Symptom
Excessive daytime sleepiness (EDS)
A persistent sense of mental cloudiness, a lack of energy, a depressed mood, or extreme exhaustion. Some people experience memory lapses, and many have great difficulty maintaining their concentration while at school, work, or home
Cataplexy
Loss of muscle tone while you’re awake. Muscle weakness affects part or all of your body. It is often triggered by sudden, strong emotions such as fear, anger, stress, excitement, or humor
Rapid entry into REM sleep
Patients may enter the REM or dream phase of sleep right after falling asleep, whereas most people take about 90 min to enter REM. Therefore, they experience the characteristics of REM sleep (vivid dreams and muscle paralysis) at the beginning of sleep, even if that sleep is during the day
Sleep paralysis
The temporary inability to move or speak while falling asleep or waking is similar to REM-induced inhibitions of voluntary muscle activity, lasting from a few seconds to minutes. After episodes end, people rapidly recover their full capacity to move and speak
Hallucinations
Unusually vivid hallucinations can occur when people are falling asleep, waking, or during sleep. Referred to as hypnagogic hallucinations when occurring during sleep onset and as hypnopompic hallucinations when occurring during waking
Disrupted nocturnal sleep
Most patients experience difficulties staying asleep or waking up repeatedly throughout the night
Obesity
After developing narcolepsy, many individuals suddenly gain weight
Other
Leg jerks, nightmares, and restlessness
The Hcrt was linked to human narcolepsy since it was discovered in a mutation in the Hcrt receptor in mice (Chemelli et al. 1999) and dogs (Lin et al. 1999). Once it was speculated that a deficiency in the Hcrt system might be the explanation for human narcolepsy, diverse groups explored this possible link. Indeed, Hcrt system was linked to human narcolepsy.
In a study of post-mortem brains of human narcoleptics, for instance, a massive reduction in the number of Hcrt-containing cells (85–98 %) was discovered compared with healthy controls (Peyron et al. 2000; Thannickal et al. 2000). On the other hand, narcoleptic patients have reduced cerebrospinal fluid (CSF) levels of Hcrt (Dalal et al. 2001; Kanbayashi et al. 2002; Mignot et al. 2002; Nishino et al. 2000; Ripley et al. 2001), a finding consistent with the loss of Hcrt-containing neurons as mentioned above. The CSF measurements of Hcrt provide a valuable diagnostic tool for narcolepsy, separating narcolepsy from other sleep and neurological disorders (Mignot et al. 2002).
3 Animal Models of Narcolepsy
There are experimental models that mimics the sleep disorder narcolepsy; for instance, Hcrt/orexin genes knockout mice (Chemelli et al. 1999), canines with a mutation in the Hcrt-2 receptor (Lin et al. 1999) or mice with a targeted destruction of the Hcrt neurons (Hara et al. 2001) that exhibit symptoms of narcolepsy (Fig. 1).
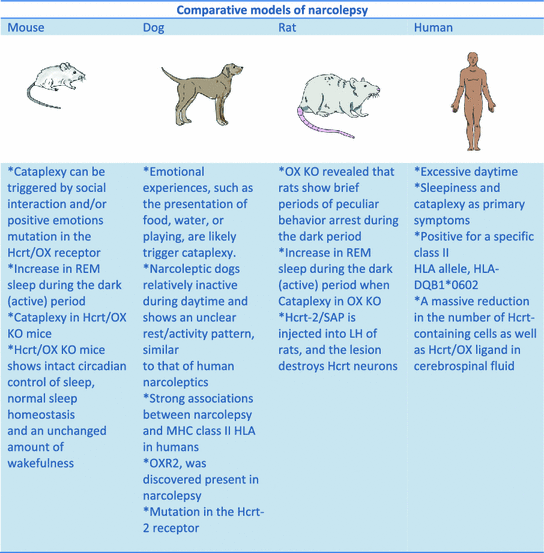
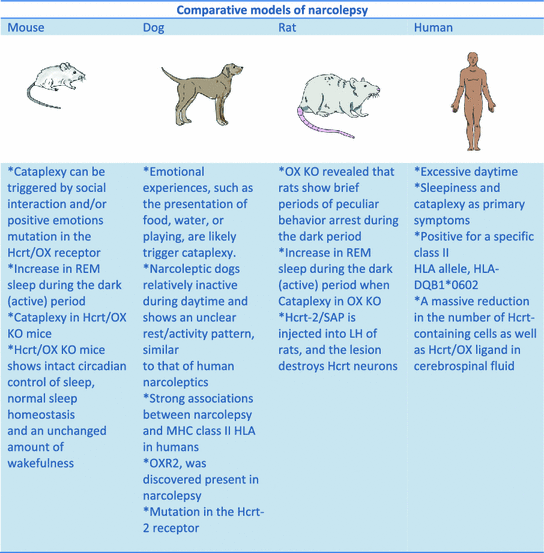
Fig. 1
Comparison of the available experimental models of nacolepsy: Mice, rat. dog and human. Please note the molecular, neurochemical, behavioral similarities among models. Abbreviations HLA Human leukocyte antigen; Hcrt hypocretin; KO knock out; OX orexin; OX R2 orexin receptor 2; REM rapid eye movement sleep
Recently, our group and other have generated an experimental model using a toxin which represents a very reliable procedure. This model consists of in a ribosome-inactivating protein saporin (SAP) (Stirpe et al. 1992) that is conjugated to the hypocretin/orexin receptor that binds ligand hypocretin-2/orexin-B (Hcrt-2) to lesion Hcrt receptor-bearing neurons. It is known that the LH contains a high concentration of Hcrt receptor mRNA (Trivedi et al. 1998) as well as immunoreactivity (Hervieu et al. 2001). This fact indicates of that presence of the Hcrt receptor on Hcrt neurons. When the Hcrt-2/SAP is injected into LH of rats, the toxin lesions Hcrt neurons, and produces behavioural symptoms that are characteristic of narcolepsy.
The Hcrt-2/SAP binds to cells containing the Hcrt receptor but does not bind to cells that do not contain the Hcrt receptor. This indicates the specificity of the toxin. For instance, the diurnal rhythm of wakefulness (W) and slow wave sleep (SWS) in a rat lesion is attenuated. This is because of an increase in sleep during the lights-off period. Hcrt-2/SAP increases SWS over the 24 h period. Total time spent in SWS and rapid eye movement sleep (REMS) was found to correlate with a decline in number of Hcrt neurons.
Using this model, Gerashchenko et al. (2001) showed that Hcrt-2/SAP induced more SWS and REM sleep at night and multiple periods of abnormal behavioural arrest during purposeful behaviour. Indeed, this experimental model of narcolepsy provides a method of investigating the contribution of the Hcrt system to the regulation of the sleep-wake cycle and its relationship with narcolepsy. Gerashchenko et al. (2003) also reported that two concentrations (90 ng or 490 ng/0.5 μl) of the Hcrt-2/SAP injected directly to the lateral hypothalamus caused a significant Hcrt cell lost. Narcoleptic-like sleep behaviour was produced by both concentrations of this toxin (Gerashchenko et al. 2003).
As a conclusion, the use of Hcrt-2/SAP induced characteristic of narcolepsy, such as sleep fragmentation, sleep-onset REM sleep periods, increased NREM sleep and REM sleep time during the normally active lights-off period (Gerashchenko et al. 2001, 2003).
The relationship between Hcrt neuronal loss and changes in levels of the peptide was not known. Using the Hcrt-2/SAP to lesion neurons in the LH was found that there was a 50 % reduction in CSF Hcrt levels when 73 % of the number of Hcrt neurons was lost (Gerashchenko et al. 2003). The sleep deprivation method is used to test the homeostatic mechanism of sleep regulation. It was also found that in lesioned rats, the Hcrt levels were not increased by 6 h prolonged W, indicating that surviving neurons were not able to increase the output of Hcrt into CSF to compensate for the Hcrt neuronal loss.
In the same study, the authors showed that Hcrt levels in CSF measured at different times of the day-night cycle showed that control rats had significantly higher Hcrt levels at ZT0 (+72.9 %) compared to a different time point (ZT8). The rats with a 72 % Hcrt neuronal loss did not show a significant difference between ZT0 and ZT8. Surprisingly, it was found a significant correlation between Hcrt neurons and Hcrt levels at ZT0 (Gerashchenko et al. 2003).
The Hcrt neurons begin to appear on embryonic day 19 E19 and are fully developed by postnatal day 20 (Yamamoto et al. 2000; Van Den Pol et al. 2001). As reported by others (Fujiki et al. 2001; Yoshida et al. 2001), Hcrt display a diurnal rhythm in young animals.
Such a rhythm of Hcrt, however, has not been determined in aged animals. It was measured CSF Hcrt levels at 4-h intervals across a 24-h period to test the hypothesis that there was a decline in Hcrt levels in aged rats. In agreement with previous studies in young rats (Fujiki et al. 2001) peak levels of Hcrt were found at the end of the wake-active period (ZT0), and lowest levels occurred at the end of the sleep period (ZT12). This profile was present in young and old rats. The authors found, however, that the old rats had a significant reduction of Hcrt levels in CSF compared to the young rats. Moreover, the aged rats had significantly less CSF Hcrt levels compared to young rats as well (Desarnaud et al. 2004).
As mentioned above, CSF Hcrt levels are increased in response to prolonged W, we tested if Hcrt levels could be enhanced in aged animals after prolonged W. Rats were kept awake for 8 h to drive the activity of the Hcrt neurons, and CSF was collected in order to measure Hcrt. All groups of rats had significantly increased CSF Hcrt levels in response to 8 h of prolonged waking (Desarnaud et al. 2004), a finding that is consistent with other studies (Yoshida et al. 2001).
The overall CSF Hcrt levels after 8 h of prolonged W, however, were still lower in the old rats when compared to the young rats. The possible explanation for this phenomenon might be a decline in Hcrt-1 in old rats reflecting a diminution in prepro-Hcrt gene expression across the aging process. We therefore measured prepro-Hcrt mRNA levels in the posterior hypothalamus of young and old rats by Northern blot analysis. No difference in mRNA between age group was found (Desarnaud et al. 2004).
4 Narcolepsy: Current Treatment Options
What are the approaches that currently are used to treat narcolepsy? The goal of all therapeutic approaches in narcolepsy is to control the narcoleptic symptoms and to allow the patient to continue full participation in familial and professional daily activities. Pharmacological treatments for narcolepsy include the use of amphetamines and modafinil whereas cataplexy is treated with tricyclic antidepressants such as clomipramine (Mignot et al. 2002; Scammell 2003; Mignot and Nishino 2005).
5 Cell Transplantation: A Future Therapy for Narcolepsy?
Neural transplantation is one of the most promising approaches for the treatment of Parkinson’s disease (PD), a major neurodegenerative disorder with a prevalence as frequent as that of narcolepsy (Arias-Carrión et al. 2004). Neural transplantation involves implantation of living neuronal tissue into a host system. Several studies using animal models have demonstrated that grafted tissue survives, integrates within the host brain, and provides functional recovery following brain inventions (Drucker-Colín and Verdugo-Díaz 2004; Lindvall et al. 2004). This type of study for PD began in the latter half of the 1970s. Initially, DA neurons from animal fetuses were used as donors, and then paraneurons such as chromaffin cells were used (Drucker-Colín and Verdugo-Díaz 2004). Based on a large number of experimental animal studies, neural transplantation has been applied clinically (Drucker-Colín and Verdugo-Díaz 2004; Lindvall et al. 2004). Studies in patients with PD after intrastriatal transplantation of human fetal mesencephalic tissue, rich in postmitotic dopaminergic neurons, have provided proof of principle that neuronal replacement can work in the human brain (Lindvall et al. 2004). The grafted neurons survive and reinnervate the striatum for as long as 10 years despite an ongoing disease process, which destroys the patient’s own dopaminergic neurons (Drucker-Colín and Verdugo-Díaz 2004). The grafts are able to normalize striatal dopamine release (Drucker-Colín and Verdugo-Díaz 2004) and to reverse the impairment of cortical activation underlying akinesia. Thus, grafted dopaminergic neurons can become functionally integrated into neuronal circuitries in the brain (Drucker-Colín and Verdugo-Díaz 2004; Lindvall et al. 2004). Several open-label trials have reported clinical benefit (for revision see Lindvall et al. 2004). Some patients have been able to withdraw from L-dopa treatment for several years and resume an independent life (Drucker-Colín and Verdugo-Díaz 2004; Lindvall et al. 2004). Beneficial effects have been demonstrated, and autopsy cases have shown that many transplanted cells were able to survive in the human brain for long periods. These findings contributed a great deal to the research in regeneration of the central nervous system.
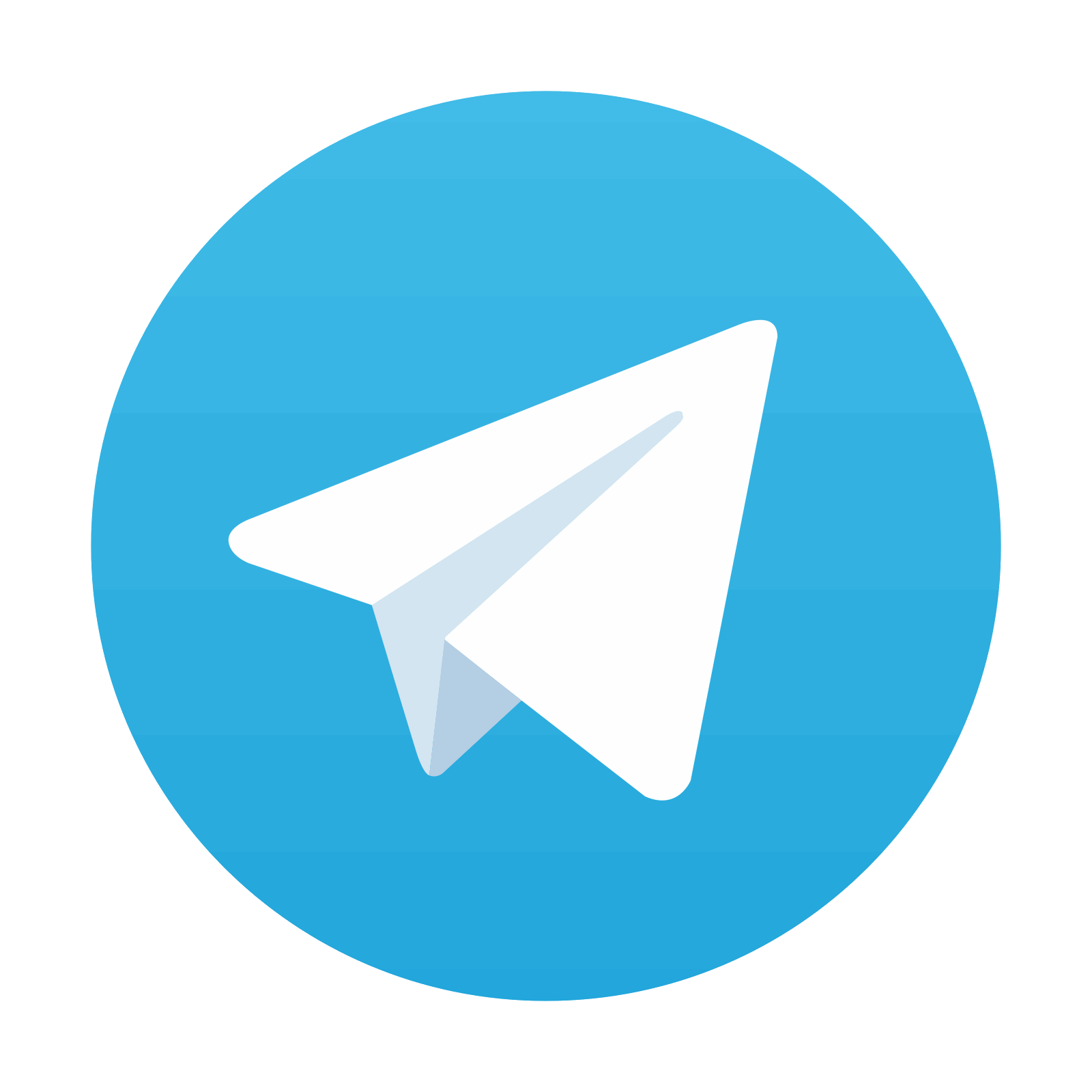
Stay updated, free articles. Join our Telegram channel

Full access? Get Clinical Tree
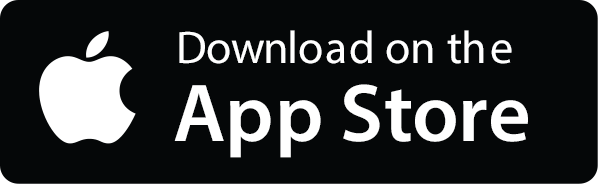
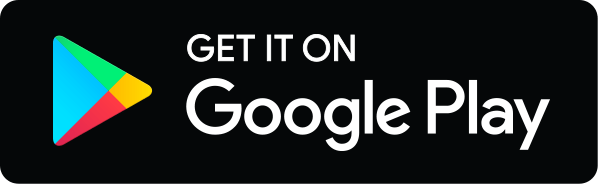