Somnambulism
Sleep terrors
(A) Ambulation occurs during sleep
(B) Persistence of sleep, an altered state of consciousness or impaired judgment during ambulation demonstrated by at least one of the following:
i. Difficulty in arousing the person
ii. Mental confusion when awakened from an episode
iii. Amnesia (complete or partial) for the episode
iv. Routine behaviors that occur at inappropriate times
v. Inappropriate or nonsensical behaviors
vi. Dangerous or potentially dangerous behaviors
(C) The disturbance is not better explained by another sleep disorder, medical or neurologic disorder, mental disorder, medication use, or substance use disorder
(A) A sudden episode of terror occurs during sleep, usually initiated by a cry or loud scream that is accompanied by autonomic nervous system and behavioral manifestations of intense fear
(B) At least one of the following associated features is present:
i. Difficulty in arousing the person
ii. Mental confusion when awakened from an episode
iii. Amnesia (complete or partial) for the episode
iv. Dangerous or potentially dangerous behaviors
(C) The disturbance is not better explained by another sleep disorder, medical or neurologic disorder, mental disorder, medication use, or substance use disorder
Experimental sleep deprivation can be used to trigger episodes of somnambulism in the sleep laboratory, since these rarely occur spontaneously in laboratory conditions. In fact, 25–38 h of sleep deprivation can significantly increase the number of actual somnambulistic events recorded in the laboratory by a factor of 2.5–5 when compared to baseline [6, 40, 41]. The fact that none of the control subjects investigated in these studies experienced nocturnal behavioral manifestations in the laboratory demonstrates that sleep deprivation alone does not lead to somnambulistic episodes, but rather that it increases the probability of somnambulistic behaviors in predisposed individuals. Experimentally triggered arousals using auditory stimulation during slow-wave sleep can also be used to successfully induce episodes during sleepwalkers’ normal sleep and even more so during recovery sleep [40]. Diagnosis may thus be substantially aided by such techniques.
Analyses of sleep architecture reveal no significant differences between adult somnambulistic patients and control subjects [42–47], except for a greater number of arousals selectively out of SWS in sleepwalkers, even for nights without sleepwalking episodes [3, 42, 44]. On quantitative analysis of their electroencephalograms (EEGs), sleepwalkers were found to have lower power in slow-wave activity (0.75–4.5 Hz) during the first NREM cycle [44]. They also had a higher number of awakenings during SWS than control subjects (Fig. 50.1) [44].


Fig. 50.1
Slow-wave activity (SWA) over 4 consecutive NREM-REM cycles in 15 sleepwalkers and 15 healthy paired controls. Power is significantly reduced in the second half of the first NREM period. Awakenings from SWS are indicated on the two horizontal lines below the graph (reproduced with permission from Gaudreau et al. [44])
Several studies have documented the presence in the EEG of high-amplitude delta waves, termed hypersynchronous delta (HSD) activity, occurring during SWS or immediately prior to somnambulistic episodes [4, 42, 45, 48]. However, more comprehensive studies have shown that HSD has a low specificity for the diagnosis of somnambulism [47, 49, 50] and that actual episode onset is often not preceded by a gradual accumulation of HSD waves [49]. However, episodes may be preceded by an abrupt change in high-amplitude slow oscillations (<1 Hz) [51], a process that may reflect cortical reactions to brain activation.
With regard to postarousal EEG, Schenck et al. [47] described three patterns that characterized the first 10 s of most SWS arousals in adults with sleepwalking/sleep terrors: pattern I, diffuse rhythmic, and synchronous delta activity (0–4 Hz), most prominent in bilateral anterior regions; pattern II, diffuse and irregular moderate- to high-voltage delta and theta activity intermixed with, or superimposed by, alpha- and beta-activity; and pattern III, prominent alpha- and beta-activity, at times intermixed with moderate-voltage theta activity. A follow-up investigation [52] of adult sleepwalkers showed that patterns II and III were the two more frequently observed forms of postarousal activity and that these patterns were also the only two that occurred during stage 2 episodes.
There is a strong genetic component to somnambulism [18]. About 80 % of somnambulistic patients have at least one family member affected by this parasomnia, and the prevalence of somnambulism is higher in children of parents with a history of sleepwalking [53–55]. A population-based twin study [18] showed a considerable genetic effect in adulthood sleepwalking (proband-wise concordance five times higher in monozygotic than dizygotic pairs), although the effect in childhood sleepwalking was not as pronounced (1.5 times higher in monozygotic than dizygotic pairs). In fact, this parasomnia was recently found to be linked to excessive transmission of the human leukocyte antigen DQB1*05 and *04 alleles [56]. However, the functional significance of this finding remains unclear. More recently, a genomewide scan conducted on a single family composed of 22 members found evidence for a significant linkage at chromosome 20q12-q13.12 [21]. Of particular interest is the fact that the candidate interval includes the adenosine deaminase gene which is believed to play a role in slow-wave sleep [57].
Although daytime somnolence or impairment in daytime functioning has never been part of the clinical portrayal of sleepwalking, there is increasing evidence showing that adult sleepwalkers often complain of excessive daytime somnolence. The association between somnambulism and daytime sleepiness has been found in a large population-based survey [58], in laboratory-based studies based on sleepwalkers’ mean sleep latencies on the Multiple Sleep Latency Test [59] as well as on their scores on the Epworth Sleepiness Scale [60, 61], The EDS reported by adult sleepwalkers does not appear to be explained by the presence of concomitant sleep disorders or PSG signs of nocturnal sleep disruption and may thus be part of the sleepwalking phenotype and linked to its underlying pathophysiology [60].
Associated Factors and Pathophysiology
Although considerable progress has been made in the conceptualization of sleepwalking and its correlates [62], the exact pathophysiologic mechanisms of somnambulism remain unclear. Several factors have been proposed, including psychopathology, genetics, and deregulation of serotonergic systems.
Traditionally, the presence of somnambulism in adulthood has been viewed as a sign of major psychopathology [52, 63, 64]. However, several studies have shown that most adult patients do not have a Diagnostic and Statistical Manual of Mental Disorders-based [1, 65] Axis I psychiatric disorder, nor do they necessarily present with highly disturbed personality traits [46, 66–69]. However, anxiety may increase its occurrence in both children and adults [70, 71]. Based on clinical and research experience, Rosen and colleagues [70] proposed that somnambulism and sleep terrors may be nocturnal expressions of repressed anger concerning major life events such as separation, divorce, marital conflict, or family relocation.
Serotonin has also been hypothesized to be involved in the pathophysiology of sleepwalking on the basis that certain factors implicating the serotonergic system (e.g., certain drugs, fever) can precipitate sleepwalking [72]. In addition, sleepwalking episodes are four to nine times more common in conditions associated with abnormalities in the metabolism of serotonin, such as Tourette’s syndrome or migraine headaches [73–75].
Finally, one single-photon emission computed tomography study was performed during sleepwalking in a 16-year-old boy with a history of somnambulism [76]. It showed that sleepwalking arose from the selective activation of thalamocingulate circuits and the persisting inhibition of other thalamocortical arousal systems. During the episode, the EEG showed diffuse, high-voltage rhythmic delta activity. This supports the notion that sleepwalking is a dissociated state consisting of motor arousal and persisting mind sleep.
Prevalence
The peak incidence of somnambulism (approximately 17 %) is around age 12 years [77]. For adults, a suggested prevalence of 2–2.5 % [78, 79] is probably an underestimate. Although many studies report no gender difference in older children, adolescents, or adults [77, 80], a study of two large cohorts of young children (2.5–6 and 4–9 years old) found it to be more common in boys than in girls [81, 82].
Treatment
Treatment is often unnecessary when the episodes are benign and not associated with potential injury. In this case, reassuring the patient/family about the benign nature of the episodes and demystifying the events is often sufficient. However, identifying and avoiding the potential precipitating factors, such as sleep deprivation, stress, and environmental disturbances, is effective in preventing episodes. Precautions should also be taken to ensure a safe sleep environment.
In children, the preferred treatment for somnambulism consists of a behavioral technique called anticipatory or scheduled awakening [83]. The parents keep a diary of their child’s episodes and determine an average time at which the episodes take place. They then awaken their child about 15–20 min before the typical time of occurrence of the episode for a period of 1 month. It has been reported that the episodes cease as soon as this intervention is started, and the benefit is maintained on long-term follow-up [84, 85]. Hypnosis (including self-hypnosis) has been found to be effective in both children and adults with sleepwalking or sleep terrors [84, 86–91].
Pharmacologic treatment should be considered only if the behaviors are hazardous or extremely disruptive to the bed partner or other household members. Benzodiazepines (clonazepam or diazepam) and tricyclic antidepressants (imipramine) can be effective [92–97]. However, pharmacotherapy does not always result in adequate control of sleepwalking [68]. Treatment should always include instructions on sleep hygiene and stress management.
Sleep Terrors
Clinical Presentation
Sleep terrors (also known as night terrors or pavor nocturnus) are “arousals from SWS accompanied by a cry or piercing scream and autonomic nervous system and behavioral manifestations of intense fear” [2]. The American Academy of Sleep Medicine [2] diagnostic criteria for sleep terrors are presented in Table 50.1. Historically, sleep terrors have been confused with nightmares, a distinct REM sleep parasomnia. In 1965, Gastaut and Broughton [98] first observed by PSG that sleep terrors were not associated with REM sleep but rather occurred suddenly during SWS. Typically, within 90 min after sleep onset, the individual will scream loudly and sit up in bed with a panic-stricken expression. There is usually intense autonomic activity (sweating, flushing of the skin, mydriasis, tachycardia, rapid breathing) and, less often, complex behavioral manifestations such as leaving the bed, fleeing the room, or thrashing around. Injuries may result in such cases. The distinction between sleep terrors and somnambulism is ambiguous, although the activity displayed during sleep terrors is usually more rapid and abrupt than it is during somnambulism [95]. Inconsolability is a key feature of sleep terrors; attempting to console or awaken an individual during an episode will only unduly prolong or intensify it. As is the case for somnambulism (and contrary to a nightmare), the individual usually does not fully wake up and remains amnesic for the event the next day.
As for somnambulism, sudden awakenings from SWS (even without an actual episode starting) are typical of this condition, especially in the second half of the first two SWS sleep episodes. Instability of the NREM EEG pattern was also demonstrated [99]. However, a normal PSG does not rule out a diagnosis of sleep terrors. Time spent in SWS preceding an episode appears to be positively correlated with severity of the episode [95]. Rarely, sleep terrors may arise from stage 2 sleep.
Associated Factors and Pathophysiology
Sleep terrors that occur in childhood are usually not associated with a neurologic condition, whereas onset in adulthood could indicate a neurologic disease. Sleep terrors in adulthood have been described in relation to various psychopathologies, but many studies have shown that such parasomnias can occur in otherwise mentally healthy individuals [3, 46, 100]. As is true for somnambulism, genetic factors play a major role. Monozygotic twins are more concordant than dizygotic twins for sleep terrors [101]. A model-fitting analysis showed that sleep terrors were explained by a two-component model at 18 and at 30 months (about 42–44 % of additive genetic effects and 56–58 % of nonshared environment) [102]. Sleep terrors are twice as frequent in children for whom one or both parents have a sleepwalking history than in children with nonaffected parents [103]. These data and the clinical similarities between these two parasomnias suggest a common genetic predisposition and similar pathophysiologic mechanisms.
As for somnambulism, the incidence of sleep terrors in the sleep laboratory is lower than in the patient’s normal environment [95, 104]. However, sleep terrors can be induced in predisposed individuals by auditory stimulation during SWS [46, 95]. Indeed, in individuals with sleep terrors, the orienting response to auditory stimuli has been reported to be more intense and persistent than in normal subjects, suggesting a hyperexcitability of the nervous system in these individuals [105]. In the sleep laboratory, the severity of the sleep terror, as assessed by heart rate increase and maximum heart rate after arousal, has been found to be proportional to the duration of the preceding SWS episode [106]. The prearousal delta power was also proportional to the sleep terror’s intensity [107]; the EEG preceding sleep terrors contained significantly more delta power in central and frontal regions than control EEG (no event) sections. This was confirmed by Espa et al. [3], who showed that sleep terrors were preceded by an increase of slow-wave activity with the main increase occurring immediately prior to the episode. During the sleep terror itself, the EEG activity demonstrated that the subject was neither fully asleep nor fully awake [95].
Prevalence
Reported incidence estimates are wide ranging [80, 108–110]. For childhood sleep terrors, the estimate is influenced by the age range studied, the sampling method, and definition used. Further, some parents may fail to differentiate between nightmares and sleep terrors. When an operational definition was supplied to parents, a high overall prevalence (40 %) was found for preschool period [82]. More specifically, the prevalence of sleep terrors was found to be 36.9 % at 18 months and 19.7 % at 30 months [102]. As for somnambulism, sleep terrors tend to resolve during adolescence and do not display a gender difference [80, 82]. The prevalence in the general adult population is about 2.2 % and declines gradually with age to attain about 1 % at 65 years of age and older [79]. In adults, there is a high degree of overlap among the two principal disorders of arousal.
Treatment
The scheduled awakening technique was shown to be effective to treat sleep terrors in children [83, 111]. Results of a randomized study in children with sleep terrors indicate satisfactory treatment with l-5-hydroxytryptophan [112]. In adults, when the episodes are not associated with injury potential, treatment is often unnecessary. If a treatment is needed, the same pharmacologic and nonpharmacologic approaches as for somnambulism can be tried.
Parasomnias Associated with REM Sleep
Nightmare Disorder
Clinical Presentation
Nightmare disorder consists of persistent disturbing dreams that arise primarily from REM sleep (and more rarely from stage 2 NREM sleep) and that usually awaken the sleeper [2, 113]. Nightmares are often distinguished from bad dreams precisely because the nightmare triggers an awakening. These awakenings are usually abrupt, not confused, and accompanied by detailed recall of disturbing content. However, there is typically a much lower level of autonomic activation during nightmares than during sleep terrors. Dream-enacting behaviors are not frequent but can occur during transitions to wakefulness [114], e.g., racing heart, perspiration, limb contraction, or bodily start. Nightmares are associated with varying levels of heart rate and respiratory activation during REM sleep, but often the autonomic arousal appears much less than might be expected from the emotions or thematic content of the disturbing dream [115].
Associated Factors and Pathophysiology
Bad dreams among 29-month-old preschoolers are predicted by material ratings of difficult temperament as early as 6 months of age and by parental ratings of child anxiety as early as 17 months [116]. Among adults, nightmares are also associated with demographic factors (e.g., low income) [117], psychopathologic traits [118–120], and personality variables such as physical and emotional reactivity [118, 121, 122], fantasy proneness [123, 124], and thin boundaries [125–128]. Nightmares are more frequent and prevalent in psychiatric populations [129–134] and are associated with pathologic symptoms such as anxiety, neuroticism, post-traumatic stress disorder, borderline symptoms, schizophrenia spectrum symptoms, dissociative phenomena, problematic health behaviors, and sleep disorders (see review by Levin and Nielsen [113]). Several studies converge on the finding that frequent nightmares signal an elevated risk for suicidal and self-harm behaviors [135–138].
Nightmares are also reactive to stressful life events [118, 122, 139–143]. This general pattern of comorbidity among nightmares, pathologic symptoms, and stress has been explained as due to an underlying distress-prone personality style [113]. More frequent nightmares are also associated with the eveningness chronotype [144, 145]. Finally, genetic contributions to nightmares have been suggested by population studies. The estimated proportion of genetic effects to the phenotypic variance in childhood was 44 % for males and 45 % for females in one study [146] and 51 % for all adults combined in another [147].
The few available PSG studies of frequent nightmare sufferers provide inconsistent findings but nonetheless all suggest disruption of REM and NREM sleep regulation. For REM sleep, there is more frequent skipping of early REM periods, longer REM latency and REM/NREM cycle length, lower REM%, and altered REM density across the night—all changes consistent with a possible disruption in REM/NREM cycle timing and duration and low REM sleep propensity in nightmare sufferers [148]. Another study found none of these differences, but did find an increase in REM% [149]. For NREM sleep, reduced NREM sleep duration, more NREM sleep awakenings, increased WASO [149], and anomalies in cyclic alternating pattern (CAP) subtypes (reduced A1, increased A2 and A3) also suggest a disruption of the regulation of sleep-promoting and arousing mechanisms during sleep [150].
A neurocognitive model has been proposed to explain nightmares [151]. The affect network dysfunction model of nightmare production is based on a combination of findings in brain imaging, sleep physiology, post-traumatic stress disorder, fear memory, and anxiety disorders. It complements a neurophysiologic description of nightmare formation, the AMPHAC model, which describes key roles for amygdala, medial prefrontal cortex, hippocampus, and anterior cingulate cortex [113]. Accordingly, nightmares result from dysfunction in a network of affective processes that, during normal dreaming, serves the function of fear memory extinction. Evidence for the involvement of prefrontal and limbic areas in nightmares has been reported [152]; frequent nightmare sufferers have impaired reaction times on Emotional Go/NoGo and Stroop tasks and more perseveration and word generation disturbance on a verbal fluency task.
Prevalence
Prevalence of nightmare disorder is difficult to assess precisely because of different operational definitions, response scales, age ranges, and study samples used (see reviews by Levin and Nielsen [113] and Spoormaker et al. [153]). In addition, prevalence studies usually evaluate nightmares as an isolated symptom but rarely as a disorder per se. The prevalence of nightmare symptoms is estimated in tandem with their temporal frequency. Accordingly, nightmares as a symptom occur occasionally in over 85 % of the general population, at least once a month in 8–29 %, and at least once a week in 2–6 % [78, 121, 129, 154, 155]. There is a consensus (e.g., DSM-5) that a frequency of one nightmare per week reflects clinical pathology.
Surprisingly, bad dreams are not frequent among preschoolers (1.5–3.9 % report them often or always). They can appear as early as 29 months, and the prevalence remains stable until age 6 years [116]. An Internet survey of 23,839 respondents found that the typical monthly recall of nightmares peaks between the ages of 20 and 29 and declines steadily thereafter. There is a gender difference in prevalence favoring girls that appears in adolescence [156, 157] and continues throughout the life span (Fig. 50.2) [158].


Fig. 50.2
Retrospective estimates of monthly nightmare frequency by 5-year age strata in an Internet sample of 23,839 respondents. Asterisk significant difference between female and male subjects at that stratum (p < 0.05) (reproduced from Nielsen and Petit [158])
Treatment
Treatments for nightmares include psychotherapy, systematic desensitization and relaxation, eye movement desensitization, imagery rehearsal, hypnosis, and pharmacological approaches [159–164]. A best practices guide to treatment of nightmares recently proposed by the Standards of Practice Committee of the American Academy of Sleep Medicine recommends only a single Level A drug treatment for nightmares—prazosin—and this only for PTSD nightmares [165]. The same committee also recommends only a single Level A nondrug treatment, Imagery Rehearsal Therapy, for Nightmare Disorder. However, more recent meta-analyses suggest that the recommendations for psychological therapies may need updating. One meta-analysis [166] found empirical support for both psychological and pharmacological (prazosin) treatments. Effect sizes for the psychological treatments (0.47–0.48) were higher than those for drug treatments (0.15–0.29), but equal to the effect size for prazosin alone (0.50). Further, studies of individual therapies had higher effect sizes than did those of self-help therapies. The second meta-analysis [167], examining only nondrug treatments, found that methods of imagery rescripting and rehearsal, which includes IRT, had lower effect sizes than did methods of imaginal confrontation with nightmare contents, such as self-exposure therapy [168].
Recurrent Isolated Sleep Paralysis
Male sex, poor mental health, drinking alcohol, taking a long daytime nap, early or late bedtime, difficulty initiating sleep, low subjective sleep assessment, presence of excessive daytime sleepiness, and presence of nightmares had higher odds ratios than other factors for sleep paralysis [133].
Clinical Presentation
Previously known as isolated sleep paralysis or simply sleep paralysis, recurrent isolated sleep paralysis is a common, generally benign, parasomnia characterized by brief episodes of motor or vocal paralysis combined with a waking state of consciousness [2]. Its benign status is perhaps responsible for its removal from the DSM-5 list of sleep disorders; it is now considered a subcategory of nightmare disorder. During sleep paralysis episodes, fear-provoking dreamlike hallucinations often intrude and produce considerable distress. Episodes occur primarily at sleep onset (hypnagogic) and upon awakening (hypnopompic). Feelings of fear and terror are accompanying sleep paralysis experiences [169], and they are often linked to a feeling of presence (i.e., a vivid impression that a sentient being is nearby, but without a clear visual image of it) [170, 171].
Associated Factors
Stress, shift work, and irregular sleep–wake schedules are factors associated with sleep paralysis episodes [173, 176–178]. Several studies link sleep paralysis to various neurologic and psychiatric disorders. It is predicted by bipolar disorder, automatic behavior, and use of anxiolytic medications [79]. It is also comorbid with post-traumatic stress disorder [179–181], depression symptoms [182, 183], anxiety disorder with agoraphobia [184], panic disorder [181, 185–188], generalized anxiety disorder, anxiety sensitivity [189], social anxiety [190, 191], excessive daytime sleepiness, and poor sleep [192]. This wide comorbidity has been attributed to mediation by an affect distress personality style (“sleep paralysis distress” [171]) in a manner analogous to that proposed for nightmare disorder (“nightmare distress” [113]).
Associations of sleep paralysis with psychiatric conditions vary among ethnic groups [193, 194]. Some of these differences may stem from cultural interpretations of sleep paralysis hallucinations, sensed presence in particular, as a form of spiritual entity, such as “ghost oppression” in China [178], “Old Hag” in Newfoundland [195], “kanashibari” in Japan [176], and “the ghost that pushes you down” in Cambodia [179]. Finally, a genetic component has also been reported: About 36 % of respondents in a Japanese sample had family members who experienced sleep paralysis [196].
Prevalence
Variations in prevalence estimates (5–40 %) depend upon differences in operational definitions, age of subjects, ethnicity, and other sociocultural factors [79, 176, 177]. One meta-analysis [197] of 35 studies (total N = 36,533) found lifetime prevalence rate to be 7.6 % of the general population, 28.3 % of students, and 31.9 % of psychiatric patients, with a slightly higher value for females (18.8 %) than for males (15.7 %). A study, which queried over 90,000 Japanese junior and senior high school students (90 % response rate) about whether or not they had experienced sleep paralysis (“kanashibari”) in the last month, found a prevalence of 8.3 % with prevalence increasing monotonically with age (Fig. 50.3) [133]. Age of onset for sleep paralysis is typically 14–17 years. Accompanying vivid dreams are common (e.g., intruders, vestibulo-motor sensations); sensed presence hallucinations are particularly characteristic, occurring in 60–69 % of cases [170, 171, 198, 199].
Treatment
Snyder and Hams [200] report three cases of isolated sleep paralysis controlled by l-tryptophan.
REM Sleep Behavior Disorder
Clinical Presentation
RBD was first described as a clinical entity in 1986 [201]. It is characterized by the loss of skeletal muscle atonia normally present during REM sleep and accompanied by complex dream-enacting motor activity. Behaviors can range from simple motor activities such as laughing, talking, shouting, or excessive body and limb jerking to complex, seemingly purposeful and goal-directed behavior, such as gesturing, punching, kicking, sitting up, leaping from bed, and running [2]. The close relationship between the dream content and the observed RBD behaviors has been demonstrated in a PSG video study with blind judges [202]. Too often, these sleep behaviors produce injuries to the patient or the bed partner such as ecchymoses, lacerations, fractures, and subdural hematomas. Injuries are a main reason for consultation, being reported by 79–96 % of consulting cases [203]. RBD episodes may also cause severe sleep disruption for the bed partner and lead to major marital discord, mood changes, and even suicide attempts [204]. In addition, the dream process and its content appear altered. Most patients (87 %) report that their dreams became more vivid, intense, action filled, and violent with the onset of RBD [203]. Dream themes associated with behaviors are largely stereotyped in structure and emotional content. The most frequent pattern is that of vigorous defense against attacks by people (58.8 %) and animals (23.5 %) (see review by Nielsen [205]).
It has been observed that the aggressiveness displayed during nocturnal behaviors stands out against the good-natured daytime personality [203]. Indeed, a controlled study using content analyses of recently remembered dreams revealed an elevated proportion of aggressive content, yet normal levels of daytime aggressiveness [206]. The mechanism by which dream content is changed is unclear. However, it has been proposed that hyperactivity (disinhibition through degeneration) at the brain stem level activating motor, perceptual, and affective pathways may be responsible for both REM sleep behaviors and altered dreams [206]. The fact that clonazepam reduces both behavioral manifestations and disturbed dreaming [203, 207] supports the notion that the two phenomena share a common neurophysiologic substrate.
Clinical diagnostic criteria include (1) complaints of violent or injurious behaviors during sleep, (2) limb or body movements associated with dream mentation, and (3) one of the following: harmful or potentially harmful sleep behaviors, dreams that appear to be acted out, and sleep behaviors that disrupt sleep continuity. Polysomnographic recordings reveal an intermittent or complete loss of REM sleep muscle atonia and an excessive phasic electromyographic (EMG) activity during REM sleep [92]. This excessive tonic and phasic EMG activity during REM sleep worsens over time [208]. Diagnostic criteria are listed in Table 50.2. Early studies reported a substantial loss of REM sleep muscle atonia in patients with RBD. For example, three large series reported that 92–100 % of patients had some loss of REM sleep muscle atonia [209–211]. Changes in REM sleep in patients with RBD seem to be restricted to the excessive tonic and phasic motor activity. All other features of REM sleep, including REM latency, REM percentage, REM density, number of REM periods, and REM/NREM cycling, are usually preserved [46, 212].
Table 50.2
ICSD-2 diagnostic criteria for RBD
1. Presence of REM sleep without atonia: electromyographic finding of excessive amounts of sustained or intermittent elevation of submental electromyographic tone or excessive phasic submental or (upper or lower) limb electromyographic twitching 2. At least one the following is present: • Sleep-related injurious, potentially injurious, or disruptive behaviors by history • Abnormal REM sleep behaviors documented during polysomnographic monitoring 3. Absence of electroencephalographic epileptiform activity during REM sleep unless RBD can be clearly distinguished from any concurrent REM-related seizure disorder 4. The sleep disturbance is not better explained by another sleep disorder, medical or neurologic disorder, mental disorder, medication use or substance use disorder |
. |
In contrast, Schenck et al. [210] originally reported an increase in the proportion of SWS in patients with RBD. In one series, 80 % of patients over the age of 50 years had more than 15 % of sleep time spent in SWS. This was not associated with prior sleep deprivation. In the Mayo Clinic series, 33 % of patients over the age of 58 years had more than 15 % SWS [209]. These observations were confirmed in a comparison with age-matched controls: RBD patients demonstrated a higher percentage of SWS and more delta power in NREM sleep [213]. Patients with RBD also showed lower occipital beta power during REM sleep [214], as well as markedly higher theta power in frontal, temporal, and occipital regions, lower occipital beta power, and lower dominant occipital frequency during wakefulness [214].
Another polygraphic characteristic of RBD is the presence of periodic leg movements in sleep (PLMS). In a study of RBD and restless legs syndrome (RLS) patients, RBD patients showed a mean PLMS index of 39.5 per hour of sleep, a value not significantly different from the mean PLMS index found in patients with RLS [215]. In this study, 70 % of RBD patients had a PLMS index greater than 10. This percentage is similar to the prevalence of PLMS previously reported in RLS patients and significantly higher than the prevalence rate found in healthy subjects of the same age [216]. One difference between RLS and RBD patients, however, is that the periodicity of leg movements was shown to be lower in RBD patients compared to that of patients with RLS [217]. Another more important difference is that, in RBD patients, PLMS occurred mainly during REM sleep [215]. This is most likely due to the lack of motor inhibition during REM sleep in this condition, and this suggests that PLMS have a different pathophysiologic basis in RBD. Also, PLMS were significantly less likely to be associated with microarousals in RBD compared to RLS patients [216], and a markedly reduced amplitude of cardiac response was found in patients with RBD [215]. These findings suggest the presence of dysautonomia and/or reduced cortical reactivity in RBD.
Until recently, the diagnosis of RBD was based on clinical manifestations; PSG recordings of patients were not necessary for diagnosis. However, there are some limitations in using clinical criteria only. RBD-like features can occur with other sleep conditions, such as obstructive sleep apnea syndrome, sleepwalking, night terrors, and sleep-related seizures. Therefore, it is important to ensure that behavioral manifestations occur exclusively during REM sleep. In addition, PSG allows the detection of subclinical forms of RBD, such as REM sleep without atonia, which can be observed in the absence of behavioral manifestations. In the ICSD-2 and ICSD-3, PSG features are essential (see also Chap. 27) to the RBD diagnosis [2]. The first essential criterion is the presence of REM sleep without atonia, that is, the EMG finding of excessive amounts of either sustained or intermittent elevation of submental EMG activity or excessive phasic submental or limb EMG twitching. The second criterion is the presence of either sleep-related injurious or disruptive behaviors by history, or abnormal REM sleep behaviors documented during PSG recording. Time-synchronized video recording is essential for helping to establish the diagnosis of RBD during PSG. The last two criteria are the absence of epileptiform activity during REM sleep and the absence of other sleep disorders or medical or neurologic disorders that could better explain the sleep disturbance.
One limitation of these new criteria was the absence of a validated and universally accepted method for scoring REM sleep in RBD. Assessing REM sleep without atonia by using standard criteria is impossible—muscle atonia is an essential defining criterion for REM sleep [218]. Montplaisir et al. [212] developed a scoring method for REM sleep based on the EEG and electro-oculography only (Table 50.3). In this method, the occurrence of the first REM is used to determine the onset of the REM sleep period. The termination of the REM sleep period is identified either by the occurrence of specific EEG features of a different sleep stage (K complexes, sleep spindles, EEG signs of arousals), or by the absence of REMs for 3 consecutive minutes. Then, tonic and phasic components of REM sleep are scored separately. Each epoch is scored as tonic or atonic depending on whether tonic chin EMG activity is present for more or less than 50 % of the epoch duration. Phasic EMG density is also scored from the submental EMG recording and is expressed as the percentage of mini-epochs (2 or 3 s depending on epoch duration of 20 or 30 s) containing phasic EMG events. Using this method, the following criteria have been proposed to diagnose RBD patients: chin EMG tonic density ≥30 % or phasic chin EMG density ≥15 %. Although some night-to-night variability in REM atonia has been shown [219], these cutoffs show a high degree of sensitivity and specificity. A study investigating 11 different muscles showed that upper limb muscles provided the highest discriminative power for RBD diagnosis, even higher than that of the chin EMG [220]. Computer-assisted scoring methods have also been developed (see [221] for a review of the various EMG scoring methods for RBD).
Table 50.3
Polysomnographic scoring criteria for RBD
REM sleep onset | Occurrence of the first REM |
REM sleep termination | Occurrence of a specific EEG feature of another sleep stage (K complex, sleep spindle, EEG sign of arousal) or absence of REMs for 3 consecutive minutes |
Muscle atonia | Each 30-s epoch is scored as tonic or atonic depending on whether chin EMG activity is present for more or less than 50 % of the epoch duration. Presence of EMG activity is defined by chin EMG amplitude at least twice that of the background or greater than 10 μV |
Phasic EMG activity | Percentage of 3-s mini-epochs containing chin EMG events. EMG events are defined by any burst of EMG activity lasting 0.1–5 s, with amplitude exceeding 4 times the amplitude of background EMG activity |
REM density | Percentage of 3-s mini-epochs of REM sleep containing at least one REM |
Associated Factors (Idiopathic and Secondary RBD) and Pathophysiology
RBD may be associated with a great variety of medical conditions or with the use of various psychotropic medications. Therefore, RBD has been divided into primary and secondary forms [1]. Primary or idiopathic RBD is diagnosed when none of the conditions listed for secondary RBD is present. Potential risk factors for idiopathic RBD have nonetheless been identified; they include smoking, head injury, pesticide exposure, and farming [226].
Secondary RBD has been classified into acute and chronic subtypes depending on the time course of clinical manifestations [2]. Acute RBD is usually associated with intoxication or withdrawal from psychotropic substances. Abuse of caffeine, tricyclics antidepressants, biperiden, and monoamine oxidase inhibitors have been reported to trigger episodes of acute RBD as well as withdrawal from alcohol or from medications such as meprobamate, pentazocine, barbiturates, and nitrazepam (for review, see [92]). Chronic secondary RBD may occur during long-term use of several medications: cholinesterase inhibitors, monoamine oxidase inhibitors, tricyclic antidepressants, or newer serotonergic agents such as fluoxetine, paroxetine, citalopram, sertraline, and venlafaxine (for review, see [227]). RBD has been reported in patients with psychiatric disorders, and several indices suggest that use of psychotropic drugs might not be the only cause of the RBD symptoms in this population [228]. Secondary RBD can occur in association with narcolepsy [229, 230], especially narcolepsy with cataplexy and hypocretin deficiency [231], and other neurologic disorders, such as olivopontocerebellar degeneration, ischemic cerebrovascular disease, multiple sclerosis, Guillain–Barré syndrome, Shy–Drager syndrome, and Arnold–Chiari malformation [92]. Finally, RBD is strongly associated with neurodegenerative diseases, especially the synucleinopathy subtype [232–236], which includes Parkinson’s disease (PD), dementia with Lewy bodies (DLB), and multiple system atrophy (MSA). In a sleep laboratory study, one-third of patients with PD (11/33) had RBD based on PSG criteria [232]. Of note, a video-monitored PSG study revealed that patients with PD show a striking improvement of their movements during their RBD episodes (compared to wakefulness) almost as if they were disease-free [237]. RBD has been shown to coexist, albeit to a lesser extent, with Alzheimer’s disease [238] and progressive supranuclear palsy [239], two tauopathies.
Patients with primary (or idiopathic) RBD are, conversely, at high risk of developing a neurodegenerative disease. As more patients with so-called idiopathic RBD are studied in a longitudinal manner, it is becoming increasingly clear that most patients will eventually develop neurodegenerative disorders, especially PD or DLB. Initially, Schenck et al. [233] had found that a parkinsonian syndrome developed in 38 % of 29 patients with idiopathic RBD after 5 years of follow-up. Seven years later, 65 % of patients from the same cohort had developed a parkinsonian syndrome [240]. Now, two prospective studies have estimated that up to 82 % of the patients with iRBD will eventually develop a neurodegenerative disease at a 10 to 16-year follow-up [241, 242]. It was shown that symptoms of RBD can precede the initial manifestation of synucleinopathies by up to 50 years [243]. Since RBD patients are at risk of developing PD, studies have looked at a variety of potential early markers of PD in patients with idiopathic RBD who were free of parkinsonism. Multiple dysfunctions have been described in the last 10 years for idiopathic RBD patients (Fig. 50.4), such as olfactory and color identification deficits [244], decreased motor speed [245], EEG slowing [214], mild dysautonomia [215, 246–250], subtle neuropsychological dysfunctions [214, 251–255], and brain perfusion anomalies [256–258]. These abnormalities are similar to those found in early stages of PD [259] and many are good predictors of conversion to PD with different prodromal period lengths and variable sensitivity and specificity (for review, see [260]). Finally, fluorodeoxyglucose positron emission tomography brain imaging of cognitively normal patients with dream-enacting behaviors revealed lower metabolic activity in several brain regions known to be affected in DLB [261]. In this respect, idiopathic RBD is considered a prodrome of neurodegenerative disease and, consequently, a form of neurodegeneration itself as the following paragraphs on pathophysiology demonstrate.


Fig. 50.4
Electroencephalographic changes and sensory and neuropsychological deficits associated with REM sleep behavior disorder (RBD). a Electroencephalographic slowing during wakefulness is indicated by a generalized increase in the theta/beta2 ratio in male RBD patients (dark blue bars) relative to male controls (black bars), female controls (light blue bars), and female RBD patients (hatched bars). b Visual discrimination deficits are apparent as higher error scores on the Farnsworth-Munsell 100-Hue Test for RBD patients. c Olfactory discrimination deficits are apparent as lower average scores on the University of Pennsylvania Brief Smell Identification Test. d and e Neuropsychological deficits are shown by higher error scores on the Corsi Supraspan Learning Test (d) and lower scores on the Rey-Osterrieth Complex Figure Design (e) (a reproduced with permission from Fantini et al. [214]. b and c reproduced with permission from Postuma et al. [403]. d and e reproduced with permission from Ferini-Strambi et al. [253])
Due to the close association between RBD and PD, the striatal dopaminergic system was investigated as a possible candidate in the pathophysiology of RBD. Single-photon emission computed tomography showed a reduction in striatal dopamine transporters in patients with idiopathic RBD [262]. A reduced density of striatal dopaminergic terminals has also been shown with positron emission tomography [263]. In addition, there appeared to be a continuum of reduction in striatal dopamine transporters on single-photon emission computed tomography from patients with subclinical RBD to clinical RBD and finally to PD [264]. Moreover, a significant correlation was found between the percentage of REM sleep muscle atonia and striatal dopaminergic transmission [264], but not with thalamic cholinergic transmission [265]. However, the dysfunction of the nigrostriatal dopaminergic system is not likely the primary cause of RBD.
Animal studies using various methodological approaches (electrophysiology, lesions, and neuropharmacology) have shown that REM sleep muscle atonia results from the interaction of several neuronal systems located in the brain stem. Bilateral tegmentopontine lesions in animals can produce both a loss of muscle atonia and the presence of motor behaviors during REM sleep [266, 267], a model for human RBD. To produce RBD in animals, two different systems must be involved: the atonia system and the locomotor system. Lesions to the atonia system will produce only REM sleep without atonia, a phenomenon frequently encountered in neurodegenerative diseases and thought to be a form of incomplete RBD. To produce RBD in animals, the lesions should also involve the system that normally suppresses the brain stem motor generators during REM sleep. Therefore, RBD may result from a dysfunction in these two systems: either a loss of REM sleep atonia, excessive locomotor drive, or both [268]. Two different reviews of a large amount of animal physiological data recently proposed that the degeneration of the glutamatergic sublaterodorsal nucleus and of the GABA/glycinergic neurons located in the raphe magnus and gigantocellular reticular nuclei is at the heart of REM sleep without atonia in RBD patients [269, 270].
Neuropathologic and imaging studies of patients with RBD, associated with or without neurodegenerative disorder, also provide answers which are consistent with this hypothesis. Autopsied cases of RBD [66, 235, 241, 271, 272] revealed histopathologic anomalies (i.e., Lewy bodies, neuronal loss, or gliosis) in the locus coeruleus–subcoeruleus complex (the structure homologous to the sublaterodorsal nucleus in humans) and the substantia nigra for all patients and anomalies in the raphe, dorsal vagus, gigantocellular reticular, and pedunculopontine nuclei in most patients. Magnetic resonance imaging revealed ischemic lesions in pontomesencephalic regions in three patients with RBD [273]. Other studies have also demonstrated that tumor, ischemic infarct, or surgery in the pontine region are sufficient to trigger RBD [274–277].
In conclusion, in humans there are probably different anatomical and neurochemical (GABAergic, dopaminergic, cholinergic) dysfunctions that, alone or in combination, could lead to RBD. This could account for the efficacy of various families of medications.
Prevalence
The overall prevalence of RBD remains largely unknown. A large telephone survey assessing violent behaviors during sleep in the general population (15–100 years of age) suggested a prevalence of about 0.5 % [278]. Another study of 1034 individuals (70+ years of age) in the Hong Kong area found a prevalence of PSG-confirmed RBD of 0.4 % [279]. However, these studies screened only for people who suffered sleep injury inclusion of those without injury [280] would likely result in a higher prevalence rate [281]. To that effect, a sleep laboratory study in a population-based sample of 348 individuals 60 years and older found a prevalence of PSG-confirmed RBD of 2.01 % [282]. There is a male predominance (87 %) with primarily men over the age of 50 being affected [174]. When RBD is triggered by antidepressant medications, demographics include younger patients and more women [283]. A certain degree of familial aggregation was recently revealed for RBD by a multicenter study. A positive family history of dream enactment behaviors was found in 13.8 % of iRBD patients compared to only 4.8 % for controls (odds ratio = 3.9, 95 % confidence interval 2.0–7.7) [284].
Treatment
Since 2010, a best practice guide for the treatment of RBD is available [165]. This guide is based on numerous reports of case series of RBD treated with a variety of medications; there are no large randomized double-blind clinical trials. In addition, many of these reports have important methodological limitations and few have included PSG assessments.
Clonazepam, a sedating benzodiazepine, is considered the treatment of choice for RBD. Two large case series have reported substantial improvement in a majority of patients treated with clonazepam [209, 210]. In most cases, a suppression of problematic sleep behaviors and nightmares was reported during the first week of treatment. Sustained efficacy was reported during long-term administration of up to 17 years [92], although some degree of tolerance did occur. Polysomnographic recordings revealed that clonazepam suppresses behavioral manifestations and decreases phasic EMG activity without restoring REM sleep muscle atonia [212]. Clonazepam was also shown to decrease NREM sleep instability in RBD patients [285]. The mechanism of action of clonazepam is unclear but likely results from its serotonergic properties [286]. This hypothesis is based on observations made in animals where selective destruction of brainstem serotonergic neurons produced a disinhibition of REM sleep phasic activity and triggered hallucinatory behaviors, whereas the administration of serotonin inhibited motor activity in several experimental designs [287, 288]. However, clonazepam is ineffective in approximately 10 % of patients [209, 210]. In addition, some patients experience serious side effects, such as an increased risk of confusion and falls in elderly individuals [289] and a worsening of sleep-related respiratory disturbances in patients with obstructive sleep apnea syndrome [290]. Therefore, alternative treatments must be considered.
A 6-week open-label trial of 3 mg of melatonin given 30 min before bedtime demonstrated a dramatic clinical improvement in five of six RBD patients [291]. A PSG recording showed a significant restoration of REM sleep muscle atonia without any significant reduction of phasic motor activity. This initial observation was confirmed in a study of 15 idiopathic RBD patients treated with melatonin 3–9 mg [292]. These authors noted a nearly threefold suppression of REM sleep tonic activity after melatonin therapy. More recently, Boeve et al. [293] looked at the efficacy of melatonin (3–12 mg) in 14 patients with RBD associated with neurologic conditions. RBD was controlled in six patients, significantly improved in four, and initially improved but subsequently returned in two. No improvement occurred in one patient and increased RBD frequency/severity occurred in one patient. Melatonin and clonazepam were recently compared in a clinical practice setting, and it was concluded that both treatments were comparably effective in reducing RBD behaviors and injuries. However, adverse effects were reported less frequently with melatonin than with clonazepam [294]. In summary, melatonin can be considered as an alternative therapy in both idiopathic and secondary RBD, but long-term, controlled trials are needed to ascertain the efficacy of melatonin in this condition. The mechanism of action of melatonin in RBD is still unknown; it appears that melatonin restores REM sleep muscle atonia, whereas clonazepam exerts its therapeutic effect by suppressing phasic motor activity.
Another drug family was shown to produce therapeutic benefit in RBD. Indeed, some studies of acetylcholinesterase inhibitors (donepezil and rivastigmine) demonstrated increased sleep quality and reduced motor events in patients with idiopathic RBD [295] and in patients with RBD associated with DLB [296–298]. However, neither of these studies used PSG recordings to confirm treatment efficacy. Other studies of patients with RBD associated with DLB did not find any change in the frequency or the severity of RBD symptoms with donepezil [293, 298].
Based on the strong association between RBD and PD, dopaminergic agents have been considered as a treatment of RBD. Pramipexole, a dopamine D2 receptor agonist with a high affinity for D3 receptors, was shown to reduce the intensity and the frequency of clinical motor events reported by patients and to decrease the number of simple motor manifestations seen on PSG video recordings [299, 300]. In neither of those studies was REM without atonia reduced. The second study postulated that the improvement in symptoms could rather be due to changes in dream content or dream frequency since the improvement observed correlated with the reduction of REM density. A third study on 98 patients concluded that pramipexole was more effective for mild iRBD cases with a lower rate of REM sleep without atonia [301]. The therapeutic effect of pramipexole was not found in RBD associated with PD [302].
Finally, since injury to the patient or to the bed partner is the most common reason that brings patients to consultation, the treatment program should start with a discussion on risk of accidents, indication of sleeping in different beds and safety measures such as removal of dangerous objects in the room, protection of the windows, and placement of cushions around the bed or the mattress on the floor. These recommendations are important even in treated patients since cases of injuries were reported in patients apparently successfully treated for RBD.
Other Parasomnias
Sleep Enuresis
Clinical Presentation
Sleep enuresis is characterized by recurrent involuntary voiding during sleep at least twice a week among individuals who are at least 5 years of age [2]. If the child has never been constantly dry during sleep, it is considered primary. Sleep enuresis is secondary when the child (or adult) had been previously dry for at least 6 consecutive months and started wetting at least twice a week for at least 3 months.
A common belief among parents is that sleep enuresis is the result of sleeping too deeply. However, changes in sleep depth and sleep architecture have not been consistently demonstrated [303]. For most enuretic children, voiding occurs in the first half of the night and is not associated with a specific sleep stage [303]. Conversely, a PSG study has demonstrated that enuretic boys are more difficult to arouse from sleep than are age-matched controls [304].
Associated Factors and Pathophysiology
A three-system model has been proposed to explain enuresis, which has wide clinical appeal [305]. It identifies three processes that alone or in combination can engender nocturnal enuresis: (1) increased nocturnal urine production due to lack of arginine vasopressin release during sleep; (2) overactivity of the bladder (uninhibited bladder contractions) or low functional bladder capacity; and (3) decreased perception of full bladder sensations during sleep [306]. Tachycardia and short EEG arousals are often present prior to enuretic events [303].
Some evidence indicates that bed-wetting may reflect delayed development of the central nervous system. It has been shown that premature and/or low-birth-weight children were bed-wetting more often than normal-birth-weight children [307, 308]. Moreover, several studies or clinical observations have suggested an association between bed-wetting and developmental delays in motricity, [309, 310] language [310–313], physical growth [311], and skeletal maturation [314, 315]. Microstructure abnormalities were found in the thalamus, medial frontal gyrus, anterior cingulate cortex, and insula of enuretic children [316]. The authors propose that, since these regions are involved in micturition control, a developmental delay in these areas may be the cause of nocturnal enuresis. The full intelligence quotient (IQ), the verbal IQ, and the performance IQ appear to be normal in older enuretic children, but impairments in memory and attention were reported, together with some structural abnormalities of the right dorsolateral prefrontal cortex and left cerebellum [317].
Children with nocturnal enuresis show a higher prevalence of sleep problems, such as disorders of initiating and maintaining sleep, excessive daytime somnolence, sleep-disordered breathing, disorders of arousals, and disorders of the sleep–wake transition [318]. Obesity is a risk factor for nocturnal enuresis in children and adolescents [319]. Enuresis is not linked with anxiety in preschoolers [82] but is in older children [320, 321]. However, anxiety is more likely a consequence than a cause of enuresis, brought about by perplexity or a sense of immaturity, humiliation, social embarrassment, or fear of detection. Similarly, lower scores on self-esteem and higher scores on depression were found in adolescents and adults with nocturnal enuresis [322].
Finally, hereditary factors have been recognized; it is inherited via an autosomal-dominant mode of transmission (for a review, see von Gontard et al. [323]). The odds ratios for severe child nocturnal enuresis were found to be 3.63 times higher in maternal and 1.85 times higher in paternal nocturnal enuresis [324].
Prevalence
In 5-year-old children, population-based studies [82, 312, 325] found a prevalence between 20 and 33 %. A male predominance in childhood prevalence is well established [80, 82, 312, 325]. Prevalence is 77 % when both parents were enuretic as children and 44 % when one parent was enuretic [326]. A Korean population-based study on the prevalence of enuresis in adolescents and adults found that 2.6 % of individuals had at least one episode in the last 6 months and 4.5 % had one in the last 12 months [322]. The percentage of people with at least one episode in the last 6 months was about the same for both genders (men = 2.1 %, women = 3.0 %) and for the different age groups: 16–20 years = 2.8 %, 21–25 years = 2.5 %, 26–30 years = 3.2 %, 31–35 years = 1.8 % and 36–40 = 2.6 %. Enuresis occurs in about 3 % of elderly women (65 years and older) and in 1 % of elderly men living at home [327].
Treatment
Practical guidelines and checklists for the diagnosis and management of enuresis now exist [328, 329]. Most cases of nocturnal enuresis can be treated with bedwetting alarms (devices that alert and sensitize the child to respond quickly to a full bladder by waking up) and by specific treatment programs integrating cognitive-behavioral elements [328, 329]. Concomitant counseling is always recommended. When bed-wetting is caused by lack of arginine vasopressin release during sleep, a pharmacologic intervention using desmopressin, a synthetic analog of vasopressin, can be indicated. The anticholinergic agent oxybutynin is preferred in cases in which the cause is bladder overactivity. Oxybutynin is a smooth muscle relaxant with specific effects on the bladder. Combining treatments is also possible when mixed causes are present (for review, see Butler [330]). In a meta-analysis of randomized trials for the treatment for enuresis (other than with desmopressin or tricyclics), bedwetting alarms were found to be more effective than amphetamine, oxybutynin, and oxybutynin plus holding exercises [331]. The treatment of nonmonosymptomatic nocturnal enuresis is more problematic (for review, see the standardization document from the International Children’s Continence Society [332].
Sleep-related Bruxism
Clinical Presentation
An international consensus [333] was recently reached for the definition and grading of bruxism. The adopted definition is as follows “Bruxism is a repetitive jaw-muscle activity characterized by clenching or grinding of the teeth and/or by bracing or thrusting of the mandible. Bruxism has two distinct circadian manifestations: it can occur during sleep (indicated as sleep bruxism) or during wakefulness (indicated as awake bruxism).” This activity results in tooth wear, headaches, jaw dysfunction, and pain. However, it does not seem to affect occlusal and functional parameters [334].
The international group of experts proposes the following diagnostic grading system for both sleep and awake bruxism: possible (self-report), probable (self-report and clinical examination), and definite (self-report + clinical examination + PSG findings preferably with audio/video monitoring). The definitive diagnosis therefore rests on the presence of rhythmic masticatory muscle activity and grinding sounds during all-night PSG recording. Bruxism episodes most frequently occur in stages 1 and 2 but can occur in all stages [335, 336]. Surprisingly, bruxers have a normal sleep architecture and high sleep efficiency (>90 %) [335]. However, rhythmic masticatory muscle activity is associated with blood pressure surges during sleep [337].
Associated Factors and Pathophysiology
Various hypotheses have been put forward to explain sleep bruxism. The first one was changes in dental occlusion, but no strong evidence-based data support this hypothesis [338]. Orofacial morphology and temporomandibular disorders were shown not to be causal factors [339, 340]. Anxiety has been reported as a strongly associated factor in children [80, 341, 342], adolescents, and adults [343, 344]. Smoking also exacerbates bruxism [345]. A deficiency of the dopaminergic system has been implicated, but that remains to be confirmed since most randomized trials with dopaminergic medications have only marginally reduced sleep bruxism episodes [346, 347]. Finally, a 2013 meta-analysis revealed that bruxism can be triggered by esophageal acidification [348]. It also confirmed that bruxism has an important dose-dependent relationship with smoking and that disturbances in the central dopaminergic system are involved in the etiology of bruxism.
A clear sequence of cortical to cardiac activation preceding jaw motor activity in bruxism patients [349] suggests that sleep bruxism is secondary to microarousals. In fact, both microarousals and rhythmic masticatory muscle activity/sleep bruxism episodes were shown to increase concomitantly just before each REM sleep period [350]. Periodic limb movements during sleep, microarousals, and bruxism often occur concomitantly during sleep and in a time-linked manner [351]. It has been suggested that the sudden apparition of sleep bruxism is under the influence of brief and transient activity of the brainstem arousal–reticular ascending system contributing to the increase of activity in autonomic cardiac and motor modulatory networks (Fig. 50.5) [352].


Fig. 50.5
Sequence of physiological events preceding rhythmic masticatory muscle activity (RMMA) associated with sleep bruxism (reproduced with permission from Lavigne et al. [352])
Finally, as it is the case for many parasomnias, there is a strong genetic influence on bruxism in both childhood and adulthood [353]. Children of sleep bruxers are more likely to be affected than those of individuals who never had the problem or who suffer from daytime bruxism only [354]. Twin studies in bruxism have suggested that genetic factors account for half of the phenotypic variance [355] and are important to both the genesis and pattern of bruxism [356].
Prevalence
Sleep bruxism is common in childhood. However, prevalence estimates reported are variable between the studies due to the different age groups studied (for review on prevalence, see [357]). A longitudinal, population-based study found that the prevalence increases from 2.5 years to reach 33 % at 6 years of age [82]. Another population-based study found prevalence of 36.8 % for preschoolers and 49.6 % for first graders for a frequency of more than once a week [358]. Another longitudinal study reported a progressive decrease from age 10 to 13, attaining 9 % at age 13 [80]. A nationwide Japanese study of adolescents reported a low prevalence of 2.3 % in boys and 3.0 % in girls [359]. A self-reported adult prevalence around 8 % had been found in Canadian [360], European (UK, Italy [361]), Finnish [355], and Japanese [362] populations. Using a representative population of 1042 adults, the overall prevalence in Brazil was found to be 12.5 % if using questionnaires alone, 7.4 % when only PSG was used as diagnosis and 5.5 % when using both questionnaires and confirmation by PSG [363]. Usually, no striking gender difference is found for either children [80, 357] or adults [360]. The presence of sleep bruxism in childhood is highly predictive of bruxism in adulthood [353].
Treatment
A meta-analysis study evaluated randomized controlled studies of seven pharmacologic treatments and three oral devices [364]. The mandibular advancement device and clonidine are the most promising experimental treatments, although both are associated with adverse effects (discomfort for the device; REM suppression and morning hypotension for clonidine). Clonazepam appears to be an acceptable short-term alternative. Occlusal appliances (soft mouth guard, occlusal stabilization splint, splint with vibration) can protect orofacial structures. A PSG study comparing the efficacy of a stabilization splint and gabapentin showed significant reductions in the total duration of bruxism episodes per night and in the number of episodes per hour and per night with both treatments [365]. A recent randomized, double-blind, placebo-controlled, clinical trial reported that hydroxyzine was effective and well tolerated for treating bruxism in children [366]. Finally, the use of biofeedback technology as treatment for bruxism was reviewed and it was concluded that there is no strong evidence of efficacy [367].
Sleep-related Rhythmic Movement Disorder
Clinical Presentation
Sleep-related rhythmic movement disorder is characterized by the repetitive, stereotyped, and rhythmic activity of large muscle groups that occurs predominantly during drowsiness (sleep onset) or sleep [2]. It is largely a parasomnia of infancy and early childhood and usually disappears around age 10 [2]. The most frequent rhythmic movements are body rocking, head rolling, and head banging, although it can involve any body part. Body rocking may be difficult to distinguish from head banging because the former sometimes includes banging of the head into a solid object. The frequency of movements is more typically around 1 Hz (one per second) but can range from 0.5 to 2.0 Hz [368]. Episodes of rhythmic movement can last from a few seconds to more than an hour [368]. In most sufferers, they will occur nightly or almost every night [369]. The majority of episodes (around 80 %), at least for head banging, occur at sleep onset [369]. When appearing at sleep onset, rhythmic movements are considered to be a self-soothing or tension-releasing behavior linked with pleasurable sensations that have hypnotic properties. However, more violent movements, usually in cases of mental retardation, have been reported to cause head or eye injuries [370, 371].
Polysomnographic recordings show that rhythmic movement disorder can arise from REM sleep, NREM sleep, or sleep onset with persisting activity in light sleep [372]. Longer movements are usually observed at sleep onset and during stage 1 sleep, whereas shorter movements are seen in stages 2, 3, and 4 NREM sleep and in REM sleep [368]. Rhythmic movements are not preceded by EEG changes, as are nocturnal seizures, and do not provoke arousals or interrupt SWS even in older children [369, 373].
Associated Factors and Pathophysiology
There are reports of rhythmic movement disorder in association with other sleep-related problems such as RLS, which is associated with body rocking [374, 375], insomnia [376], and RBD [377, 378]. Familial forms are rare but have been reported [372, 376, 379]. Adult cases of rhythmic movement disorder are not usually associated with severe psychiatric disorders as previously believed [372], but a relationship with ADHD has been documented [368, 380]. Finally, some studies have reported daytime complaints such as attentional difficulties, sleepiness, morning headaches, fatigue, and poor concentration—and even more serious problems such as anxiety, depression, hyperactivity, and irritability [368, 372, 381]. Whether or not the daytime symptoms result from poor sleep caused by the rhythmic movements remains to be determined.
The etiology of rhythmic movement disorder is still unknown. The involvement of the central motor pattern generator in the genesis of motor phenomena during sleep has been suggested [382]. This network is involved in the control of early locomotor function [383] and is thought to be under the inhibitory control of the cortex. Immaturity of the inhibitory cortical system in early infancy might account for rhythmic movements occurring during sleep, coinciding with the acquisition of motor milestones.
Prevalence
This parasomnia is quite common in infancy but decreases rapidly in prevalence with increasing age. Incidences of 66 % at 9 months, 26 % at 2 years, and 6 % at 5 years had been reported using a sample of children [384], but an epidemiologic study reported lower incidences of about 6 % at 2.5 years, 3 % at 4 and 5 years, and 2 % at 6 years [82]. Body rocking was found to be present in 3 % of children ages 11–13 years [80]. Rhythmic movement disorder can persist into adulthood [372]. No gender difference has been demonstrated for childhood cases, but there is a male preponderance in adult cases [372].
Treatment
There are no systematic pharmacologic studies or behavioral trials for rhythmic movement disorder; it generally has a benign course and is not usually associated with a severe clinical picture. Benzodiazepines, especially clonazepam, or tricyclic antidepressants can be effective [379, 381, 385]. For rhythmic movement disorder associated with RLS in adulthood, pramipexole seems to be effective in alleviating both conditions simultaneously [375].
Somniloquy
Clinical Presentation
Somniloquy, also known as sleep talking, is defined as talking during sleep “with varying degrees of comprehensibility” [2]. Somniloquy is such a prevalent phenomenon that it is considered to be a normal sleep behavior, especially in childhood.
Somniloquy can arise from all sleep stages [386]. Since there are few systematic PSG studies, no clear profiles have been identified. However, EMG-induced artifact is common and may begin several seconds prior to, and continue several seconds following, verbalizations [387]. Temporary suspension of eye movements and occurrence of sustained alpha-EEG trains during REM sleep somniloquy episodes have also been noted [387], as has suppression of theta- and alpha-activity prior to utterances [388]. Episodes frequently occur in parallel with sleep mentation, but concordance between verbal utterances and ongoing dreamed speech may vary from isomorphic to completely absent [389].
Associated Factors and Pathophysiology
The pathophysiology is unknown. In addition, since somniloquy is so prevalent, it is virtually impossible to isolate predisposing factors. Nonetheless, a clear genetic influence has been demonstrated [390]. Somniloquy is also the parasomnia that most often co-occurs with other parasomnias. It often accompanies the behavioral manifestations of either RBD or somnambulism. Stereotyped vocalizations can also be heard during nocturnal seizures. Recently, somniloquy (especially loud sleep talking) has been found helpful in the differential diagnosis of DLB versus Alzheimer’s disease and other forms of dementia [391]. In most cases, however, somniloquy is idiopathic.
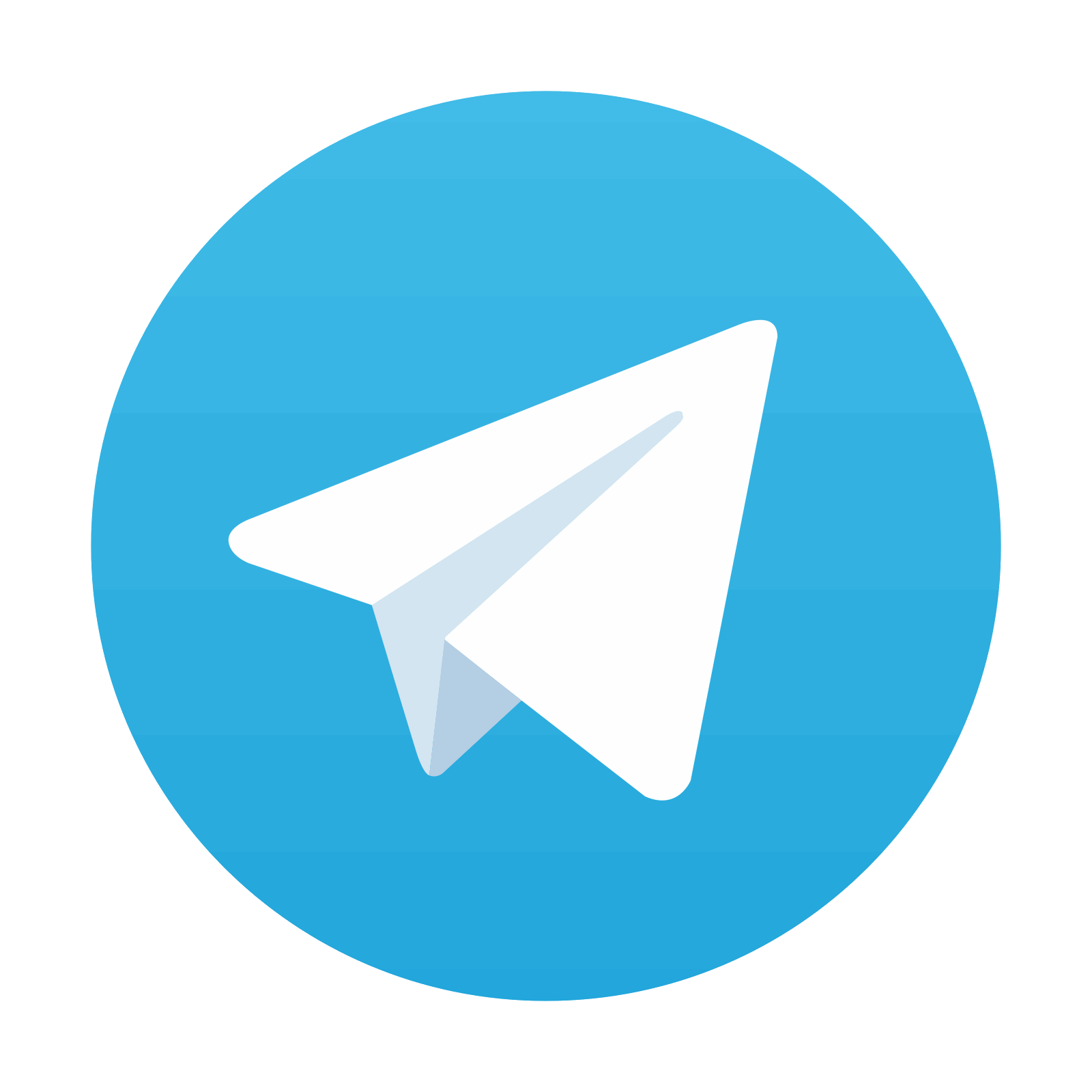
Stay updated, free articles. Join our Telegram channel

Full access? Get Clinical Tree
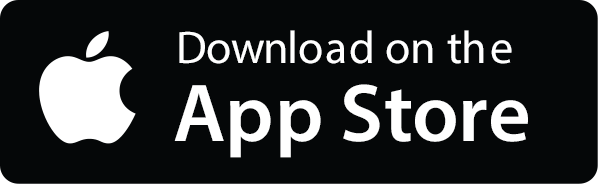
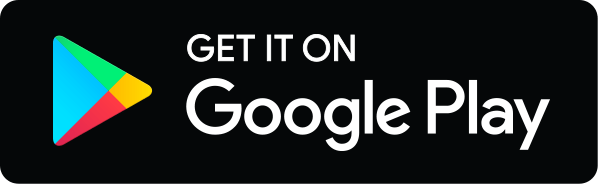