Serotonin Transporter
Alterations in serotonin signaling in PTSD are not only seen at the receptor level. A genetic variant of the serotonin transporter (5-HTT) has been shown to be associated with an increased risk of developing PTSD after a traumatic event [13, 25, 30, 46]. Moreover, some pharmacologic therapies for PTSD target 5-HTT and have been shown to exert a specific neural responses after administration. For instance, a recent PET study of PTSD patients demonstrated increased function in the orbitofrontal cortex during exposure to personalized trauma scripts after treatment with paroxetine [17]. This increase in function may be partially responsible for the decrease in PTSD symptomology seen after treatment with an SSRI. Based on this evidence, Murrough et al. [41] examined the 5-HTT receptor BPND, under resting state conditions, using another selective radioligand [11C]AFM in PTSD patients versus healthy controls. The authors chose the amygdala as their primary region of interest based on the amygdala’s key role in fear processing and the robust body of evidence implicating the amygdala in the pathophysiology of PTSD. Significantly reduced [11C]AFM BPND was found in the amygdala of PTSD patients compared to controls. Additionally, measures of anxiety severity were inversely correlated with BPND. The authors hypothesized that decreased 5-HTT leads to abnormal functioning in the amygdala, which in turn leads to hyperreactive fear responses and increased anxiety. Though these results indicate that altered 5-HTT signaling in the amygdala contributes to the pathophysiology of PTSD, the authors caution that it is presently unknown whether this reduction represents an antecedent risk factor for developing PTSD or results after a severe traumatic event [41]. Importantly though, the described alterations in 5-HTT binding are line with the therapeutic effects of 5-HTT reuptake inhibitors in PTSD where subjective improvement in sleep quality has been reported to be associated with paroxetine treatment [52].
γ-Aminobutyric Acid Receptor
The γ-aminobutyric acid receptor (GABAAR) has been a target of interest ever since the efficacy of benzodiazepines was demonstrated in anxiety disorders [5]. Animal models of chronic stress have shown GABAAR alterations in cerebral cortices and hippocampus [12, 35, 36, 59]. Human pharmacologic studies also provide evidence that modified GABAAR signaling can lead to the severe anxiety seen in PTSD [15]. Furthermore, treatment of PTSD with benzodiazepines has been shown to be efficacious, though there are mixed reports of its ability to treat principal symptomology [2, 7]. Specifically, more recently a magnetic resonance spectroscopy study in combat veterans with PTSD reports an association between low brain GABA concentrations and poor sleep quality providing further evidence about the importance of GABAergic mechanisms mediating certain components of the PTSD phenotype, i.e., abnormal sleep behaviors [37]. Based on this existing body of evidence, Bremner et al. sought to examine GABAAR volume of distribution in Vietnam veterans with combat-related PTSD using SPECT and the selective radioligand [123I]iomazenil. Consistent with the animal literature, they found a decreased volume of distribution of GABAAR in the prefrontal cortex of PTSD patients compared to healthy controls. The authors offered three explanations for these findings: (1) stress downregulates GABAAR expression, (2) stress alters receptor or endogenous ligand affinity, or (3) low levels of GABAAR exist prior to their traumatic event predisposing the individual to developing PTSD. Regardless of the actual pathophysiology, this group concluded that since these alterations were found within the prefrontal cortex, an area implicated in PTSD, this decrease in GABAAR has a role in PTSD symptomology [5].
A few years later, the same group conducted a follow-up study to assess the generalizability of their initial findings in Vietnam veterans. They once again employed SPECT and [123I]iomazenil; however, their patient population was limited to Gulf War veterans with PTSD. This group was selected to test whether GABAAR alterations could be seen after a shorter duration of PTSD. Unlike their original report, they did not find differences in GABAAR in any brain region between the PTSD group and matched healthy controls. Though the authors offered several explanations for this discrepancy, their primary justification was that the Gulf War study group was experiencing a milder form of PTSD as evidenced by fewer severe symptoms and a shorter duration [20]. In 2008, Geuze et al. continued the investigation into GABAAR using PET and the ligand [11C]flumazenil in veterans with and without PTSD. Consistent with the animal data and [5], they found significantly reduced GABAAR binding throughout the frontal cortex, amygdala, hippocampus, and caudate nucleus. The authors discussed possible deficits that may result from GABAAR within a certain region (Table 13.1). Overall, these three studies provide ample evidence to implicate the GABAAR in PTSD; however, further work will be required to tease out differences in GABAergic function related to duration, symptom severity, and trauma type [22]. In addition, while neuroimaging has been used to elucidate GABAergic mechanisms in sleep regulation and its dysfunction in PTSD, molecular imaging techniques have not yet sufficiently explored this topic.
Table 13.1
Hypothesized functional deficits in brain regions found to have decreased GABAAR binding [22]
Region | Functional deficit |
---|---|
Frontal cortex | Working memory |
Hippocampus | Modulation of emotional behavior |
Amygdala | Fear processing |
Caudate nucleus | Implicated in other anxiety disorders |
Dopamine
Dopamine is associated with mood, emotion, cognitive functioning, and stress response. Furthermore, dopaminergic dysfunction has been implicated in the hyperarousal symptom cluster commonly seen in PTSD patients [8, 57, 58]. For instance, high plasma and urinary dopamine levels have been reported in traumatized individuals with and without PTSD [14, 18, 26]. In addition, several genetic alterations have been linked to dopaminergic dysfunction in PTSD [6, 16, 51].
Of particular interest in recent years has been variation in the dopamine transporter (DAT) gene (SLC6A3), which functions in synaptic dopamine reuptake. The 9-repetition (9R) allele , a specific variant located at this gene, has been shown to increase PTSD susceptibility [55]. Furthermore, healthy individuals with the 9R allele have demonstrated increased DAT levels in particular brain areas (e.g., [56]). With this evidence in mind, Hoexter et al. performed one of the only molecular imaging studies of dopamine using single-photon emission computed tomography (SPECT) and the selective ligand [99mTC]-TRODAT to investigate DAT density in PTSD compared to trauma-exposed controls. They found increased DAT BPND in the striatum of the patient group compared to trauma controls, indicating dopaminergic hyperactivity leading to amplified and prolonged fear responses in PTSD [27]. The specific implications of these findings are discussed in Fig. 13.2. Although not yet sufficiently explored using molecular neuroimaging techniques, the association between dopamine dysfunction and abnormal sleep in PTSD [21] was supported by reports that periodic leg movements are often found in sleep disorder patients [28], including PTSD [48]. Similar to other monoaminergic mechanisms, neuroimaging methods have not yet been sufficiently used to explore the specific molecular targets that could play a role in mediating abnormal sleep patterns and nightmares in PTSD. With novel treatments that target this system being currently explored, one would hope that this specific link will be studied in the near future.
μ-Opioid Receptor
Preclinical work has suggested that opioidergic mechanisms are implicated in amygdala-regulated processes including feeding behaviors [24], antinociception [53], normal fear responses [60], and conditioned fear [23]. The development of [11C]-carfentanil , a high affinity opiate ligand, made possible the translation of some of these preclinical findings to humans by facilitating the localization of μ-opioid receptors via PET imaging [19].
Abnormal cerebral blood flow in brain areas such as the hippocampus, somatosensory areas, cerebellum, insula, putamen, medial prefrontal cortex, and anterior cingulate cortex is known to be associated with dysfunctional emotional processing [31, 32, 44, 45]. To more clearly understand the mechanisms that underlie these blood flow changes, Liberzon et al. [33] used [11C]-carfentanil and PET in a group of 12 healthy people to examine the functional relationship between blood flow and μ-opioid binding potential , a measure of receptor density. They found that higher baseline binding potential was related to lower blood flow in temporal and basal forebrain regions during exposure to emotionally aversive images. The inverse association between receptor density and regional blood flow implied an inhibitory or anxiolytic role of the endogenous opioid system.
This relationship was further investigated by Zubieta et al. using [11C]-carfentanil and PET in a group of healthy women [61]. They found that during a state of self-induced sadness , μ-opioid receptor density increased in the anterior cingulate, ventral pallidum, amygdala, and inferior temporal cortex, signifying a compensatory response following downregulation of the endogenous neurotransmitter in these areas. These data further demonstrated a strong link between emotional regulation and μ-opioid neurotransmission.
Liberzon et al. conducted the first study to investigate the role of μ-opioid transmission in PTSD specifically [31, 32]. Rather than using a cognitive activation paradigm like previous related studies, here they investigated baseline μ-opioid binding potential in a group of combat veterans with PTSD, combat-exposed veterans without PTSD, and a healthy control group. Compared to healthy controls, both trauma-exposed groups demonstrated reduced binding potential in the amygdala, nucleus accumbens, dorsal frontal cortex, and insular cortex and increased binding potential in the orbitofrontal cortex. Concerning the PTSD group specifically, data revealed reduced binding potential in the anterior cingulate cortex and increased binding in orbitofrontal cortex. These results validated previous studies concerning the relationship between the μ-opioid system and emotional processing, but, importantly, they revealed a more intricate relationship between this system and PTSD specifically.
Conclusion
The development of novel radioligands has allowed us to explore precise molecular processes that are implicated in the pathophysiology of PTSD via PET imaging. Importantly, molecular quantification of receptors and transporters has enabled us translate hypotheses about neural dysfunction from animal models to the living human brain. However, while there is strong evidence that abnormal sleep and nightmares are key symptoms of PTSD, PET and SPECT imaging techniques have not yet been sufficiently used to describe the neurochemical mechanisms that mediate these symptom domains. Such studies, however, offer the possibility to not only unravel further the etiology of PTSD but have the potential to also support treatment development efforts.
As we continue to explore the pathophysiology of PTSD by using existing radioligands in innovative ways, new radioligands will continue to emerge. Novel targets can be identified through animal experiments and other molecular imaging projects in disorders that are related or comorbid with PTSD. Bailey and Neumeister [1] recently reviewed several animal and human studies of anxiety that implicate altered cannabinoid type 1 (CB1) receptor-mediated signaling in the development of stress-related psychopathology. Furthermore, recent PET imaging with the CB1-selective radioligand [11C]OMAR has demonstrated an upregulation of CB1 receptor density in men with alcohol dependence, a frequent comorbidity with PTSD, when compared to healthy individuals [43]. This work represents one of the many avenues that could be explored in the future with specific ligands and PET imaging. The endocannabinoid system appears of particular interest for better understanding the role of sleep disturbances and its treatment in PTSD given a recent report showing that use of cannabis was particularly high in trauma survivors who aimed to improve their sleep [3].
The data presented in this chapter represent remarkable leaps in our understanding of PTSD, although abnormal sleep which is a core symptom of PTSD has not yet taken advantage of recent developments in this area. However, it is important to recognize the limits of their applications. PTSD is a multiplex disorder whose pathogenesis involves many interconnected neurobiological systems. It is necessary to understand how these individual systems function differently in people with and without PTSD; but to fully understand the pathogenesis of this complex disorder, we must decipher how each of these pieces fits into a larger, more synergetic picture.
References
1.
Bailey CR, Neumeister A (2011) Cb1 receptor-mediated signaling emerges as a novel lead to evidencebased treatment development for stress-related psychopathology. Neurosci Lett. 502(1):1–4.
2.
3.
Bonn-Miller M, Babson K, Vandrey R. Using cannabis to help you sleep: heightened frequency of medical cannabis use among those with PTSD. Drug Alcohol Depend. 2014;136:162–5.CrossrefPubMed
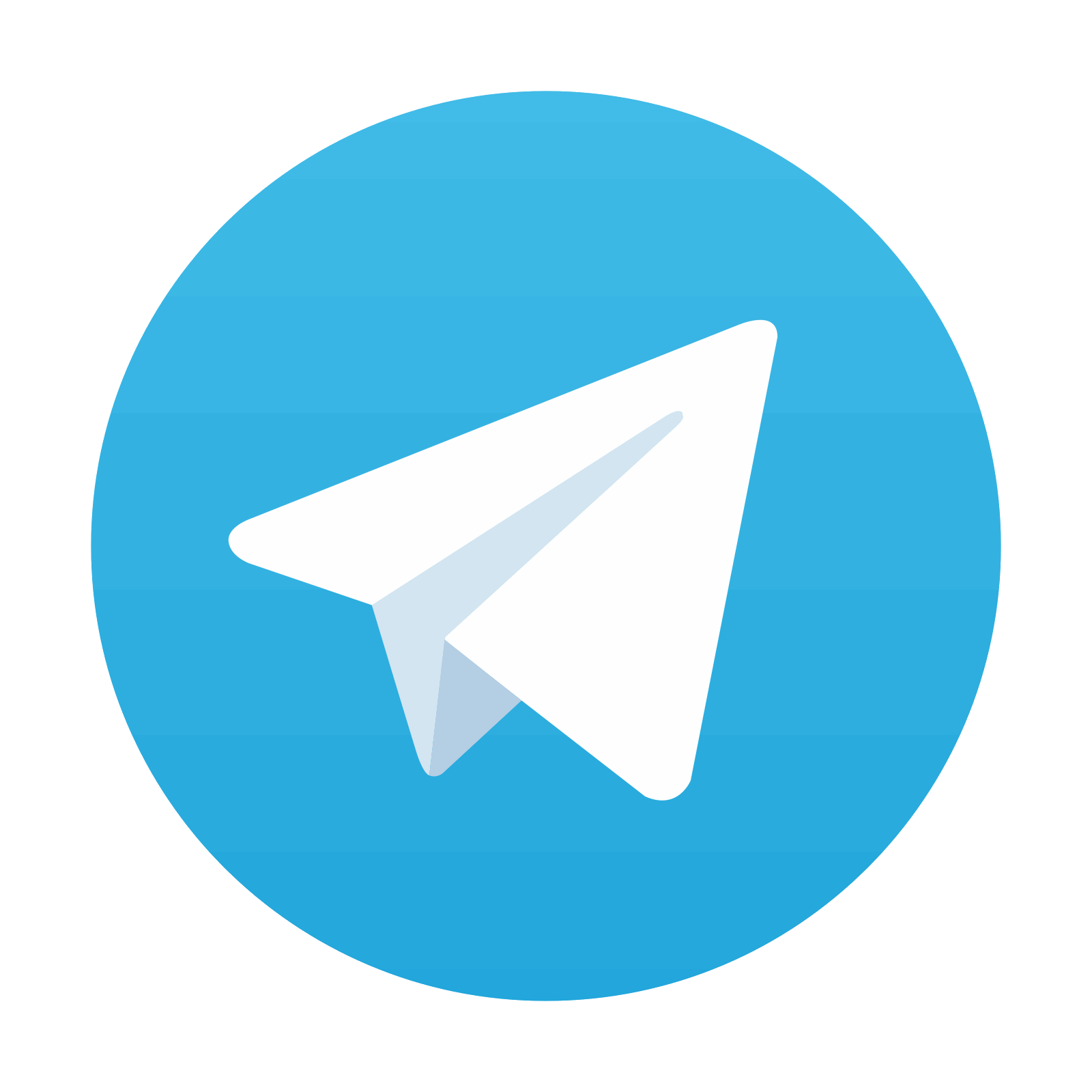
Stay updated, free articles. Join our Telegram channel

Full access? Get Clinical Tree
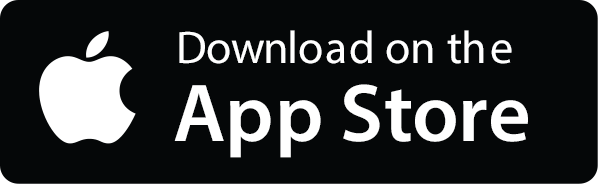
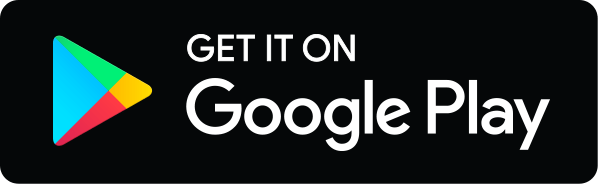