Pain
Noxious Insults Activate Nociceptors
Signals from Nociceptors Are Conveyed to Neurons in the Dorsal Horn of the Spinal Cord
Hyperalgesia Has Both Peripheral and Central Origins
Nociceptive Information Is Transmitted from the Spinal Cord to the Thalamus
Five Major Ascending Pathways Convey Nociceptive Information
Several Thalamic Nuclei Relay Nociceptive Information to the Cerebral Cortex
Pain Is Controlled by Cortical Mechanisms
Cingulate and Insular Areas Are Active During the Perception of Pain
Opioid Peptides Contribute to Endogenous Pain Control
Endogenous Opioid Peptides and Their Receptors Are Distributed in Pain-Modulatory Systems
PAIN DESCRIBES THE UNPLEASANT sensory and emotional experiences associated with actual or potential tissue damage. Pricking, burning, aching, stinging, and soreness are among the most distinctive of all the sensory modalities. As with the other somatic sensory modalities—touch, pressure, and position sense—pain serves an important protective function, alerting us to injuries that require evasion or treatment. In children born with insensitivity to pain, severe injuries often go unnoticed and can lead to permanent tissue damage. Yet pain is unlike other somatic sensory modalities, or vision, hearing, and smell in that it has an urgent and primitive quality, possessing both affective and emotional components.
The perception of pain is subjective and is influenced by many factors. An identical sensory stimulus can elicit quite distinct responses in the same individual under different conditions. Many wounded soldiers, for example, do not feel pain until they have been removed from the battlefield; injured athletes are often not aware of pain until a game is over. Simply put, there are no purely “painful” stimuli, sensory stimuli that invariably elicit the perception of pain in all individuals. The variability of the perception of pain is yet another example of a principle that we have encountered in earlier chapters: Pain is not the direct expression of a sensory event but rather the product of elaborate processing by the brain of a variety of neural signals.
When pain is experienced it can be acute, persistent, or in extreme cases chronic. Persistent pain characterizes many clinical conditions and is usually the reason that patients seek medical attention. In contrast, chronic pain appears to have no useful purpose; it only makes patients miserable. Pain’s highly individual and subjective nature is one of the factors that make it so difficult to define objectively and to treat clinically.
In this chapter we discuss the neural processes that underlie the perception of pain in normal individuals and explain the origins of some of the abnormal pain states that are encountered clinically.
Noxious Insults Activate Nociceptors
Many organs in the periphery, including skin and subcutaneous structures such as joints and muscles, possess specialized sensory receptors that are activated by noxious insults. Unlike the specialized somatosensory receptors for light touch and pressure, most of these nociceptors are simply the free nerve endings of primary sensory neurons. There are three main classes of nociceptors—thermal, mechanical, and polymodal—as well as a more enigmatic fourth class, termed silent nociceptors.
Thermal nociceptors are activated by extremes in temperature, typically greater than 45°C (115°F) or less than 5°C (41°F). They are the peripheral endings of small-diameter, thinly myelinated AΔ axons that conduct action potentials at speeds of 5 to 30 m/s (Figure 24–1A). Mechanical nociceptors are activated optimally by intense pressure applied to the skin; they too are the endings of thinly myelinated AΔ axons. Polymodal nociceptors can be activated by high-intensity mechanical, chemical, or thermal (both hot and cold) stimuli. This class of nociceptors is found at the ends of small-diameter, unmyelinated C axons that conduct more slowly, at speeds less than 1.0 m/s (Figure 24–1A).
Figure 24-1 Propagation of action potentials in different classes of nociceptive fibers.
A. The speed at which action potentials are conducted is a function of each fiber’s cross-sectional diameter. Wave peaks in the figure are labeled alphabetically in order of latency. The first peak and its subdivisions are the summed electrical activity of myelinated A fibers. A delayed (slowly conducting) deflection represents the summed action potentials of unmyelinated C fibers. The compound action potential of the A fibers is shown on a faster time-base to depict the summation of the action potentials of several fibers. (Modified, with permission, from Perl 2007.)
B. First and second pain are carried by two different primary afferent fibers. (Modified, with permission, from Fields 1987.)
These three classes of nociceptors are widely distributed in skin and deep tissues and are often coactivated. When a hammer hits your thumb, you initially feel a sharp pain (“first pain”) followed by a more prolonged aching and sometimes burning pain (“second pain”) (Figure 24–1B). The fast sharp pain is transmitted by AΔ fibers that carry information from damaged thermal and mechanical nociceptors. The slow dull pain is transmitted by C fibers that convey signals from polymodal nociceptors.
Silent nociceptors are found in the viscera. This class of receptors is not normally activated by noxious stimulation; instead, inflammation and various chemical agents dramatically reduce their firing threshold. Their activation is thought to contribute to the emergence of secondary hyperalgesia and central sensitization, two prominent pain syndromes.
Noxious stimuli depolarize the bare nerve endings of afferent axons and generate action potentials that are propagated centrally. How is this achieved? The membrane of the nociceptor contains receptors that convert the thermal, mechanical, or chemical energy of noxious stimuli into a depolarizing electrical potential. One such protein is a member of a large family of so-called transient receptor potential (TRP) ion channels. This receptor-channel, TRPV1, is expressed selectively by nociceptive neurons and mediates the pain-producing actions of capsaicin, the active ingredient of hot peppers, and many other pungent chemicals. The TRPV1 channel is also activated by noxious thermal stimuli, which suggests that it normally transduces the sensation of painful heat. In addition, TRPV1-mediated membrane currents are enhanced by a reduction in pH, a characteristic of the chemical milieu of inflammation.
Additional members of the TRP channel family are expressed by nociceptive neurons, and the variety of TRP channels in nociceptors is thought to underlie the perception of a wide range of temperatures from extreme cold to intense heat. The TRPV2 channel is expressed predominantly in AΔ fiber terminals and is activated by very high temperatures, whereas the TRPM8 channel is activated by low temperatures and by chemicals such as menthol (Figure 24-2).
Figure 24-2 Transient receptor potential ion channels in nociceptive neurons.
A. Xenopus oocytes are injected with mRNA encoding transient receptor potential (TRP) channels. Electrophysiological recordings from the oocytes reveal the thermosensitivity of the channels. The temperature (centigrade) at which a specific TRP channel is activated is shown by the downward deflection of the recording. (Photograph on left reproduced, with permission, from Erwin Sigel 1987. Traces on the right reproduced, with permission, from Tominaga and Caterina 2004.)
B. Temperature response profiles of different TRP channels expressed by dorsal root ganglion neurons. (Modified, with permission, from Jordt, McKemy, and Julius 2003; Dhaka, Viswanath, and Patapoutian 2006.)
C. Bradykinin (BK) binds to G protein-coupled receptors on the surface of primary afferent neurons to activate phospholipase C (PLC), leading to the hydrolysis of membrane phos-phatidylinositol bisphosphate (PIP2), the production of inositol 1,4,5-trisphosphate (IP3), and the release of Ca2+ from intracellular stores. Activation of protein kinase C (PKC) regulates TRP channel activity. The TRPV1 channel is sensitized, leading to channel opening and Ca2+ influx. (Modified, with permission, from Bautista et al. 2007.)
In addition to this constellation of TRP channels, other receptors and ion channels that participate in the transduction of peripheral stimuli are expressed in nociceptive sensory endings. Nociceptors selectively express tetrodotoxin-resistant Na+ channels. One Na+ channel (SCN9A, also called Nav1.7) plays a key role in the perception of pain in humans, as revealed by the rare pain-insensitive individuals who possess mutations in the corresponding gene. One class of mutations inactivates the SCN9A channel and results in a complete inability to sense pain. But in all other respects these individuals are healthy and exhibit normal sensory responses to touch, mild temperature, proprioception, tickle, and pressure. A second class of mutations in the SCN9A gene changes the inactivation kinetics of this channel; individuals with these mutations exhibit an inherited condition called paroxysmal extreme pain disorder, characterized by rectal, ocular, and submandibular pain.
Nociceptors also express an ionotropic purinergic receptor, PTX3, that is activated by adenosine triphosphate (ATP) released from peripheral cells after tissue damage. In addition, they express members of the Mas-related G protein-coupled receptor (Mrg) family, which are activated by peptide ligands and serve to sensitize nociceptors to other chemicals released in their local environment (see Figure 24-7). These receptors and channels provide attractive targets for the development of drugs with actions selective for nociceptive sensory neurons.
The uncontrolled activation of nociceptors is associated with several pathological conditions. Allodynia and hyperalgesia are two common pain states that reflect changes in nociceptor activity. Patients with allodynia feel pain in response to stimuli that are normally innocuous: by a light stroking of sunburned skin, by the movement of joints in patients with rheumatoid arthritis, and even by the act of getting out of bed in the morning after a vigorous workout. Nevertheless, patients with allodynia do not feel pain constantly; in the absence of a peripheral stimulus there is no pain. In contrast, patients with hyperalgesia—an exaggerated response to noxious stimuli—typically report persistent pain in the absence of sensory stimulation.
Persistent pain can be subdivided into two broad classes, nociceptive and neuropathic. Nociceptive pain results from the activation of nociceptors in the skin or soft tissue in response to tissue injury, and it usually arises from an accompanying inflammation. Sprains and strains produce mild forms of nociceptive pain, whereas arthritis or a tumor that invades soft tissue produces a much more severe nociceptive pain. Neuropathic pain results from direct injury to nerves in the peripheral or central nervous system and is often accompanied by a burning or electric sensation. Neuropathic pains include the syndromes of reflex sympathetic dystrophy, also called complex regional pain syndrome, and post-herpetic neuralgia, the severe pain experienced by patients after a bout of shingles. Other neuropathic pains include phantom limb pain, the pain that occurs after limb amputation, which we discuss below. In some instances pain can even occur without a peripheral stimulus, a phenomenon termed anesthesia dolorosa. This syndrome can be triggered following attempts to block chronic pain, for example after therapeutic transection of sensory afferent fibers in the dorsal roots.
Signals from Nociceptors Are Conveyed to Neurons in the Dorsal Horn of the Spinal Cord
The perception of noxious stimuli arises from signals in the peripheral axonal branches of nociceptive sensory neurons whose cell bodies are located in dorsal root ganglia or the trigeminal ganglia. The central branches of these neurons terminate in the spinal cord in a highly orderly manner. Most terminate in the dorsal horn. Primary afferent neurons that convey distinct sensory modalities terminate in different laminae (Figure 24–3B). Thus there is a tight link between the anatomical organization of dorsal horn neurons, their receptive properties, and their function in sensory processing.
Figure 24-3 Nociceptive fibers terminate in the dorsal horn of the spinal cord.
A. Peripheral nociceptor classes.
B. Neurons in lamina I of the dorsal horn receive direct input from myelinated (AΔ) nociceptive fibers and both direct and indirect input from unmyelinated (C) nociceptive fibers via interneurons in lamina II. Lamina V neurons receive low-threshold input from large-diameter myelinated fibers (Aβ) of mechanoreceptors as well as inputs from nociceptive afferent fibers (AΔ and C fibers). Lamina V neurons send dendrites to lamina IV, where they are contacted by the terminals of Aβ primary afferents. Dendrites in lamina III arising from cells in lamina V are contacted by the axon terminals of lamina II interneurons. Aα fibers innervate motor neurons and interneurons in the ventral spinal cord (not shown). (Modified, with permission, from Fields 1987.)
Many neurons in the most superficial lamina of the dorsal horn, termed lamina I or the marginal layer, respond to noxious stimuli conveyed by AΔ and C fibers. Because they respond selectively to noxious stimulation they have been called nociception-specific neurons. This set of neurons projects to higher brain centers, notably the thalamus. A second class of lamina I neurons receives input from C fibers that are activated selectively by intense cold. Other classes of lamina I neurons respond in a graded fashion to both innocuous and noxious mechanical stimulation and thus are termed wide-dynamic-range neurons.
Lamina II, the substantia gelatinosa, is a densely packed layer that contains many different classes of local interneurons, some excitatory and others inhibitory. Some of these interneurons respond selectively to nociceptive inputs, whereas others also respond to innocuous stimuli. Laminae III and IV contain a mixture of local interneurons and supraspinal projection neurons. Many of these neurons receive input from Aβ afferent fibers that respond to innocuous cutaneous stimuli, such as the deflection of hairs and light pressure. Lamina V contains neurons that respond to a wide variety of noxious stimuli and project to the brain stem and thalamus. These neurons receive direct inputs from Aβ and AΔ fibers and, because their dendrites extend into lamina II, are also innervated by C fiber nociceptors (Figure 24–3B).
Neurons in lamina V also receive input from nociceptors in visceral tissues. The convergence of somatic and visceral nociceptive inputs onto individual lamina V neurons provides one explanation for a phenomenon called “referred pain,” a condition in which pain from injury to a visceral tissue is perceived as originating from a region of the body surface. Patients with myocardial infarction, for example, frequently report pain from the left arm as well as the chest (Figure 24-4). This phenomenon occurs because a single lamina V neuron receives sensory input from both regions, and thus a signal from this neuron does not inform higher brain centers about the source of the input. As a consequence, the brain often incorrectly attributes the pain to the skin, possibly because cutaneous inputs predominate. Another anatomical explanation for instances of referred pain is that the axons of nociceptive sensory neurons branch in the periphery, innervating both skin and visceral targets.
Figure 24-4 Signals from nociceptors in the viscera can be felt as “referred pain” elsewhere in the body.
A. Myocardial infarction and angina can be experienced as deep referred pain in the chest and left arm. The source of the pain can be readily predicted from the site of referred pain.
B. Convergence of visceral and somatic afferent fibers may account for referred pain. Nociceptive afferent fibers from the viscera and fibers from specific areas of the skin converge on the same projection neurons in the dorsal horn. The brain has no way of knowing the actual site of the noxious stimulus and mistakenly associates a signal from a visceral organ with an area of skin. (Adapted, with permission, from Fields 1987.)
Neurons in lamina VI receive inputs from large-diameter fibers that innervate muscles and joints. These neurons are activated by innocuous joint movement and do not contribute to the transmission of nociceptive information. In contrast, many neurons located in laminae VII and VIII, the intermediate and ventral regions of the spinal cord, do respond to noxious stimuli. These neurons typically have complex response properties because the inputs from nociceptors to these neurons are conveyed through many intervening synapses. Neurons in lamina VII often respond to stimulation of either side of the body, whereas most dorsal horn neurons receive unilateral input. The activation of lamina VII neurons is therefore thought to contribute to the diffuse quality of many pain conditions.
Nociceptive sensory neurons that activate neurons in the dorsal horn of the spinal cord release two major classes of neurotransmitters. Glutamate is the primary neurotransmitter of all primary sensory neurons, regardless of sensory modality. Neuropeptides are released as cotransmitters by many nociceptors with unmyelinated axons. These peptides include substance P, calcitonin gene-related peptide (CGRP), somatostatin, and galanin (Figure 24-5). Glutamate is stored in small, electron-lucent vesicles, whereas peptides are sequestered in large, dense-core vesicles at the central terminals of nociceptive sensory neurons (Figure 24-6). Separate storage sites permit these two classes of neurotransmitters to be released under different physiological conditions.
Figure 24-5 Neuropeptides and their receptors in the superficial dorsal horn of the rat spinal cord. (Images from A. Basbaum, reproduced with permission.)
A. Substance P is concentrated in the terminals of primary sensory neurons in the superficial dorsal horn. Its receptor, neurokinin-1 antagonist (NK1) is also expressed by neurons in the superficial dorsal horn
B. Enkephalin is localized in interneurons and found in the same region of the dorsal horn as terminals containing substance P. The μ-opioid receptor for enkephalins is expressed by neurons in the superficial dorsal horn and also in sensory afferent terminals.
Figure 24-6 Transmitter storage in the synaptic terminals of primary nociceptive neurons in the dorsal spinal cord.
A. The terminal of a C fiber on the dendrite (D) of a dorsal horn neuron has two classes of synaptic vesicles that contain different transmitters. Small electron-lucent vesicles contain glutamate while large dense-cored vesicles hold neuropeptides. (Image from H. J. Ralston III, reproduced with permission.)
B. Glutamate and the peptide substance P are scattered in the axoplasm of a sensory terminal in lamina II of the dorsal horn. (Image from A. Rustioni, reproduced with permission.)
Of the neuropeptides expressed by nociceptive sensory neurons, the actions of substance P, a member of the neurokinin peptide family, have been studied in most detail. Substance P is released from the central terminals of nociceptive afferents in response to tissue injury or after intense stimulation of peripheral nerves. Its interaction with neurokinin receptors on dorsal horn neurons elicits slow excitatory postsynaptic potentials that prolong the depolarization elicited by glutamate. Although the physiological actions of glutamate and neuropeptides on dorsal horn neurons are different, these transmitters act coordinately to regulate the firing properties of dorsal horn neurons.
There is no efficient means for peptide reuptake into nerve terminals, so neuropeptides released from sensory terminals diffuse over a greater distance than glutamate and thus have excitatory influences on many dorsal horn neurons in the immediate vicinity of the release site. This diffuse signaling system may contribute to the poorly localized character of many pain conditions. In addition, the levels of neuropeptide expression in primary nociceptive neurons are elevated in some pathological conditions. Such reactive changes in peptide expression may contribute to the enhanced excitability of dorsal horn neurons that accompanies some chronic pain states.
Details of the interaction of neuropeptides with their receptors on dorsal horn neurons have suggested strategies for pain regulation. Infusion of substance P coupled to a neurotoxin into the dorsal horn of experimental animals results in selective destruction of neurons that express neurokinin receptors. Animals treated in this way fail to develop the central sensitization that is normally associated with peripheral injury. This method of neuronal ablation is more selective than traditional surgical interventions such as partial spinal cord transection (anterolateral cordotomy) and is being explored as a treatment for patients suffering from chronic pain syndrome.
Hyperalgesia Has Both Peripheral and Central Origins
Up to this point we have considered the mechanisms that convey noxious signals in the normal physiological state. But the normal process of sensory signaling can be dramatically altered when peripheral tissue is damaged, resulting in an increase in pain sensitivity or hyperalgesia. This condition can be elicited by sensitizing peripheral nociceptors through repetitive exposure to noxious mechanical stimuli (Figures 24-7).
Figure 24-7 Hyperalgesia results from sensitization of nociceptors. (Reproduced, with permission, from Raja, Campbell, and Meyer 1984.)
A. Mechanical thresholds for pain were recorded at sites A,
B. and C before and after burns at sites A and D. The areas of reddening (flare) and mechanical hyperalgesia resulting from the burns are shown on the hand of one subject. In all subjects the area of mechanical hyperalgesia was larger than the area of flare. Mechanical hyperalgesia was present even after the flare disappeared.
B. Mean mechanical pain thresholds before and after burns. The mechanical threshold for pain is significantly decreased after the burn.
The sensitization is triggered by a complex mix of chemicals released from damaged cells that accumulate at the site of tissue injury. This cocktail contains peptides and proteins such as bradykinin, substance P, and nerve growth factor, as well as molecules such as ATP, histamine, serotonin, prostaglandins, leukotrienes, and acetylcholine. Many of these chemical mediators are released from distinct cell types, but together they act to decrease the threshold of nociceptor activation.
Where do these chemicals come from and what exactly do they do? Histamine is released from mast cells after tissue injury and activates polymodal nociceptors. The lipid anandamide, an endogenous can-nabinoid agonist, is released under conditions of inflammation, activates the TRPV1 channel, and may trigger pain associated with inflammation. ATP, acetylcholine, and serotonin are released from damaged endothelial cells and platelets; they act indirectly to sensitize nociceptors by triggering the release of chemical agents such as prostaglandins and bradykinin from peripheral cells. Bradykinin is one of the most active pain-producing agents. Its potency stems in part from the fact that it directly activates AΔ and C nociceptors as well as increasing the synthesis and release of prostaglandins from nearby cells.
Damaged cells also release prostaglandins, metabolites of arachidonic acid that are generated through the activity of cyclooxygenase (COX) enzymes that cleave arachidonic acid. The COX2 enzyme is preferentially induced under conditions of peripheral inflammation, contributing to enhanced pain sensitivity. The enzymatic pathways of prostaglandin synthesis are targets of commonly used analgesic drugs. Aspirin and other nonsteroidal anti-inflammatory analgesics are effective in controlling pain because they block the activity of most COX enzymes, reducing prostaglandin synthesis. The drug acetaminophen appears to exert its analgesic effects through selective inhibition of COX3.
Tissue inflammation also results from peripheral injury. The cardinal signs of inflammation are heat (calor), redness (rubor), and swelling (tumor). Heat and redness result from the dilation of peripheral blood vessels, whereas swelling results from plasma extravasation, a process in which proteins, cells, and fluids are able to penetrate post-capillary venules. Release of the neuropeptides substance P and CGRP from the peripheral terminals of C fibers can elicit each of these pathological responses. Because this form of inflammation depends on neural activity, it has been termed neurogenic inflammation (Figure 24–8).
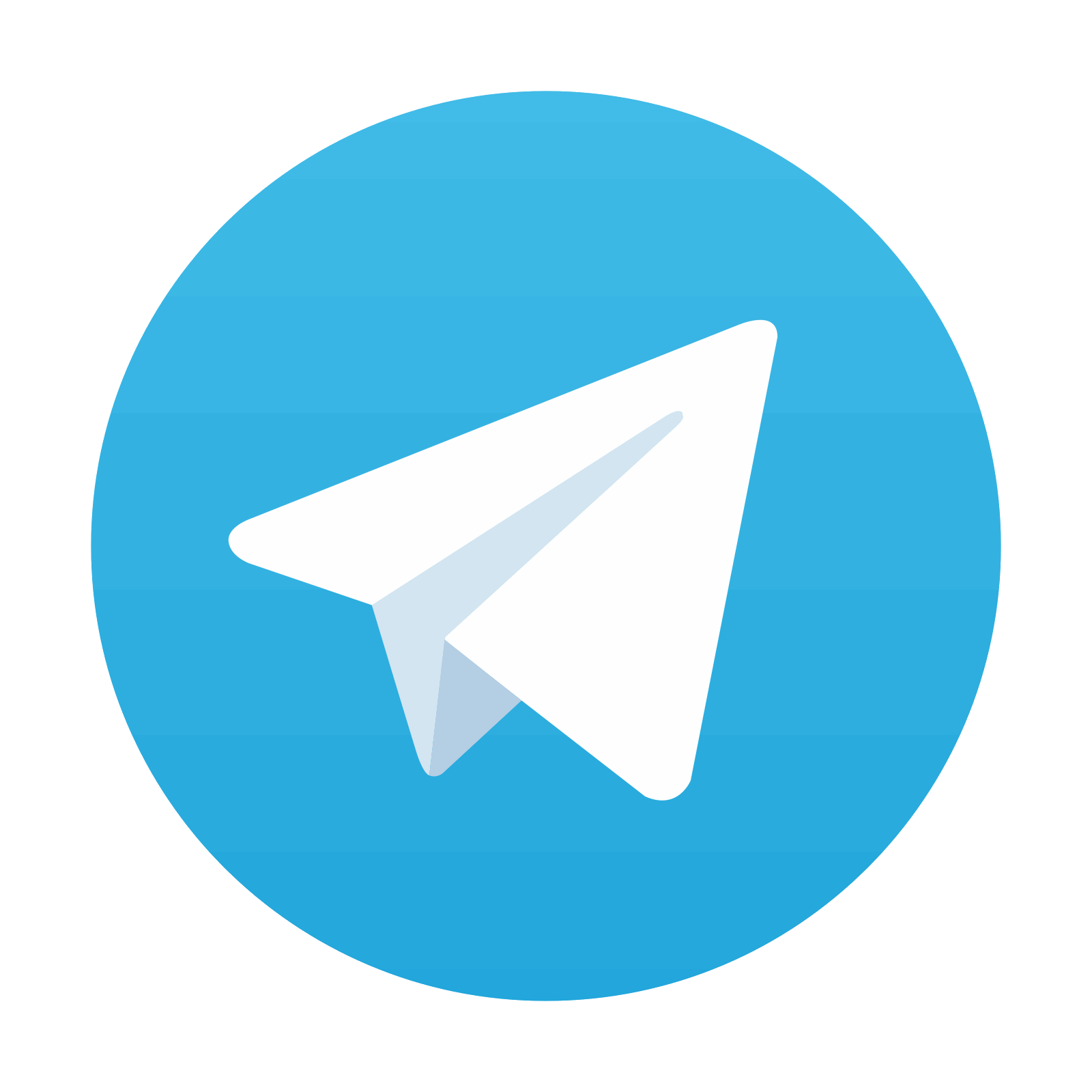
Stay updated, free articles. Join our Telegram channel

Full access? Get Clinical Tree
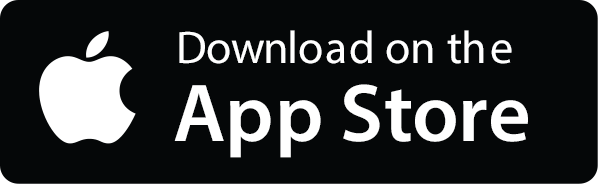
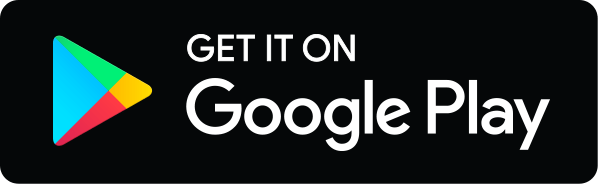