CHAPTER 105 PERIPHERAL NERVE INJURY
Peripheral nerve trauma is any injury to a nerve caused by an extrinsic agent; such injuries constitute a distinct category of peripheral nerve disorders. Peripheral nerve trauma is a commonly encountered problem in level I trauma centers after severe trauma, and residual manifestations of nerve dysfunction are a common reason for referral to a neuromuscular practice. In one population-based study, injury to the peripheral nerves was responsible for 2% of hospital admissions and 29% of surgical procedures after trauma.1 In the majority of cases, nerve dysfunction is the result of penetrating, stretch, or crush injury from violent accidents, although a variety of other extrinsic agents may also produce nerve injury. Appropriate early identification and evaluation of nerve trauma is important in guiding the treatment and the attempts to preserve or improve function of the nerve. The clinical features, pathophysiology, evaluation, and treatment of physical nerve injury are discussed in this chapter.
ANATOMY AND PHYSIOLOGY OF PERIPHERAL NERVE INJURY
The foundation for understanding nerve injury begins with a brief discussion of nerve anatomy and physiology. This topic and its relation to nerve injury have been reviewed extensively.2,3 Through the classic work of Sunderland (1990), the details of the microstructure of nerve anatomy have been elucidated and have formed the foundation for the development of classification schemata of nerve injury.2 The peripheral nerve is composed of several components, each of which plays an integral role in nerve function (Fig. 105-1). The cell body, or cyton, is the metabolic center of the nerve and produces the structural elements and nutrients that are important for maintaining nerve function. The axon is the main transporting segment of the nerve and conducts action potentials and transports proteins from the cell body to the nerve terminal. Most nerves contain a myelin sheath that surrounds the axon and provides a mechanism for rapid conduction of the action potential along the axon.
The primary function of a nerve is to conduct information from one site to another in the nervous system. In the peripheral nervous system, the motor nerves conduct impulses from the brainstem or spinal cord to a muscle, whereas the sensory nerves conduct sensory stimulus from a peripheral receptor back to the spinal cord or brainstem. An inability to conduct these impulses produces weakness, sensory loss or disturbance, or autonomic dysfunction. The physiology of propagation of these impulses begins with an action potential generated at the cell body of the motor neuron or peripheral sensory receptor. The action potential then rapidly spreads from the point of origin over the entire axon membrane to the nerve terminal at the neuromuscular junction, in the case of a motor axon, or to the central nervous system, in the case of a sensory axon. Rapid propagation of the action potential along the nerve relies on the integrity of the axon, the transmembrane channels, and the myelin sheath. When nerve injury occurs, interference with action potential development and propagation may occur, leading to neurological symptoms.
CLASSIFICATION OF PERIPHERAL NERVE INJURY
Different classifications of stages of nerve injury have been developed.2,4 One scheme, proposed by Sunderland (1990), separates nerve injury into five stages on the basis of the extent of anatomical disruption of the nerve (Table 105-1).2 In this classification, the higher stages of nerve injury represent a greater degree of damage to the nerve and the surrounding structures, and therefore the potential for nerve regeneration and regrowth diminishes, leading to a poorer prognosis. The other classification scheme, developed by Seddon, consists of fewer stages that also reflect the degree of damage to the different components of the nerve and supporting structures. Seddon proposed three stages of nerve injury: neurapraxia, axonotmesis, and neurotmesis.4
Axonotmesis in Seddon’s classification scheme is a more severe stage of injury. In this stage, the continuity of the axon is disrupted and the portion of the axon separated from the anterior horn cell or dorsal root ganglia undergoes wallerian degeneration. For the first week after an axonotmetic injury, the distal portion of the axon that is separated from the cell body still has the ability to propagate an action potential when stimulated electrically. After 1 week, wallerian degeneration of the axon occurs, and the disconnected segment of the axon can no longer conduct an action potential when stimulated. Axonal regeneration and regrowth along the endoneurial tubes is possible. Sunderland divided axonotmetic lesions into three separate degrees, on the basis of the amount of damage to the connective tissue. In Sunderland’s stage 2 injuries, the axon is disrupted but the endoneurial tube, and remainder of the connective tissue is intact. In stage 3, the axon and endoneurial tube are disrupted, but the perineurium and epineurium are intact. In stage 4 lesions, the axon, endoneurial tube, and perineurium are damaged, and only the epineurium is preserved. The duration and extent of axonal regrowth and regeneration depend on the degree of disruption of the nerve and the distance required for the nerve to regrow. The amount and speed of recovery are worse with higher grades of injury in Sunderland’s classification.
EVALUATION OF NERVE TRAUMA
Electrophysiological Evaluation
Motor NCS are often more useful than sensory NCS in identifying the degree and stage of nerve injury. In a normal nerve, supramaximal electrical stimulation of a nerve depolarizes all of the motor axons within the nerve, which subsequently produce action potentials within all muscle fibers innervated by that nerve. This produces a summated compound muscle action potential (CMAP), or M wave, recorded from a muscle innervated by the nerve. When the nerve is stimulated at a proximal and distal site, the CMAP amplitudes and areas of the two sites are similar, inasmuch as all axons within the nerve are similar in size and conduct at a similar rate. In most motor nerves, there is never more than a 20% reduction in amplitude and area between the two sites of stimulation (Fig. 105-2).
In nerve trauma characterized by neurapraxia or early axonotmesis, the evoked action potential initiated at a site proximal to the injury is unable to propagate through the lesion. Thus, the lesion produces a “conduction block” of the action potential. However, because the axonal segment and supporting structures distal to the injury remain intact, stimulation distal to the lesion produces a normal response. In the most severe cases in which all axons within a nerve are affected, such as with complete nerve transection or a neurapractic lesion involving all motor axons, no response is obtained with proximal stimulation (complete conduction block) (Fig. 105-3). Stimulation distal to the site of nerve injury produces a propagated action potential and a normal CMAP. In cases of incomplete nerve injury, only some axons are affected, and a partial conduction block may occur (Fig. 105-4). Stimulation proximal to the lesion produces action potentials in all the motor axons. Some percentage of these action potentials are propagated by motor axons that are damaged, and these action potentials do not spread through the lesion. There, action potential spread is blocked. Other motor axons in the nerve may be spared by the lesion, and these spread across the lesion to reach the muscle and produce a CMAP. The amplitude and area of the CMAP are a rough guide to the number of axons spared in an acute to subacute lesion. In more superficial nerves, or with near-nerve stimulation with a monopolar needle, the precise site of the nerve injury can be localized to within 2 cm by short-segment incremental stimulation (“inching”) studies.
In neurapractic lesions, the conduction block persists until the underlying process is reversed. However, in lesions characterized by axonotmesis or neurotmesis, subsequent events produce further changes on NCS. Within the first 5 to 7 days after injury, the NCS simulates a focal conduction block that is indistinguishable from a neurapractic lesion, with normal conduction distal to the injury but block of conduction with stimulation proximal to the lesion. However, after 5 to 7 days, wallerian degeneration of the axons in the distal segment of the nerve occurs, leading to inexcitability of the distal nerve. This is manifest on NCS by a low or absent CMAP response with stimulation proximal and distal to the site of the lesion. Therefore, within the first week after injury, NCS is useful in localizing the lesion but not in determining severity or prognosis. However, after approximately 2 weeks, NCS can help the clinician distinguish neurapractic from axonotmetic lesions and better determine severity, pathophysiology, and prognosis, but in the case of axonotmetic lesions, it may be less helpful in precisely localizing the injury.
Electromyographic assessment of voluntary motor unit potentials is helpful in identifying whether reinnervation by regrowth or collateral spouting is occurring. In pure neurapractic lesions, the needle examination reveals a reduced number of voluntary motor unit potentials recruited when the patient attempts to produce a more forceful activation of the muscle under examination. There are no fibrillation potentials or change in the amplitude, duration, or structure of the motor unit potentials. In axonotmetic or neurotmetic lesions, the disintegration of the motor axons extends to the nerve terminal at the neuromuscular junction, and the muscle fiber is separated from its innervation. Immediately after the injury, the needle examination reveals reduced recruitment if the lesion is partial or no motor unit activity if the lesion is complete. In partial lesions, the structure of the motor unit potentials should be normal. Fibrillation potentials appear in the denervated muscles after 2 to 3 weeks. In partial or incomplete axonotmetic lesions, the nerve terminals of surviving motor axons begin to produce axonal sprouts 4 days after the injury. These effectively reinnervate some denervated muscle fibers after 3 weeks. At that time, the structure of the surviving motor unit potentials begins to change. There is an increased number of turns or phases on the motor unit potential. As more and more collateral sprouts from a motor neuron reinnervate muscle fibers, the motor unit potentials become more complex, larger in amplitude, and longer in duration.
Imaging of Peripheral Nerve Trauma
Historically, the sensitivity of imaging studies for identifying the anatomy of the peripheral nerve in detail was low, and imaging studies had low utility in the evaluation of peripheral nerve trauma. However, as the quality of imaging has improved, these studies are becoming increasingly useful adjunctive measures for assessing peripheral nerve trauma. Imaging studies can provide important information regarding the continuity of a nerve after trauma. Magnetic resonance imaging with short-time inversion recovery sequences can demonstrate denervated skeletal muscle, which is high in extracellular water content and thereby produces increased signal on short-time inversion recovery and T2-weighted images.5 The distribution of muscle involvement can be extrapolated to determine which nerve or nerves are affected by trauma. More recently, magnetic resonance neurography has evolved to allow for visualization of large peripheral nerves.6,7 Indications for magnetic resonance neurography include the need to visualize roots and nerves for entrapments, adhesions, or the effects of trauma.8 In some cases, magnetic resonance neurography can identify discontinuity of the nerve and therefore is helpful in determining prognosis of recovery.8
Ultrasonography provides less detail of nerve anatomy than does magnetic resonance neurography, but it has been evaluated for its utility in monitoring patients who have undergone direct nerve repair after peripheral nerve injury.9 In a group of 11 patients, reliable identification of the nerve was made with ultrasonography, and neuroma formation, scarring with compression of underlying nerve, and partial discontinuity of nerve fascicles could be identified.9
In cases of injury to the brachial plexus in which root avulsion may have occurred, myelography and computed tomographic myelography are the imaging modalities of choice.10 The myelogram may demonstrate several findings to indicate root avulsion, including pseudomeningocele, poor root sleeve filling, and spinal cord edema or atrophy (Fig. 105-5). Although magnetic resonance imaging may also demonstrate findings suggestive of root avulsion, the findings on computed tomographic myelogram were correlated better with such injury at surgery than were the findings on magnetic resonance imaging.11
CAUSES OF PERIPHERAL NERVE TRAUMA
There are many different causes of nerve trauma, each of which presents distinct issues related to nerve function, recovery, and treatment (Table 105-2). Much of the early information related to nerve trauma was derived from wartime injuries during the American Civil War and the World Wars. Currently, the most commonly encountered cause is blunt or stretch injury related to high-speed motor vehicle accidents, followed by falls, penetrating trauma, and industrial accidents.12 Population studies have estimated that 5% of patients admitted to level I trauma centers have sustained peripheral nerve injuries.13 In many instances of injury related to major trauma, the precise mechanism of nerve injury may not be identified, or several mechanisms may have contributed. For example, in a severe motor vehicle accident, compression, crush, and stretch injury may occur, as may ischemia from arterial damage related to the injury.
TABLE 105-2 Causes and Types of Nerve Trauma
Type of Trauma | Examples |
---|---|
Acute transection | Glass or knife wound, surgically induced |
Chronic compression | Carpal tunnel syndrome |
Stretch injury (stretch, rupture, avulsion) | Traction from motor vehicle accidents |
Injection | Intraneural injections |
Ischemic | Compartment syndromes |
Radiation | Post-irradiation plexopathy |
Temperature (cold) | Frostbite, trench foot |
Acute Nerve Transection or Laceration
Acute transection of the nerve is less common than blunt or compressive injury and occurs with clean, sharp lacerations, such as wounds inflicted by glass or knife injuries. Nerve transactions may also occur as a result of fracture of an adjacent bone or as an iatrogenic injury after inadvertent nerve transection during surgical procedures. In a study of 722 surgically treated cases of nerve trauma, approximately 17% were iatrogenic, the majority occurring during orthopedic procedures or minor surgery.14 The nerve most commonly affected was the spinal accessory nerve (after lymph node biopsy), followed by the common peroneal, radial, and genitofemoral nerves.
In most cases of nerve laceration, neurotmesis or complete transection of the entire axon and surrounding connective tissue structures occurs, producing a distinct separation of the two ends of the nerve. Depending on the length of the nerve separation, potential for regrowth or reinnervation is low. After clean nerve laceration, early surgical repair is usually necessary to align the two ends of the nerve. With lacerations related to blunt injuries, such as chainsaw or propeller blades, a delay of several weeks before surgical intervention may allow for identification and resection of damaged nerve tissue before realignment.15 In iatrogenic surgically induced nerve lacerations, the outcome after surgical intervention was good in approximately half of the patients, with some improvement in sensory, motor, or pain.
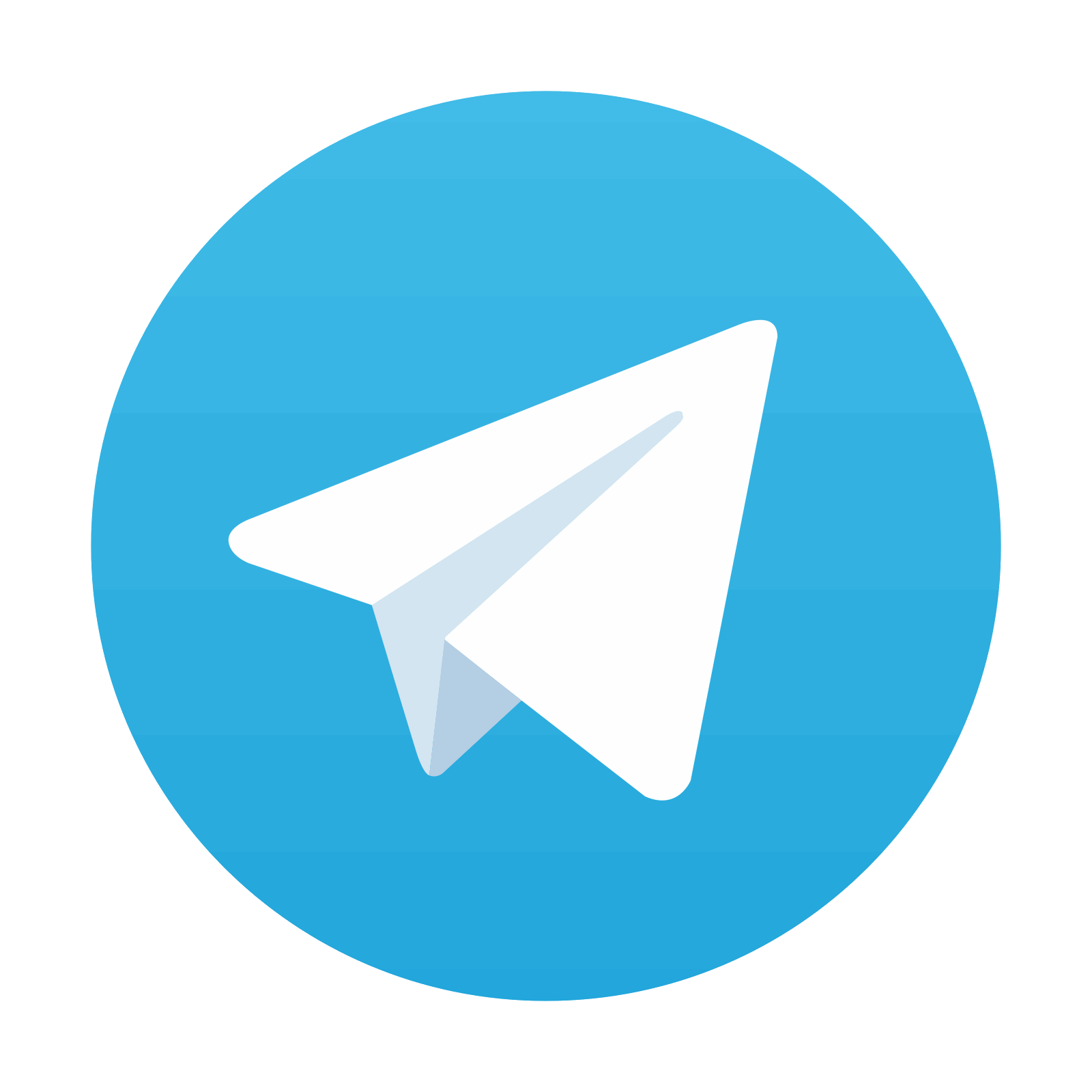
Stay updated, free articles. Join our Telegram channel

Full access? Get Clinical Tree
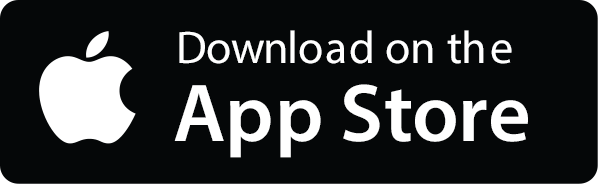
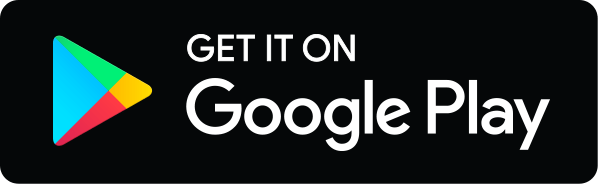