INTRODUCTION
It is well recognized that in a process of cognitive decline leading to mild cognitive impairment (MCI) and dementia, detection of the underlying changes in brain structure and function will contribute to establishing an accurate diagnosis, with direct implications for pharmacological and non-pharmacological therapy planning. Since the first positron imaging device in 1950 or the first tomographs from single photon emission data in the 1960s, scientific advances in radionuclide development and detection research have led us to look beyond the brain’s regional blood flow (SPECT) or glucose metabolism (FDG-PET) into the patterns of amyloid plaque (PIB-PET) and tau tangle brain deposits (FDDNP-PET) or neuronal disintegrity (MPPF-PET). With new methods entering clinical settings, we will need to balance our expectations for these new tools against availability and costs. Here we review the current state of metabolic and molecular imaging tools used in ageing and dementia, their utility, promise, as well as potential difficulties in bringing them into common practice. We will first review the basic techniques and then discuss their applications, including practical guidelines and results from the most recent studies reporting sensitivity, specificity and optimal uses.
Image Acquisition
Single photon emission computed tomography (SPECT) measures the distribution of radioligands in the brain reflecting regional cerebral blood flow (rCBF), which itself is linked to brain metabolism. The tracer accumulates in proportion to the rate of delivery of blood to a distinct volume of brain tissue. Decades ago to patients this would have meant injection of 133Xe the carotid artery. Today, within the commercial and experimental available radioligands technetium-99m labelled hexamethylpropyleneamine (99mTc-HMPAO) and ethylcysteinate dimer (99mTc-ECD) tracers are commonly used in brain SPECT imaging. After intravenous injection, these lipophilic compounds quickly cross the blood-brain barrier by diffusion and, due to slow brain clearance, the distribution of the radioligands then remains stable for hours. The underlying multicompartment clearance (‘microsphere’) model assumes that the tracer is freely diffusible, completely extracted to the brain and ‘fixed’ without redistribution. The emitted single photons can be detected by a rotating gamma camera or ring-type imaging system. Spatial resolution is about 10 mm and could reach 5-6mm using special-purpose detection systems that usually are not available for routine clinical scans.
Spatial resolution in SPECT is only limited by technology (whereas PET resolution also depends on other factors1 ; see below) but today SPECT cannot compete with PET on image resolution and begins to lose its uniqueness within other factors. Cost-effectiveness still remains a controversy from a perspective that would take diagnostic accuracy and secondary costs into account; further, PET has become much more available, which also will make it less expensive. Resulting from physics-based differences, the possibility of simultaneous imaging of more than one radioagent is SPECT territory and a growing field of interest. Since all PET tracers share the same energy level, it would be much more difficult to perform simultaneous multiple tracer studies in PET. Signals would have to be separated based upon half-life or kinetics, which seems feasible in the future1,2.
Advanced SPECT technology is heavily reliant on detector improvements, use of hybrid imaging (e.g. SPECT/CT) and incorporation of attenuation or collimator-detector response modelling into image reconstruction algorithms (for review see1).
The clinical protocol requires the application of 20mCi (740 MBq) of 99mTc-HMPAO or 99mTc-ECD 10 to 20 minutes before imaging with HMPAO and 30 to 60 minutes before image acquisition with ECD. Image acquisition itself will take 30 minutes or less. Total radiation exposure is small (about 9 mSv) and in the range from one to three times of the annual natural background.
Memory Impairment and SPECT Imaging
In the field of dementia and related disorders, SPECT has been used widely for both basic science and clinical applications. Alzheimer’s disease patients display a characteristic pattern of posterior temporal and parietal perfusion deficits. Sometimes additional reduced frontal rCBF has been detected, associated with behavioural disturbances, such as depressed mood or apathy3. Correlations between neuropsychological performance and rCBF have been reported consistently, especially with focus on language and global functioning4. Pharmacological activation studies demonstrated the relationship of, e.g. cholinergic stimulation and rCBF5.
In addition to clinical assessment, SPECT studies have been shown to improve diagnostic precision. Jagust and colleagues reported that a positive SPECT result would increase the likelihood of a diagnosis of Alzheimer’s disease by a factor of five6. In a meta-analysis, 99mTc-HMPAO-SPECT could discriminate between Alzheimer’s disease, vascular dementia (VaD), frontotemporal dementia (FTD) and normal controls with sensitivities between 66% and 72% and specificities between 76% and 79%. Diagnostic accuracy is improved especially in patients who present with additional signs of cerebrovascular disease or who do not exactly match clinical criteria7. Furthermore, SPECT has been shown to be useful predicting the conversion to Alzheimer’s disease in patients with MCI8.
Measuring cerebral metabolism indirectly using rCBF enhances vulnerability to vascular-related confounders, such as hypertension and other specific cerebrovascular diseases. It has been shown that rCBF differences can be found with respect to gender and advanced age itself9. Changes in rCBF are not unique to memory disorders and have been observed in depression10 and obsessive-compulsive disorder11 as well.
Currently, most clinical SPECT scans are performed in cardiology. 99mTc-labelled tracers can be used to perform dynamic imaging (e.g. myocardial perfusion SPECT) but in the future, dynamic PET (e.g. 82Rb-based cardiac PET) imaging might have an intrinsic advantage over SPECT since temporal resolution is very closely related to the imaging system’s specificity1.
In addition to regional cerebral perfusion, various neurotransmitter systems have been a target of interest in SPECT tracer development. Dopamine transporter imaging with 123I-2ß-carbomethoxy-3ß-(4-iodophenyl)-N -(3-fluoropropyl) nortropane (123I-FPCIT), which can be used in the differential diagnosis of Parkinson’s disease, could help to distinguish between dementia with Lewy bodies (DLB) and Alzheimer’s disease, if the clinical diagnosis remains ambiguous. Since the cholinergic system is of particular interest in dementia, radiolabelled muscarinic and nicotinic acetylcholine SPECT ligands are currently under investigation in preliminary studies.
Image Acquisition
Positron emission tomography (PET) has more diverse applications in imaging in ageing and dementia, as it can be used to generate images of regional cerebral blood flow (e.g. [15O]H2O-PET), metabolic activity (e.g. [18F]FDG-PET), microstructures (e.g. [18F]FDDNP-PET) or neurotransmitter receptor distribution (e.g. [18F]MPPF-PET) in the brain. After being injected intravenously, the isotope emits a positron, which after travelling a few millimetres annihilates with an electron. As a result, two 511 keV gamma photons, moving in almost opposite directions, are produced and then detected using a scintillator material (luminescent when excited by ionizing radiation) in the ring-type scanner. Only coincident arrivals of photons are recognized (within a few nanoseconds’ error margin). Because of the peculiar dataset (‘coincidence events’, each representing a line connecting the two points of detection along which the annihilation occurred) compared to other neuroimaging techniques data, preprocessing and mathematical image reconstruction are challenging. Spatial resolution of PET images is about 3 mm and higher and limited by technological factors (detector-related effects) but also physics-related limitations (positron range and photon non-collinearity)1. Improvements in PET technology will be seen in both cases. New scintillators for reduction of random coincidences and silicon-based photodetectors will improve detection. PET/MRI hybrid imaging systems could be used for monitoring patient motion via MRI to incorporate the data in PET image reconstruction, maybe replacing PET/CT in the future1,12. Even ultimate limitations (positron range/photon non-collinearity) have been a target since new statistical reconstruction algorithms could incorporate their probability distributions into image processing or a strong magnetic field (preferably 5 T or higher) of a future PET/MRI system should be able to reduce positron range and therefore enhance scanner resolution1. Time-of-flight-PET, as one of the most promising technological advances, uses the nanosecond difference of photon arrival times to restrict the position of the positron emission to a subsection of the photons’ connection line, which reduces the statistical noise variance in PET reconstruction1.
PET isotopes usually have shorter half-lives compared to SPECT ligands. The most common PET isotopes are 11C (half-life: 20min), 13N (10min), 15O (2min) and 18F (110min). Only a few hospitals have costly on-site cyclotrons, needed to produce them. Third-party suppliers are able to provide 18F because of its relatively long half-life and therefore clinical use is often restricted to 18F-labelled ligands. On the other hand, short half-lives allow the safe injection of higher activities, increasing detectable radiation.
The most common clinical application of PET is in resting measures of glucose metabolism, with [18
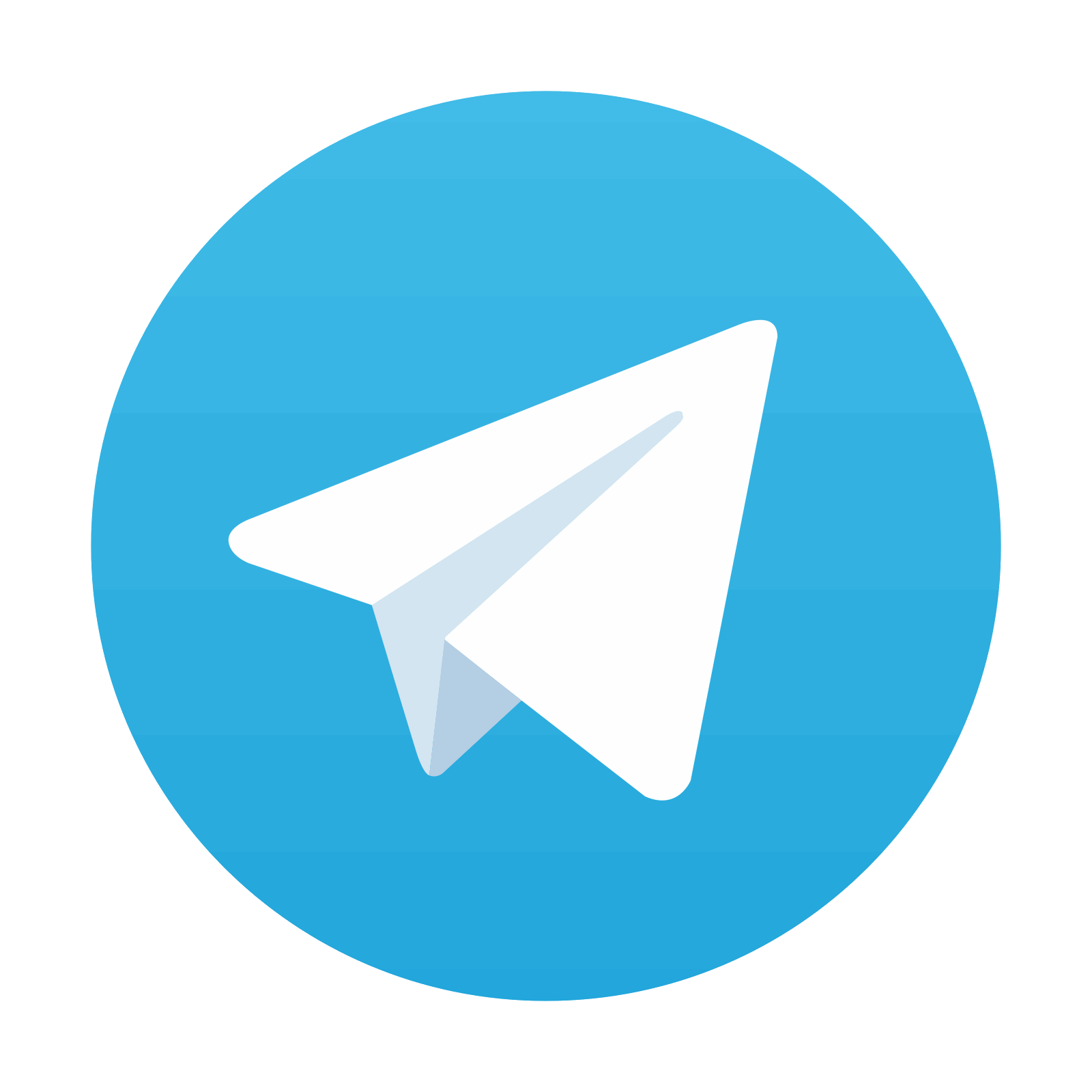
Stay updated, free articles. Join our Telegram channel

Full access? Get Clinical Tree
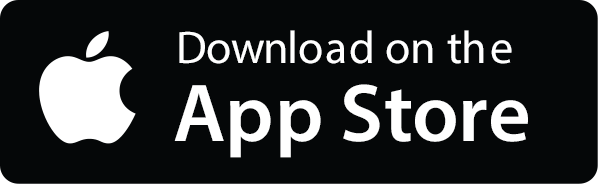
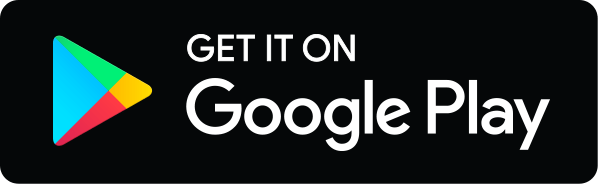