spinal cord may preserve neurologic function and may allow improvement through plasticity. Additionally, in a separate set of animal experiments, hindbrain herniation mimicking the Chiari malformation was created by creating a cerebrospinal fluid (CSF) leak in the spinal canal; hindbrain herniation was reversed by fetal repair in the lambs.17 Although provocative, these large animal experiments clearly rely on a model system that has distinct differences from the human disease.
22.3 Initial Fetal Surgery for Myelomeningocele
The first cases of fetal myelomeningocele repair were performed in 1994 with an endoscopic technique,18 followed by an open hysterotomy approach.19,20 These early experiences were encouraging in that some of the secondary neurologic features associated with myelomeningocele, such as hindbrain herniation and hydrocephalus, were potentially reversible.21–24 In the combined series of patients undergoing fetal surgery from The Children’s Hospital of Philadelphia, and Vanderbilt University (Nashville, TN), 104 patients followed for at least 1 year had an overall incidence of shunting of 54%, compared with 86% in a historical control group from The Children’s Hospital of Philadelphia.25 The effect was most evident for those with lumbar lesions. The incidence of shunting in those patients who underwent fetal closure before 26 weeks of gestation was 42.7% but was 75% in those who had fetal surgery after 25 weeks of gestation. Although speculative, a potential mechanism explaining this improvement is that chronic CSF leakage and a reduction in subarachnoid pressure during fetal development lead to both hindbrain herniation and eventual permanent failure of CSF absorption. Interpretation of the results of these early case series was limited by selection bias and the lack of true control arm.
22.4 Management of Myelomeningocele Study
22.4.1 Study Design
Prospective randomized surgical clinical trials are difficult to conduct for a variety of reasons. The complexity, controversy, cost, and risks of fetal surgery, however, provided a stronger rationalization for examining these novel techniques in rigorous clinical trials. Other conditions treated by fetal surgery, such as congenital diaphramatic hernia and twin–twin transfusion, have been successfully studied with a randomized clinical trial design. Therefore, once the feasibility of fetal repair for myelomeningocele and a possibility of benefit had been demonstrated in pilot studies,22,26–28 the logical next step was to conduct a randomized trial to determine if a real benefit was present.
The design of MOMS required an initial screening by a nonsurgical coordinating center (George Washington University, Washington, DC), and if patients met the inclusion criteria, they traveled to one of three surgical institutions—The Children’s Hospital of Philadelphia, Vanderbilt University, or the University of California at San Francisco. Additional evaluation was performed at the surgical sites, and if consent was provided, the patient was randomized into either a postnatal or a prenatal treatment arm. Mothers sometimes stayed for many weeks at the study site, depending upon the development of any complications.
An unusual feature of the trial was the development of two primary clinical outcomes. First was the need for a ventricular decompressive procedure, usually a ventriculoperitoneal (VP) shunt, for the treatment of hydrocephalus. The second primary outcome consisted of a composite score derived from the Bayley Scales of Infant Development and the difference between motor function and lesion level. A great deal of planning was done to minimize the impact of bias. Because there are recognized variations in practice across the country, simply receiving a VP shunt was not a satisfactory outcome measure for evaluating hydrocephalus. Therefore, objective shunt criteria were created, and an outside panel of pediatric neurosurgeons, blinded to type of repair, were assigned to determine whether the subjects met those shunt criteria. For neurologic outcomes, the subjects were examined in a blinded fashion by an independent group of specialists not associated with any of the surgical sites.
22.4.2 Results
MOMS was halted after 183 subjects, of the planned 200, had been recruited. An interim analysis of 158 subjects who had reached 12 months of age and of 138 who had reached 30 months of age demonstrated efficacy. The two treatment groups did not differ in terms of maternal age, body mass index, or gestational age at the time of surgery. The lesion level was higher in the fetal treatment group, with 33% having a lesion at L2 or higher, compared with 17% in the postnatal group. In terms of the treatment of hydrocephalus, by objective criteria, 65% of the subjects in the fetal group met previously determined shunt criteria, compared with 92% of the postnatal group (a significant difference; p < 0.001). Of note, the treating neurosurgeons at the family’s community were not blinded to the treatment that patient had received, and in the fetal group, only 40% actually received a shunt, compared with 82% in the postnatal group.
The difference in the second primary outcome, determined at 30 months after birth, also reached statistical significance (p < 0.007), with the difference between motor function and lesion level higher than expected in the fetal treatment group. The difference in the other component of this outcome measure, the Bayley Scales, was not statistically significant. The most striking difference was the presence of hindbrain herniation, with 76% of the fetal group having none or a mildly severe form and 67% of the postnatal group having a moderate or severe form (▶ Fig. 22.1). Finally, twice as many of the fetal group (42%) were ambulating at 30 months than of the postnatal group (21%).
Fig. 22.1 Effect of fetal closure on hindbrain appearance. (a) Fetal sagittal magnetic resonance (MR) image showing a tight posterior fossa with tonsillar descent into the upper cervical spine. (b) MR imaging (T1-weighted sagittal sequence) after delivery showing near-normal appearance of the cerebellum with normal-appearing brainstem morphology and the presence of a cisterna magna.
(Reproduced with permission of the publisher from Jallo G, Kothbauer KF, Pradilla G, eds. Controversies in Pediatric Neurosurgery. New York, NY: Thieme Medical Publishers; 2010:chap 16.)
The maternal risks in the fetal group were notable: chorioamniotic separation (26%), oligohydramnios (21%), need for blood transfusion at delivery (9%), placental abruption (6%), and pulmonary edema (6%). Although there were 2 fetal or neonatal deaths in each group, fully 80% of the fetal group were delivered prematurely (before 36 weeks of gestational age), compared with 15% in the postnatal group.
22.4.3 Interpretation of Results
MOMS required nearly 10 years to complete with the active participation of several large clinical and research teams. Despite its complexity, the results obtained are relatively clear. The fetal treatment group, despite having a higher lesion level, had better clinical outcomes in terms of hydrocephalus, Chiari malformation, and ambulation. Several caveats must be recognized. First, the outcomes were measured at 12 and 30 months. It is possible that these benefits are not durable and will not persist throughout life. Second, although the differences in the anatomical appearance of the hindbrain herniation and in the reduction of treated hydrocephalus appear real, whether these will result in a meaningful difference in outcome and reduction in morbidity is unknown. A long-term follow-up study of the two treatment groups from MOMS is under way, and it is hoped that it will provide some of the answers to these questions. The final important point is that the risks of the procedure are defined. These include prematurity, maternal complications, and the impact on future pregnancies. They are not to be underemphasized when fetal surgery is being considered.
22.5 Planning and Conduct of Fetal Surgery for Myelomeningocele
22.5.1 Initial Evaluation
From a theoretical perspective, fetal repair should be performed as early as possible. In practice, surgical timing is determined by the existing standards of prenatal care in normal pregnancies. Most myelomeningoceles are detected during the second trimester, either during an investigation of a positive maternal serum screening test (i.e., elevated maternal serum level of alpha fetoprotein) or during a routinely scheduled fetal anatomical ultrasound survey (usually scheduled at or after 18 weeks of gestation). The quality of current ultrasonography allows the detection of most fetuses with myelomeningoceles by the middle of the second trimester.29 From a practical viewpoint, this means that a diagnosis is most often made between 18 and 22 weeks of gestation. It is unlikely that the detection of fetuses affected with spina bifida will occur any earlier unless more sensitive screening tests become widely used.
22.5.2 Preoperative Screening
Patients referred to a fetal surgery center should undergo a preliminary screening evaluation to confirm if an in-person evaluation is appropriate. An evaluation of the mother’s risk as a surgical candidate requires recorded information of all physical examinations and the medical, surgical, and anesthetic history. A normal fetal karyotype is required if a patient is to be considered a potential candidate for prenatal fetal surgery. Before any decision is made by the patient and surgical team that leads to fetal surgery, a detailed evaluation at the fetal surgery center is required. This includes but is not restricted to:
Comprehensive obstetric ultrasonographic examination, including documentation of the cervical length, placental location, fetal gestational age, degree of fetal kyphosis, level of the myelomeningocele, lateral ventricular size, presence (and severity) of a Chiari malformation, leg and foot position, lower extremity movement, and presence or absence of placenta previa or other potential contraindications to fetal or maternal surgery
Fetal echocardiography, to exclude any concurrent/occult structural cardiac defects
Fetal MR imaging of the brain and spine to confirm and supplement the sonographic findings
Maternal physical examination and consultation by anesthesia and perinatology
Psychosocial evaluation to identify maternal suitability for surgery. In addition to being screened for depression and anxiety, it is critical that the patient have adequate support available to accommodate her inability to care for herself and her other children for most of her pregnancy following prenatal surgery, and that she be capable of understanding and complying with the lifelong proscription against labor.
Preoperative teaching about NTDs, latex allergy, and community resources; perioperative instruction regarding the surgery itself and the tocolytic program; postoperative management in the hospital; postoperative care and recommendations following discharge; prenatal care; recommendations for the continued care of the child
22.5.3 Imaging Studies
A detailed sonographic examination is able to determine a number of anatomical features with precision. These include the size of the overlying CSF-containing sac, the level of the defect, the position of the cerebellar tonsils, the presence of lower extremity deformities, and potential concurrent nonneurologic anomalies. The limitations include difficulty in determining the number of associated brain anomalies, other intraspinal anomalies, and at times the exact dysraphic level.
Mothers should also have a fetal MR imaging study of the brain and spine. In order to minimize the effects of fetal motion, images in sagittal, axial, and coronal planes are obtained randomly by repeatedly imaging the fetus over time. The preferred MR imaging technique is a single-shot, fast spin-echo T2-weighted sequence. Evidence suggests that MR imaging may improve the ability to detect coexisting spinal and brain anomalies that may not be apparent on ultrasound studies.30,31
22.5.4 Maternal Counseling and Informed Consent
In order for the patient and family to provide a truly informed consent, every effort must be made to present an unbiased and balanced description of the potential benefits of prenatal myelomeningocele repair versus the surgical risks. Potential candidates for fetal surgery should meet with all members of the team: fetal surgeon, perinatologist, pediatric neurosurgeon, anesthesiologist, social worker, and spina bifida nurse coordinator. Critical issues for discussion include the following: (1) maternal surgical risk; (2) risk for preterm birth that would otherwise be essentially nonexistent, and the sequelae of preterm birth at a variety of gestational ages; (3) details of the postoperative course, which can be quite challenging and unexpected for a previously healthy patient; (4) potential postoperative complications, such as tocolytic toxicity, pulmonary edema, blood clots, oligohydramnios, and preterm premature rupture of membranes (PPROM); (5) risk for uterine dehiscence and rupture in the setting of a fresh hysterotomy, (6) need for the avoidance of labor and the requirement for cesarean delivery in all future pregnancies; and (7) a balanced and accurate review of the outcomes reported in MOMS.
Maternal risks of the procedure are those of general and regional anesthesia, bleeding and the potential need for blood products, and intraoperative complications, including the need for fetal resuscitation and, rarely, fetal death or maternal death. Additional maternal risks include postoperative complications, such as infection, pulmonary embolism, and oligohydramnios; however, the most common complication is that of preterm labor and preterm birth. It is essential for parents to understand that a pregnancy complicated by a fetal myelomeningocele that does not undergo fetal surgical repair has the same risk for preterm birth as that of the general obstetric population—and that this risk is significantly increased by prenatal surgery with an open hysterotomy. Neurologic outcomes for infants with a myelomeningocele may be compounded by complications of prematurity that might not have otherwise happened, particularly if delivery occurs at a vulnerable gestational age. These risks are weighed against the potential benefits and the improved quality of life that those affected by spinal cord defects may experience following prenatal surgical repair.
22.5.5 Anesthetic Considerations
Open fetal procedures that require a uterine incision (e.g., myelomeningocele repair) are performed under general anesthesia, which provides the needed uterine relaxation with the use of volatile anesthetic agents. In fact, the primary difference between fetal surgery and a cesarean delivery is that with the latter, the normal reflexive uterine contraction is relied upon to help achieve hemostasis. During fetal surgery, the same contractile force would result in inadequate blood flow to the umbilical cord and potential placental separation, which must be avoided.
The potential consequences of the profound uterine relaxation achieved with this approach to anesthesia include an increased potential for significant maternal hypotension resulting in decreased uterine blood flow and alterations in fetal hemodynamics. An epidural catheter is typically placed preoperatively for smoother emergence from general anesthesia and for postoperative analgesia. The advent of instrumentation specifically designed for fetal surgery has been critical in helping to prevent hemorrhage that would otherwise result in the setting of the necessary uterine relaxation and a fresh uterine incision.
22.5.6 Preoperative Treatment
Preoperatively, a nonparticulate antacid is given for prophylaxis against aspiration, rectal indomethacin is placed as an additional prophylactic tocolytic, and an epidural catheter is inserted. The position and efficacy of the epidural catheter are verified with a preoperative test dose (epidural placement often occurs before entry into the operating room, allowing time for a test dose). Blood products (type and crossmatch) are obtained for both mother and fetus. Sequential compression devices are placed on the mother’s lower extremities to minimize the risk for deep venous thrombosis. Minimal doses of preanesthetic medications (opioids or anxiolytics) are admininstered in order to reduce the incidence of hypotension when maximal doses of a volatile halogenated agent are administered. When any general anesthetic is administered after approximately 20 weeks of gestation, the patient is (1) positioned with left uterine displacement and (2) adequately preoxygenated, after which (3) rapid-sequence induction and tracheal intubation are performed with cricoid pressure. Before incision, anesthesia is typically maintained with low concentrations of a volatile agent. During this time, the sonologist determines the fetal presentation and maps out the placental location and edges, additional large-bore vascular access is obtained, the urinary bladder is catheterized, and prophylactic antibiotics are administered.
22.5.7 Intraoperative Treatment
During surgery, 2 to 3 MAC (minimal anesthetic concentration) of a volatile anesthetic agent are used to provide maternal and fetal anesthesia and surgical tocolysis (uterine atony). Volatile anesthetic agents inhibit myometrial contractility by calcium-sensitive potassium channel modulation.32 The human uterus has a thick, muscular layer that is sensitive to stimulation or manipulation. Thus, uterine incision and stimulation may produce strong uterine contractions. Halogenated anesthetics cause a dose-dependent inhibition of uterine contractions and myometrial tone. Complete uterine relaxation is essential because increased uterine tone compromises uterine perfusion, especially during uterine manipulation. The increased tone increases the risk for partial placental separation. When needed, high doses of intravenous nitroglycerin may also be used as a supplemental agent for intraoperative uterine relaxation.33
Once hysterotomy closure begins, the inspired concentrations of halogenated agents are slowly decreased. Discontinuing a high-dose volatile halogenated agent at the beginning of surgical closure allows more time for its elimination from the tissues. To combat the increase in uterine tone that results when volatile gas concentrations are decreased, a loading dose of 4 to 6 g of magnesium sulfate is simultaneously administered intravenously over 20 minutes, followed by a continuous infusion of 1 to 2 g/h. This infusion continues for at least 24 hours postoperatively in an attempt to maintain uterine relaxation and minimize postoperative contractions (see later section on postoperative care). Intraoperatively, maternal intravenous fluids are restricted (< 2 L) in order to minimize the risk for postoperative pulmonary edema, which is often associated with the use of tocolytic agents,34,35 particularly in the context of maternal surgery and general anesthesia. Inspired halogenated agents are discontinued once the continuous infusion of magnesium sulfate is running, and maternal anesthesia is maintained by the activation of epidural anesthesia and the intravenous administration of fentanyl and/or nitrous oxide in oxygen. If neuromuscular agents are utilized, the absence of neuromuscular blockade should be determined before tracheal extubation because magnesium sulfate potentiates neuromuscular blockade.
Whatever anesthetic technique is used for open fetal surgery, it must ensure adequate uteroplacental perfusion, profound uterine relaxation, maternal hemodynamic stability, fetal anesthesia and immobility, and minimal fetal myocardial depression and compromise. Excessive volume replacement should be avoided—particularly in conjunction with maternal tocolysis—in order to prevent maternal pulmonary edema, which has historically been a significant cause of postoperative morbidity.
22.5.8 Hysterotomy and Exposure
Successful open fetal surgery requires the execution of several critical technical steps: (1) optimizing the hysterotomy location, (2) opening the highly vascular and perfused gravid uterus with a minimum of bleeding while simultaneously preventing separation of the chorioamniotic membranes, (3) ensuring fetal stability throughout the procedure, (4) maintaining uterine relaxation, (5) achieving a watertight closure of the hysterotomy following the reinfusion of fluid into the amniotic cavity at the conclusion of the procedure, and (6) initiating aggressive intraoperative tocolysis before extubation to prevent preterm labor.1
An epidural catheter is placed and a test dose given before the procedure, so that it is ready for use immediately postoperatively for pain control. The patient is then anesthetized with a halogenated agent for uterine relaxation. The fetus is monitored throughout with continuous intraoperative ultrasound and, when necessary, fetal echocardiography. A generously sized low transverse maternal skin incision is made and carried through the fascia and into the intraperitoneal cavity. For optimal exposure, and to prevent compression of the engorged uterine vessels, the medial portion of the maternal rectus muscles may be split and the epigastric arteries identified and ligated. The uterus is exposed and the placental location re-evaluated. If the placenta is completely anterior, the uterus will be delivered and tipped forward to allow posterior surgical access. The fetal lie is evaluated by the perinatologist for optimal surgical positioning; if needed, an external version can be performed at this time through the relaxed, hypotonic uterus, so that the incision can be placed directly over where the fetal back is positioned. Before hysterotomy, a narcotic is administered to the fetus intramuscularly with ultrasound guidance (usually in the shoulder or rump). With care taken to avoid the placental edge, the hysterotomy is initiated with the placement of two stay sutures that will be utilized to place a specially designed absorbable uterine stapler device that provides hemostasis and seals the membranes to the myometrium.
The uterine incision is usually between 6 and 8 cm in length. After the hysterotomy is completed and hemostasis is ensured, a catheter is placed into the uterus to allow the continuous infusion of warm lactated Ringer solution around the fetus to maintain fetal temperature and avoid umbilical cord compression. With the continuous infusion of fluid, the fetus will float in the uterine cavity, which helps to push the torso upward against the open hysterotomy. This is preferred because the fetus will otherwise tend to move or drift during the surgery, which can make the neurosurgical repair difficult. The fetus is rarely removed from the uterus in order to avoid unnecessary manipulation, dehydration, hypothermia, or umbilical cord spasm. After repair of the fetal defect, a two-layer closure of the hysterotomy is begun, and several boluses of warm lactated Ringer solution are added through the catheter to restore a low-normal amniotic fluid level (as determined by intraoperative ultrasound).
22.5.9 Surgical Repair of the Fetal Defect
The anatomical features of the fetal myelomeningocele defect are visually very distinct, and determining the different structures is easier than at full term. The neural placode is always in the midline surrounded by an extremely thin layer of translucent arachnoid (▶ Fig. 22.2a). If the myelomeningocele sac is present, the placode is usually lifted upward away from the surface of the back. In some other situations, the placode is flat and at the same level as the surrounding skin. The epithelium of the skin does not usually reach the edge of the placode. The clear identification of the intervening arachnoid usually allows the placode to be divided from its attachments with sharp dissection.
Fig. 22.2 Fetal closure of a myelomeningocele lesion. (a) The fetal myelomeningocele is framed in the hysterotomy incision. The fetus is not removed from the uterus, and every effort is made to maintain the amniotic fluid volume. The actual sac consists of thin membranous tissue with a minimum of skin extending upward toward the placode. (b) The skin is closed as a single layer, in this case without a patch being required. (c) After delivery at 36 weeks, the wound appears well closed, although the sutures, which are of the monofilament absorbable type, are still visible.
(Reproduced with permission of the publisher from Jallo G, Kothbauer KF, Pradilla G, eds. Controversies in Pediatric Neurosurgery. New York, NY: Thieme Medical Publishers; 2010, chap. 16.)
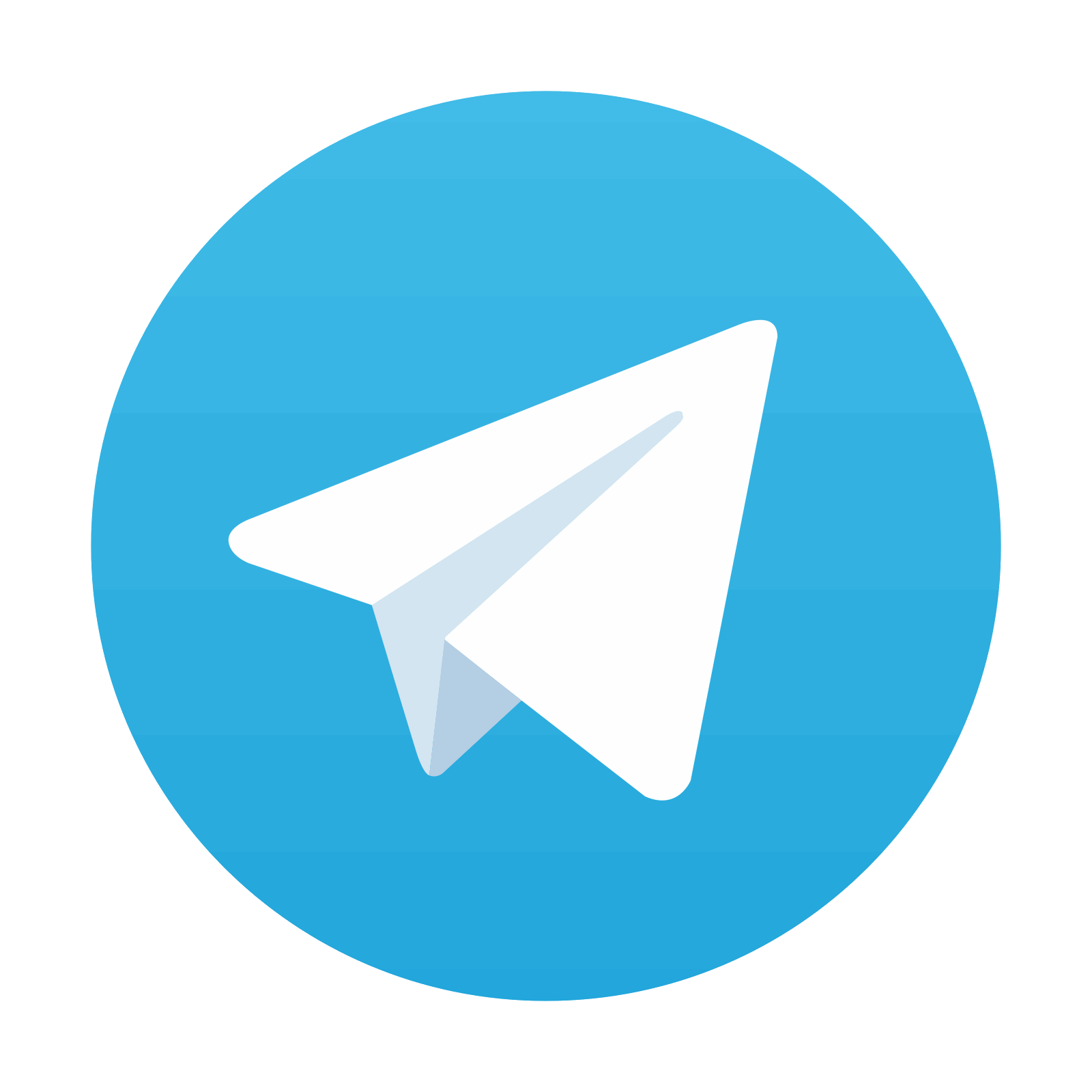