4 Pathological CSF Cell Findings in Intracranial Hemorrhage and Traumatic and Hypoxic-Ischemic Brain Injury H. Kluge, V. Wieczorek, O. W. Witte, E. Linke, K. Zimmermann, M. M. Kluska, S. Isenmann Intracranial hemorrhage and traumatic and hypoxic-ischemic brain injury induce a variety of cellular responses. The major question posed to the cerebrospinal fluid (CSF) cytologist generally concerns the macrophage populations capable of phagocytosis of blood cells that have entered the subarachnoid space or tissue cell fragments that are anomalously present within it. The information of greatest importance to the clinician is whether the CSF contains hematomacrophages, i.e., erythrophages, hemosiderophages, leukophages, and mixed forms. The experienced CSF cytologist will have little difficulty identifying these macrophage populations in the traditional May–Grünwald–Giemsa cytological preparation. These clinically important types of cell are described in this section and illustrated in Figures 4.1–4.37. An understanding of the cellular origin of the various types of macrophage is essential background knowledge for the CSF cytologist, even if it is not the primary object of clinical interest. We will therefore briefly summarize current knowledge in this area. The entry of blood and cellular degeneration products of brain tissue into the CSF spaces as the result of the above-named pathological processes causes, in the initial phase, an acute, nonspecific foreign body reaction. In this reaction, intense leptomeningeal irritation manifests itself as an elevated granulocyte fraction with beginning activation of local monocytes derived from the blood (reactive pleocytosis). Its intensity depends on the severity of the precipitating injury. The disposal (clearing) phase first becomes evident in the CSF about 6–10 hours after the primary event. Activated mononuclear cells begin to appear at greater frequency, either individually or in loose clusters; these cells are predominantly activated monocytes and histiocytes (monocytic origin) but may be activated forms of ependymal/epithelial, or mesenchymal origin (Figs. 4.2–4.9). Their nucleus is rounded, sometimes indented, eccentrically placed, and occasionally hyperchromatic. Their cytoplasm is homogeneous or dispersed depending on the degree of activation. It contains fine acidophilic granules, and it usually has an irregular border with protuberances and pseudopodia. The activated monocytes or histiocytes are occasionally already engaged in erythro- and/or lipophagocytosis at this early stage (Figs. 4.4–4.9). This is most commonly seen in children in the early phase of hemorrhage into the CSF space. Erythrophages are the first type of hematomacrophage to develop from the activated cells of monocytic origin (see Figs. 4.1, 4.10, 4.11). They appear in the CSF about 8–12 hours after the precipitating hemorrhage (in the literature times range from 8 to 24 hours), rapidly increase in number in accordance with the extent and severity of the hemorrhage, and begin the task of the enzymatic breakdown of hemoglobin. Hemosiderin, a product of hemoglobin degradation, appears in the cytological picture about 3–4 days after hemorrhage (2–5 days in the literature). It is visible as brownish-black granules or clumps inside the hematomacrophages, occupying the spaces in between the more or less thoroughly digested erythrocytes, which are often arranged in a gridlike pattern. The macrophages at this stage consist of mixed forms of erythro-/hemosiderophages (see, among others, Figs. 4.12–4.16, 4.18, and 4.20). These mixed forms are also seen in all cases of recurrent hemorrhage. Pure hemosiderophages appear 4–5 days after a single or multiple hemorrhage and can still be found in the CSF for weeks or even months thereafter (Fig. 4.29a–c). Hemosiderin should not be confused with melanin, a pigment associated with melanoma and metastases of melanoma in the CNS, which appears greenish-black with the May–Grünwald–Giemsa stain (see Chapter 5, Melanoma). The distinction is of obvious importance in differential diagnosis. Iron-free hematoidin (identical to bilirubin) is formed in the final stage of hemoglobin breakdown. It is seen as amorphous, brownish-yellow or yellowish crystalline deposits, which can be either intracellular or extracellular after phage autolysis (Figs. 4.25, 4.30, 4.31). Macrophages or hematomacrophages that have phagocytosed not only erythrocytes and/or hemosiderin, but also white blood cells, are called multipotent macrophages (Figs. 4.18, 4.21, 4.22, 4.25, 4.26). Granulocytes, for example, can be seen within the macrophages, either intact or in the form of spherocytes. Hematomacrophages can also phagocytose tumor cells in the CSF (Fig. 4.23). The artifactual admixture of blood in the CSF is a common and often unavoidable result of lumbar puncture (see the neurological literature and Chapter 2, Lumbar puncture artifacts). If the lumbar puncture yields bloody CSF despite the absence of clinical suspicion of subarachnoid hemorrhage, then the physician performing the lumbar puncture should inform the cytology laboratory that this is the case, and perhaps also perform a three-test-tube test to provide further evidence of a “traumatic tap.” Communication between the physician and the laboratory is of the essence (and should be part of routine practice anyway). The cytology laboratory may have considerable difficulty processing the specimen if it is not told of the bloody tap and receives only a single test tube for analysis. It will be in a much better position to establish a diagnosis if informed, for example, that the CSF became progressively less bloody in the second and third tubes. As long as the conditions detailed in Chapter 1 (Proper Handling of CSF Specimens Before Cytological Examination) are met, it still should be possible for the cytological preparation to be carried out within 2 hours of the lumbar puncture. If the ratio of erythrocytes to granulocytes in the cell picture is more or less the same as that in blood, then the occasional finding of erythrocyte aggregates on monocytes cannot be considered proof of hemorrhage (cf. Figs. 4.7, 4.15, 4.16). Various auxiliary CSF tests are often said to be useful for the detection or exclusion of hemorrhage, including measurement of the ferritin level and various spectrophotometric methods based on the different spectral properties of hemoglobin, oxyhemoglobin, methemoglobin, and bilirubin. These tests may provide additional information, but a positive result is not necessarily specific for hemorrhage. We must digress here to comment briefly on the relative diagnostic value of imaging studies and CSF cytological examination in the detection of bleeding into the CSF spaces. It is certainly true that CSF cytological examination, whenever it provides evidence of subarachnoid hemorrhage, still furnishes no information about the source and location of the bleeding (unlike computed tomography (CT) and magnetic resonance imaging (MRI), which might, for example, reveal a ruptured saccular aneurysm). Yet CT and MRI, despite their ever-improving sensitivity, still cannot reveal small amounts of blood in the CSF so well that CSF cytology can simply be dispensed with after a negative imaging study, when the crucial clinical question is, “Subarachnoid hemorrhage, yes or no?” CSF cytological examination is the more sensitive test and should therefore be performed in all cases of suspected subarachnoid hemorrhage when no blood is detected by CT or MRI (as long as there is no contraindication to lumbar puncture, such as anticoagulation, an intracranial mass, or obstructive hydrocephalus). This is especially true later on in the course of subarachnoid hemorrhage, i.e., in the subacute phase and afterward, when blood will have disappeared from the CT image, but cytological examination will still reveal evidence of hemorrhage. Hematomacrophages often additionally exhibit lipophagocytosis, i.e., phagocytosis of lipids derived from cell fragments. The May–Grünwald–Giemsa cell preparation shows phagocytosed lipids as empty (i.e., unstained) vacuoles that are usually considerably smaller than the phagocytosed erythrocytes (cf. Figs. 4.15, 4.21, 4.22, 4.25–4.28, 4.32a, b). Pure lipophages (also known as gitter cells, scavenger cells, or foam cells) can be found in the CSF in traumatic brain injury, cerebral atrophy, and ischemic brain damage, regardless of the presence or absence of accompanying hemorrhage. Their frequency depends on the extent of the injury and its proximity to the CSF spaces. They are often found in very large numbers in ventricular CSF obtained postoperatively from external ventricular drains. They may be as large as hematomacrophages, or even larger. Sometimes the borders between their vacuoles dissolve and the vacuoles join; in the extreme case, a signet-ring form
Stay updated, free articles. Join our Telegram channel

Full access? Get Clinical Tree
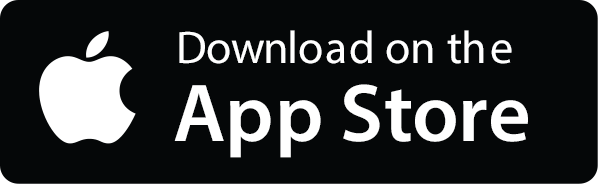
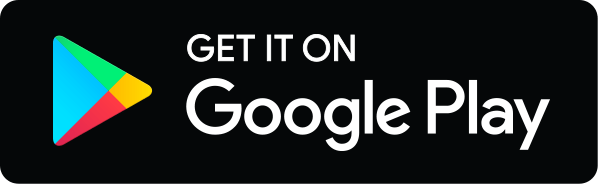