Pediatric Neuroanesthesia Advances in pediatric neurosurgery and anesthetic techniques have dramatically improved the outcome in infants and children afflicted with surgical lesions of the central nervous system (CNS). However, the physiologic and developmental differences inherent in pediatric patients present challenges to neurosurgeons and anesthesiologists alike. The aim of this chapter is to highlight these age-dependent aspects of the perioperative management of the pediatric neurosurgical patient. Age-dependent differences in cerebrovascular physiology and cranial bone development have a significant impact on the perioperative management of neurosurgical patients. Cerebral blood flow is coupled tightly to metabolic demand, and both increase proportionally immediately after birth. Estimates from animal studies place the autoregulatory range of blood pressure in a normal newborn between 20 and 60 mm Hg.1 This is consistent with the relatively low cerebral metabolic requirements and blood pressure during the perinatal period. Importantly, the slope of the autoregulatory curve drops and rises significantly at the lower and upper limits of the curve, respectively (▶ Fig. 82.1). This narrow range, with sudden hypotension and hypertension at either end of the autoregulatory curve, places the neonate, especially when premature, at risk for cerebral ischemia and intraventricular hemorrhage, respectively. Generally, full-term healthy infants have the ability to autoregulate their cerebral circulation, but premature neonates do not.2 Furthermore, high-risk term and premature neonates do not exhibit intact cerebral autoregulation.3 Therefore, tight blood pressure control is essential in the management of neonates to minimize both cerebral ischemia and intraventricular hemorrhage. On studies performed with noninvasive transcranial Doppler technology, the lower limits of cerebral autoregulation appear to be equivalent among older and younger children.4 However, children younger than 2 years old have lower baseline mean arterial pressures; they have less autoregulatory reserve and can theoretically be at greater risk for cerebral ischemia. Another developmental difference between an adult and a pediatric patient is the larger percentage of cardiac output that is directed to the brain in a child because the head of an infant or child accounts for a larger percentage of the body surface area and blood volume (▶ Fig. 82.2), resulting in proportionally greater blood loss and more significant hemodynamic instability in a small child than in an adult. Fig. 82.1 Autoregulation of cerebral blood flow (CBF) in children. The slope of the autoregulatory curve drops and rises significantly at the lower and upper limits of the curve, respectively, and is shifted to the left in neonates and small children. Fig. 82.2 Head size. The head size and surface area are proportionally greater in an infant than in an adult. The infant’s cranial vault is also in a state of development. The open fontanels and cranial sutures result in a compliant intracranial space (▶ Fig. 82.3). The mass effect of a slow-growing tumor or insidious hemorrhage is often masked by a compensatory increase in intracranial volume accompanied by head growth. As a result, infants presenting with signs and symptoms of intracranial hypertension frequently have fairly advanced pathology. However, acute increases in cranial volume due to massive hemorrhage or an obstructed ventricular system will lead to life-threatening intracranial hypertension.5 Fig. 82.3 (a,b) Effect of open cranial sutures and fontanels in neonates and infants. Initially, the compliant skull of the neonate will minimize insidious increases in intracranial volume. However, acute increases in intracranial volume (hemorrhage and obstructed ventriculoperitoneal shunt) will lead to rapid rises in intracranial pressure (ICP). CSF, cerebrospinal fluid. Age-dependent variations also have pharmacologic and physiologic consequences for the perioperative course of neonates and infants. Total body water decreases from 85% in premature infants to 65% in adults, while body fat content increases from less than 1% in premature infants to 15% in term infants and 35% in adults. Total protein follows a similar trend. Therefore, hydrophilic drugs have more binding sites and hydrophobic drugs have fewer binding sites in infancy. The immature renal system is characterized by a decreased glomerular filtration rate and concentrating ability. These changes result in the diminished excretion of saline and water and limit the neonate’s ability to compensate for fluctuations in fluid and solute loads. Furthermore, drugs that are excreted primarily in the urine may have a prolonged half-life. Hepatic function is also diminished in neonates, and the metabolism of drugs may be delayed because of the decreased activity of hepatic enzymes. The constellation of these factors should prompt the clinician generally to decrease the dose and frequency of administration of drugs given to the newborn. Preparation of the pediatric patient for anesthesia and surgery is frequently overlooked in the perioperative period. Given the systemic effects of general anesthesia and the physiologic stress of surgery, an organ system review is essential for anticipating potential physiologic derangements and coexisting disease states that may place the patient at increased risk for perioperative complications.6 General perioperative concerns in infants and children are listed in ▶ Table 82.1. Preoperative laboratory testing should be tailored to the proposed neurosurgical procedure. Given the risk for significant blood loss associated with surgery, a hematocrit in addition to prothrombin time and partial thromboplastin time tests, should be obtained to uncover any insidious hematologic disorder. Serum thyroid hormone levels should be obtained for patients with suprasellar pathology. Type- and cross-matched blood should be ordered before all craniotomies. ▶ Table 82.2 matches special concerns in pediatric patients with specific neurologic problems. Subspecialists in these areas may be needed to help optimize the patient’s condition before surgery and to assist in postoperative management. Closed-claim analysis studies have revealed that neonates and infants are at higher risk for morbidity and mortality than any other age group.7,8 Respiratory- and cardiac-related events account for a majority of these complications. However, no studies indicate any difference in the influence of neurosurgical disease on the morbidity and mortality of pediatric patients. Given the urgent nature of many pediatric neurosurgical procedures, a thorough preoperative evaluation may be difficult. However, a complete airway examination is essential because some craniofacial anomalies may require specialized techniques to secure the airway.9 Most cardiac morbidity due to congenital heart disease occurs during the first year of life. Congenital heart disease may not be apparent immediately after birth. Therefore, echocardiography can be helpful in the assessment of the cardiovascular system, especially in the neonate, and a pediatric cardiologist should evaluate a patient with suspected problems to help optimize cardiac function before surgery. Anesthetic drug–induced neurotoxicity has been the focus of extensive preclinical and clinical research.10 It has been clearly demonstrated in fetal and neonatal laboratory animals that all classes of anesthetic drugs lead to a dose- and duration-dependent increase in neurodegeneration. However, retrospective epidemiologic reports have been inconclusive. Therefore, parents should be reassured that vital surgery cannot be performed without adequate anesthesia, and that the likelihood of the neurodegenerative effects of anesthetic drugs is insignificant in comparison with delaying or withholding anesthesia and surgery. Separation from parents and perioperative anxiety play a significant role in the care of the pediatric neurosurgical patient. These issues are related to the cognitive development and age of the child (▶ Table 82.3). Preoperative sedatives given before the induction of anesthesia can ease the transition from the preoperative holding area to the operating room.11 Midazolam administered orally is particularly effective in relieving anxiety and producing amnesia. If an indwelling intravenous (IV) catheter is in place, midazolam can be slowly titrated to achieve sedation. Preoperative fasting regimens have dramatically evolved over the years and vary according to local preferences.12 The purpose of limiting oral intake is to minimize the risk for pulmonary aspiration of the gastric contents. However, prolonged fasting periods can result in both hypovolemia and hypoglycemia, which, in turn, can lead to hemodynamic and metabolic instability during anesthesia. Although the scientific validity of many recommendations has not been investigated, a common guideline is shown in ▶ Table 82.4. The patient’s neurologic status and coexisting abnormalities will dictate the appropriate technique and drugs for the induction of anesthesia. Often, general anesthesia can be established with the inhalation of sevoflurane and nitrous oxide with oxygen. A nondepolarizing muscle relaxant is then administered after IV access has been established to facilitate intubation of the trachea. Alternatively, if the patient already has an IV catheter, anesthesia can be induced with a sedative–hypnotic drug, such as propofol (3 to 4 mg/kg). These IV agents rapidly induce unconsciousness and can blunt the hemodynamic effects of tracheal intubation. Furthermore, they have minimal effects on cerebrovascular hemodynamics and maintain tight coupling of the cerebral blood flow and cerebral metabolic rate. Patients at risk for aspiration pneumonitis should undergo a rapid-sequence induction of anesthesia with propofol, immediately followed by the administration of a rapidly acting muscle relaxant and the application of cricoid pressure. Rocuronium can be used when succinylcholine is contraindicated, such as for patients with spinal cord injuries or paretic extremities. In these instances, succinylcholine can result in sudden, catastrophic hyperkalemia. Etomidate and ketamine are frequently used to induce anesthesia in hemodynamically compromised patients because these drugs are less likely to cause hypotension than is propofol. However, CNS excitation and increased intracranial pressure (ICP) have been associated with these drugs, respectively, and they may not be appropriate for some neurosurgical patients. Developmental features of the airway anatomy have a significant impact on the management of the pediatric airway. The infant’s larynx is funnel-shaped and narrowest at the level of the cricoid ring. This feature places the infant at risk for subglottic obstruction secondary to mucosal swelling after prolonged endotracheal intubation with a tightly fitting endotracheal tube. Because the trachea is relatively short in the neonate and infant, an endotracheal tube can easily migrate into a main bronchus when the head is flexed, as is done during a suboccipital approach to the posterior fossa or the cervical spine (▶ Fig. 82.4). Therefore, great care should be devoted to ensuring proper positioning of the endotracheal tube during tracheal intubation, and the anesthesiologist should auscultate both lung fields to rule out inadvertent intubation of a main bronchus after final positioning of the patient for the procedure. Nasotracheal tubes are best suited for situations in which the patient will be prone.13 Furthermore, orotracheal tubes can kink at the base of the tongue when the head is flexed, resulting in airway obstruction and direct pressure injury to the tongue. Fig. 82.4 Effect of head flexion and extension on endotracheal tube position. Patient positioning for surgery requires careful preoperative planning to allow both the neurosurgeon and the anesthesiologist adequate access to the patient. ▶ Table 82.5 describes various surgical positions and their physiologic sequelae. The prone position is commonly used for posterior fossa and spinal cord surgery, although the sitting position may be more appropriate for obese patients, who may be difficult to ventilate in the prone position because of increased intra-abdominal pressure (▶ Fig. 82.5). In addition to the physiologic sequelae of this position, a whole spectrum of compression and stretch injuries have been reported. Padding under the chest and pelvis can support the torso. It is important to ensure free abdominal wall motion because increased intra-abdominal pressure can impair ventilation, cause vena caval compression, and increase epidural venous pressure and bleeding. Soft rolls are generally used to elevate and support the lateral chest wall and hips to minimize the increase in abdominal and thoracic pressure. In addition, they allow a Doppler probe to be placed on the chest without causing direct pressure injury. Many neurosurgical procedures are performed with the head slightly elevated to facilitate venous and cerebrospinal fluid (CSF) drainage from the surgical site. However, superior sagittal sinus pressure decreases with increasing head elevation; this increases the likelihood of venous air emboli (VAE).14 Extreme head flexion can cause brainstem compression in patients with posterior fossa pathology, such as mass lesions or a Chiari malformation. However, significant rotation of the head can impede venous return through the jugular veins and lead to impaired cerebral perfusion, increased ICP, and venous bleeding. Fig. 82.5 Sitting position. Because of the limited access to the patient (especially small children) during neurosurgical procedures, ensuring optimal IV access is mandatory before the start of surgery. Typically, two large-bore venous cannulas are sufficient for most craniotomies. Should initial attempts fail, central venous cannulation may be necessary. Use of the femoral vein avoids the risk for pneumothorax associated with subclavian catheters and does not interfere with cerebral venous return, as may be the case with jugular catheters. Furthermore, femoral catheters are more easily accessible to the anesthesiologist during operations on the head. Because significant blood loss and hemodynamic instability can occur during craniotomies, cannulation of the radial artery provides direct blood pressure monitoring and sampling for blood gas analysis. Other useful arterial sites in infants and children include the dorsalis pedis artery and posterior tibial artery. Several classes of drugs are used to maintain general anesthesia. Each has distinct characteristics that make it potentially appropriate for neurosurgery. Potent, volatile anesthetic agents (i.e., sevoflurane, isoflurane, and desflurane) are administered by inhalation. Sevoflurane has virtually replaced halothane as the principal agent for the induction of anesthesia in infants and children.15 It rapidly induces anesthesia and minimally depresses the cardiovascular system.16 Changing the inspired concentration of the drug can rapidly alter anesthetic depth. These inhaled drugs are potent cerebrovascular dilators and cerebral metabolic depressants, two qualities that can lead to a dose-dependent uncoupling of cerebral metabolic supply and demand and to increased cerebral blood volume and ICP. Moreover, their use can be associated with a significant decrease in cerebral perfusion pressure that is due primarily to a dose-dependent reduction in arterial blood pressure.17 Given these issues, volatile anesthetics are rarely used as the sole anesthetic for neurosurgery. IV anesthetics are categorized as sedative–hypnotic or opioid. These drugs are also potent cerebral metabolic depressants but do not cause cerebral vasodilation. Therefore, they are closer to “ideal” anesthetics. Fentanyl is the opioid most commonly used in this setting. The context-sensitive half-life of fentanyl and sufentanil increase with repeated dosing or prolonged infusion, and the drugs require hepatic metabolism, which is immature in neonates. As a result, their narcotic effects, such as respiratory depression and sedation, may be prolonged. Remifentanil is a unique opioid that is rapidly cleared by plasma esterases. Therefore, when administered at a rate of 0.2 to 1.0 μg/kg per minute, it is an ideal opioid for rapid emergence from anesthesia.18,19 However, this rapid recovery is frequently accompanied by delirium and inadequate analgesia.20,21 Nevertheless, the choice of agents for the maintenance of anesthesia has been shown not to affect the outcome of neurosurgical procedures when they are properly administered.22 The most frequently used anesthetic technique during neurosurgery consists of an opioid (fentanyl or remifentanil) along with inhaled nitrous oxide (70%) and low-dose (0.2 to 0.5%) isoflurane. The incidence of awareness under anesthesia has been reported to be 0.8%, a value higher than that in adults.23 Processed electroencephalographic algorithms have been advocated to measure the depth of anesthesia. However, their utility in children younger than 3 years is still in question.24 Deep neuromuscular blockade with a nondepolarizing muscle relaxant is maintained to avoid patient movement and to minimize the amount of anesthetic agents needed. Patients receiving chronic anticonvulsant therapy will require larger doses of muscle relaxants and narcotics because of the induced enzymatic metabolism of these agents25 (▶ Fig. 82.6). Muscle relaxants should be withheld or their effects permitted to wear off when an assessment of motor function during neurosurgery is planned. Fig. 82.6 Effect of chronic anticonvulsant therapy on the half-life of the muscle relaxant vecuronium. Vecuronium plasma concentrations are plotted against time after a single bolus dose of vecuronium (0.15 mg•kg-1). Mean ± standard deviation is plotted for all three groups. Hemodynamic stability during intracranial surgery requires the careful maintenance of intravascular volume and electrolytes. Preoperative fluid restriction and/or diuretic therapy may lead to blood pressure instability and even cardiovascular collapse if sudden blood loss occurs during surgery. Therefore, normovolemia should be maintained throughout the procedure. Estimation of the patient’s blood volume is essential in determining the amount of allowable blood loss and when blood will need to be transfused. Blood volume depends on the age and size of the patient, as delineated in ▶ Table 82.6. Normal saline is commonly used as the maintenance fluid during neurosurgery because it is mildly hyperosmolar (308 mOsm/kg) and should minimize cerebral edema. However, rapid infusion of large quantities of normal saline (> 60 mL/kg) can be associated with hyperchloremic acidosis.26 Given the relatively large blood volume of the neonate and infant, the maintenance rate of fluid administration depends on the weight of the patient (▶ Table 82.7). Because significant blood loss is likely to occur during most craniotomies in infants and children, the maximum allowable blood loss should be determined in advance to know when blood should be transfused. However, there are no guidelines regarding the threshold for transfusing blood, and the decision to transfuse should be dictated by the type of surgery, the underlying medical condition of the patient, and the potential for additional blood loss both intra- and postoperatively. Hematocrits of 21 to 25% should increase consideration for blood transfusion. Packed red blood cells (10 mL/kg) will increase the hematocrit by 10%. Initially, lost blood should be replaced with 3 mL of normal saline for each 1 mL of blood lost or with a volume of colloid solution, such as 5% albumin, equal to the volume of blood lost. Depending on the extent and length of the surgical procedure and the exposure of vascular beds, additional fluid administration at 3 to 10 mL/kg per hour may be necessary. Because gluconeogenesis in neonates is underdeveloped, it may be necessary to add some glucose to the maintenance IV fluids for those who were receiving glucose infusions in the preoperative period. However, surgery elicits a stress response, and children are generally able to maintain normal serum glucose levels without exogenous glucose administration.27 In any case, hyperglycemia is always best avoided because it may exacerbate neurologic injury if ischemia occurs. Brain swelling can be initially managed with hyperventilation and elevation of the head above the heart. Should these maneuvers fail, mannitol can be given IV at a dose of 0.25 to 1.0 g/kg. This will transiently alter the cerebral hemodynamics and raise the serum osmolality by 10 to 20 mOsm/kg.28 However, repeated dosing can lead to extreme hyperosmolality, renal failure, and brain edema.29 Furosemide is a useful adjunct to mannitol in decreasing acute cerebral edema and has been shown in vitro to prevent rebound swelling due to mannitol.29,30 All diuretics will interfere with the ability to use the urine output as a guide to the intravascular volume status. Patients undergoing major craniotomies and spine surgery are at risk for sudden hemodynamic instability due to hemorrhage, VAE, herniation syndromes, and/or manipulation of the cranial nerves. The potential for cerebral hypoperfusion generally warrants placement of an arterial cannula for continuous blood pressure monitoring and access for sampling serial blood gases, electrolytes, glucose levels, and hematocrit. Although it may initially appear counterintuitive, small patient size decreases the threshold for inserting an arterial catheter because small children cannot tolerate as much blood loss as adults can. The utility of central venous catheterization remains controversial. In adults, cannulation of the jugular or subclavian veins with catheters that have multiple orifices is often preferred, particularly when VAE are anticipated. However, these catheters are too large for infants and most small children. Furthermore, the central venous pressure may not accurately reflect intravascular volume in small children, particularly when they are in the prone position. Therefore, the risks of a central venous catheter may outweigh the benefits. In infants, even when VAE occur, the use of a central venous catheter for aspirating air is not frequently successful, presumably because of the high resistance of the small-gauge catheters placed in these patients.31 VAE have been detected during many craniotomies in infants and children, primarily because the head of a small child is large in relation to the rest of body and rests above the heart when the child is in either the prone or supine position (▶ Fig. 82.7).32,33 Standard neurosurgical techniques may elevate the patient’s head even further by raising the head of the table to improve cerebral venous drainage. This maneuver can increase the risk for air entrainment into the venous system through open venous channels in bone and sinuses.14 Patients with cardiac defects and the potential for right-to-left shunting, such as those with a patent foramen ovale or patent ductus arteriosus, are at risk for paradoxical air emboli leading to potential cerebral or myocardial infarction. A precordial Doppler ultrasound device can detect minute VAE and should be routinely used in conjunction with an end-tidal carbon dioxide analyzer and arterial catheter in all craniotomies to detect VAE early, before significant hemodynamic instability develops. The Doppler probe is best positioned on the anterior chest, usually just over or to the right of the sternum at the fourth intercostal space (i.e., the nipple line). An alternate site on the posterior thorax can be used in infants weighing approximately 6 kg or less who are in the prone position.34 In addition to the characteristic changes in Doppler sounds, sudden decreases in end-tidal CO2, dysrhythmias, and/or ischemic changes in the electrocardiogram can occur with VAE. Fig. 82.7 (a) Supine and (b) prone infant. Note that the infant’s head lies at a higher plane than the rest of his body. This increases the likelihood for venous air embolism during craniotomies. Recent advances in neurophysiologic monitoring have enhanced the ability to perform more definitive neurosurgical resections safely in functional areas of the brain and spinal cord. However, the depressant effects of many anesthetic agents limit the utility of these monitors. A major part of preoperative planning should include a thorough discussion of the modality and type of neurophysiologic monitoring to be used during any surgical procedure. In general, electrocorticography (ECoG) and electroencephalography (EEG) can be used when levels of volatile anesthetics are low. Somatosensory evoked potentials (SSEPs), used during spinal and brainstem surgery, can also be depressed by high levels of volatile agents and, to a lesser extent, nitrous oxide. An opioid-based anesthetic is the most appropriate agent for this type of monitoring. Spinal cord and peripheral nerve surgery may require electromyography (EMG) and the detection of muscle movement as an end point. Therefore, muscle relaxation should be either avoided or permitted to dissipate during the monitoring period. ▶ Table 82.8 lists common anesthetic agents and their effects on various neurophysiologic monitors. The primary cause of cerebral ischemia in infants and children is cerebral hypoperfusion secondary to systemic arterial hypotension, especially when it is accompanied by intracranial hypertension. The three potential clinical intraoperative modalities for monitoring cerebral ischemia are (1) EEG, (2) transcranial Doppler, and (3) near-infrared spectroscopy. However, during the routine use of these monitoring modalities, technical difficulties with positioning and recording are frequently encountered because of the proximity of the surgery. Clinical studies have yet to demonstrate any clinical improvement with their use in the intraoperative setting. Recent advances in neurophysiologic monitoring have enhanced the ability to perform more definitive neurosurgical resections safely in functional areas of the brain and spinal cord. However, the depressant effects of many anesthetic agents limit the utility of these monitors. A major part of preoperative planning should include a thorough discussion of the modality and type of neurophysiologic monitoring to be used during the surgical procedure. In ECoG, grid and strip electrodes are placed on the surface of the brain following dural opening. Because some epileptogenic foci are in close proximity to cortical areas controlling speech, memory, and motor or sensory function, monitoring of the patient’s electrophysiologic responses is frequently used to minimize iatrogenic injury to these areas.35–37 The detection of cortical stimulation of the motor strip in a child under general anesthesia will require either EMG or the direct visualization of muscle movement. Neuromuscular blockade should not be used in this situation. Cortical stimulation is possible with a dual-channel stimulator. Epileptogenic activity may be evidenced by clearly documented electrographic seizures or EEG spike activity, which consists of either interictal spikes of 50 to 80 milliseconds or sharp waves of 80 to 200 milliseconds. During anesthesia, the use of low concentrations of volatile anesthetics or nitrous oxide and opioids alone should avoid the attenuation of ECoG and EEG signals. Neural function is best assessed in an awake and cooperative patient.38 Positioning of the patient is critical for the success of this technique. A semilateral position allows both patient comfort and surgical and airway access to the patient (▶ Fig. 82.8). Motor and sensory regions of the cortex are localized by inducing motor movements or sensory changes with cortical stimulation. Language function is tested by eliciting speech arrest with cortical stimulation. Verbal memory is tested by stimulating the hippocampus or lateral temporal cortex. In a series of children undergoing awake craniotomy, in which local anesthesia and propofol and fentanyl or dexmedetomidine were used for sedation and analgesia during the resection of eloquent areas of the brain, we found that propofol, when discontinued 20 minutes before monitoring, did not interfere with ECoG, and cooperative children older than 10 years were able to withstand the procedure without incident.39 However, it is imperative that candidates for an awake craniotomy be mature and psychologically prepared to participate in this procedure. Therefore, an awake craniotomy should not be considered appropriate for patients who are developmentally delayed or have a history of severe anxiety or psychiatric disorders. Very young patients cannot be expected to cooperate during these procedures and usually require general anesthesia with extensive neurophysiologic monitoring to minimize inadvertent resection of the eloquent cortex. Fig. 82.8 Positioning the patient for an awake craniotomy. Note that there is clear access to the patient to facilitate neuropsychological testing.
82.1 Developmental Considerations
82.2 Preoperative Evaluation and Preparation
Condition
Anesthetic implications
Congenital heart disease
Hypoxia, arrhythmias, and cardiovascular instability. Paradoxical air emboli
Prematurity
Postoperative apnea
Gastrointestinal reflux
Aspiration pneumonia
Upper respiratory tract infection
Laryngospasm, bronchospasm, hypoxia, pneumonia
Craniofacial abnormality
Difficulty with airway management
Condition
Anesthetic implications
Denervation injuries
Hyperkalemia after succinylcholine Resistance to nondepolarizing muscle relaxants Abnormal response to nerve stimulation
Chronic anticonvulsant therapy
Hepatic and hematologic abnormalities Increased metabolism of anesthetic agents
Arteriovenous malformation
Potential congestive heart failure
Neuromuscular disease
Malignant hyperthermia Respiratory failure Sudden cardiac death
Ketogenic diet and/or topiramate therapy
Chronic metabolic acidosis
Chiari malformation
Apnea Aspiration pneumonia
Hypothalamic/pituitary lesions
Diabetes insipidus Hypothyroidism Adrenal insufficiency
Age group
Concerns
Infants (0 to 9 months)
None, will separate easily from parents
Preschoolers (9 months to 5 years)
Stranger anxiety, difficulty with parental separation
Grade-schoolers (6 to 12 years)
Fear of needles and pain
Adolescents (> 12 years)
Anxiety about surgery and self-image
Fasting time (h)
Oral intake
2
Clear liquids
4
Breast milk
6
Formula
8
Solid food
82.3 Intraoperative Management
82.3.1 Induction of Anesthesia
82.3.2 Airway Management
82.3.3 Positioning
Position
Physiologic effect
Head elevated
Enhanced cerebral venous drainage Decreased cerebral blood flow Increased venous pooling in lower extremities Postural hypotension
Head down
Increased cerebral venous and intracranial pressure Decreased functional residual capacity (lung function) Decreased lung compliance
Prone
Venous congestion of face, tongue, and neck Decreased lung compliance Increased abdominal pressure possibly leading to vena caval compression
Lateral decubitus
Decreased compliance of downside lung
82.3.4 Vascular Access
82.3.5 Maintenance of Anesthesia
82.3.6 Intraoperative Fluid and Electrolyte Management
Age
Estimated blood volume (mL/kg)
Preterm neonates
100
Full-term neonates
90
Children 1 year or younger
80
Children 1 to 12 years
75
Adolescents and adults
70
Weight (kg)
Rate
≤ 10
4 mL/kg/h
10–20
40 mL + 2 mL/kg/h for every kg > 10 kg
≥ 20
60 mL + 1 mL/kg/h for every kg > 20 kg
82.3.7 Monitoring
Neurophysiologic Monitoring
MAP
CBF
CPP
ICP
CMRO2
SSEP/MEP amplitude
SSEP/MEP latency
Inhaled agents
Halothane
↓↓
↑↑↑
↑↑
↑↑
↓↓
↓
↑
Isoflurane
↓↓
↑
↑↑
↑
↓↓↓
↓
↑
Sevoflurane
↓↓
↑
↑
Ø – ↑
↓↓↓
↓
↑
Desflurane
↓↓
↑
↑
↑
↓
↓
↑
Nitrous oxide
Ø – ↓
↑ – ↑↑
↓
↑ – ↑↑
↓↑
↓
Ø – ↑
IV agents
Thiopental
↓↓
↓↓↓
↑↑↑
↓↓↓
↓↓↓
↓
↑
Propofol
↓↓↓
↓↓↓
↑↑
↓↓
↓↓↓
↑
↑
Etomidate
Ø – ↓
↓↓↓
↑↑
↓↓↓
↓↓↓
↑
↑
Ketamine
↑↑
↑↑↑
↓
↑↑↑
↑
↑
Ø
Midazolam
Ø – ↓
↓↓
↑
Ø
↓↓
↓
Ø – ↑
Fentanyl
Ø – ↓
↓
↓↑
Ø – ↓
↓
↓
↑
Dexmedetomidine
Ø –↑
↓
↓↑
Ø – ↓
↓
↓
↑
Abbreviations: CBF, cerebral blood flow; CMRO2, cerebral metabolic rate of oxygen; CPP, cerebral perfusion pressure; ICP, intracranial pressure; MAP, mean arterial pressure; MEP, motor evoked potential; SSEP, somatosensory evoked potential.
Monitoring of Cerebral Oxygenation
Detection of Seizure Foci
Monitoring in Awake Craniotomy
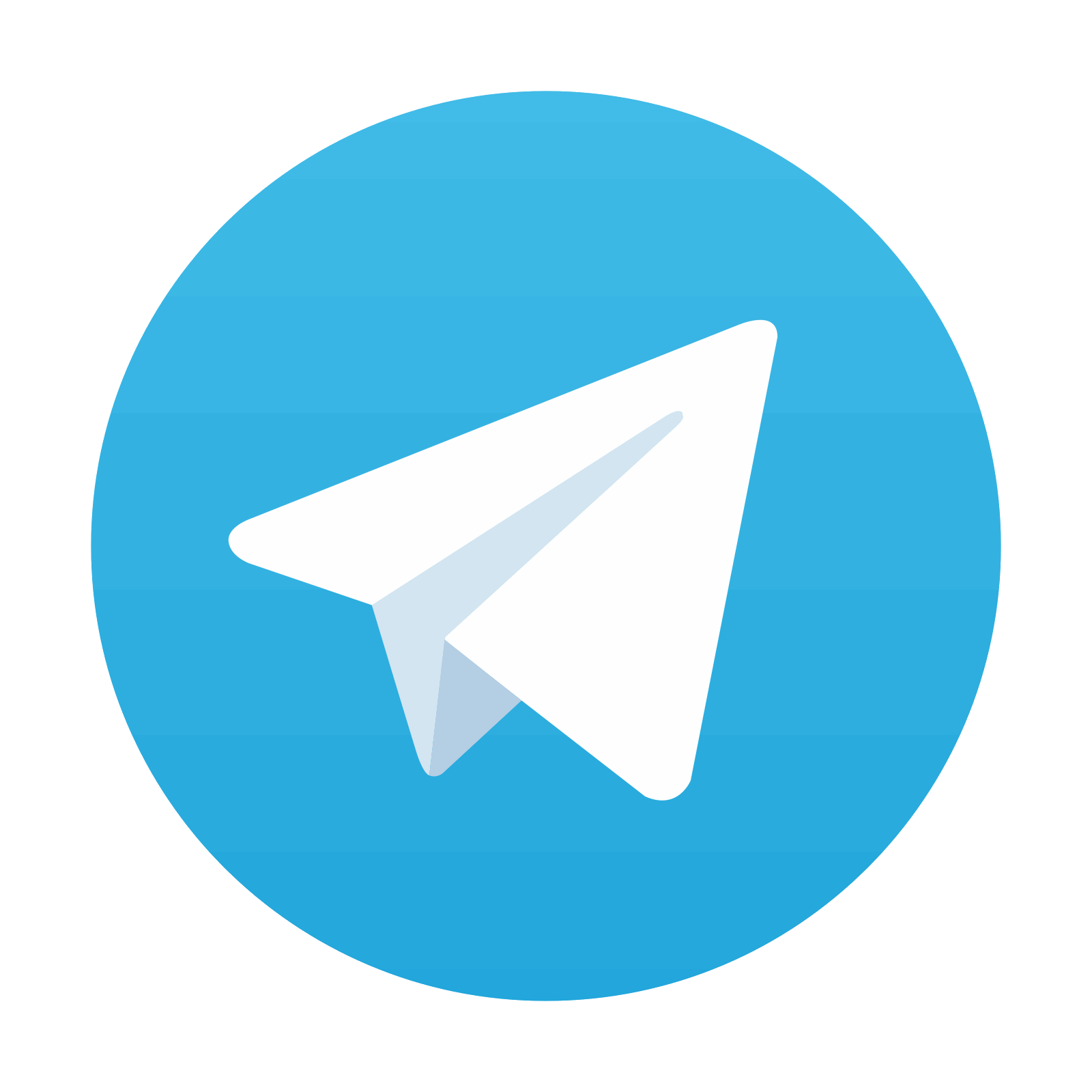