Cardiac arrest and hypoxic brain injury can cause encephalopathy, coma and seizures. There are several clinical and electrographic considerations that are fairly specific to the post cardiac arrest EEG. After cardiac arrest, patients can be in myoclonic status epilepticus. This is a clinical syndrome that is associated with significant hypoxic brain injury and for the most part holds a poor outcome (although not universally). In the post cardiac arrest setting, one thing EEGers are asked frequently is to make some estimate on prognosis based on the EEG. This chapter will introduce patterns that are more specific to the post cardiac arrest setting that are associated with a poor (or good) prognosis. It should be noted, however, that none of these are absolute (although some reportedly close) and a discussion of prognosis must be integrated with the remainder of the clinical features, and other electrophysiologic information, including somatosensory evoked potentials (SSEPs). Myoclonic status epilepticus is the syndrome whereby there is continuous or near continuous myoclonus after cardiac arrest. Myoclonus is often proximal with jerking of the eyes, face, shoulders or trunk/abdomen. Although often dramatic the state can also be fairly subtle, limited to just the eyelids and eyes for example. The EEG correlate of myoclonic status epilepticus is often suppression-burst or generalized periodic discharges, with the clinical jerk associated with the beginning of the burst or the discharge. Myoclonic status epilepticus is said to carry a grave prognosis; however, many of the studies that concluded this preceded the era of therapeutic hypothermia or targeted temperature management (TTM) and are retrospective. This raises the possibility of a self-fulfilling prophecy whereby a common practice was to withdraw care based on the clinical and EEG finding. This has been questioned in more recent times with the emergence of an increasing number of reported cases that have had myoclonic status epilepticus and have gone on to have a good clinical outcome; this is usually in patients with a better (continuous) EEG background and no other indicators of poor prognosis (e.g., loss of brainstem reflexes or bilaterally absent SSEPs). Prognostication after cardiac arrest is a complex integration of clinical history, patient examination, neuroimaging and neurophysiologic measures. The EEG is merely one of these factors and the electrographic patterns should be weighted in conjunction with the remainder of the patient’s findings. A patient whose EEG demonstrates reactive slowing with clear physiologic sleep states has a much better chance of good outcome than a patient with no clear cerebral rhythms, including after stimulation. However, these points are never absolute (i.e., a patient with reactive slowing still has a reasonable chance of succumbing to death). The patients who fall in between these end points can be more challenging, and there are a number of patterns emerging that sway prognosis toward one end of the spectrum versus the other. In general, the background EEG is the most important prognosticator, and the EEG around 24 h after arrest may be the most useful timepoint (though the trajectory may be important as well). There are many features of the EEG that have demonstrated prognostic significance in post cardiac arrest patients. The background voltage and continuity, the theta spectral content within burst suppression (i.e., the amount of relative power in the theta range), the time taken to recovery of a normal-voltage continuous background, and reactivity have all shown to have prognostic implications. Negative prognostic indicators have included the presence of SIRPIDs, a shorter time to the emergence of epileptiform activity, and electrographic and electroclinical seizures (usually myoclonic status epilepticus); again, none of these are absolute indicators of poor outcome, but they are associated with worse outcomes overall. A pattern with a great degree of emerging dichotomy is burst suppression. Burst suppression is defined as 50–99% of the record being suppressed, but the amount of inherent brain dysfunction in a patient with 98% of their record suppressed is clearly greater than a patient with 50% of their record attenuated. Burst suppression actually consists of a wide variety of patterns. Bursts can consist of fairly benign appearing admixed theta and delta range frequencies, or bursts can appear highly epileptiform (with a significant component of epileptiform activity). Two concepts have emerged to further refine the group of burst suppression. Highly epileptiform bursts are defined as either: Highly epileptiform bursts (HEBs) were initially described (in the non-cardiac arrest setting) as a differentiating factor for seizure recurrence if highly sedating ASMs used to control refractory status epilepticus were withdrawn. For example, in one study, in patients with HEBs at the time of sedative withdrawal, they had a 65% chance of seizure recurrence in the next 24 hours, vs. 0% if the EEG did not demonstrate HEBs at the time of medication withdrawal. It was subsequently established that the presence of HEBs in post cardiac arrets patients also inferred an independent poor prognostic factor. It should be noted that unequivocal seizure also pertains a poor prognostic factor in this setting, but the majority of these refer to the emergence of myoclonic status epilepticus; other forms of postanoxic status epilepticus seem to have a better chance of favorable outcome. The other prognostic feature is ‘identical’ vs ‘non-identical’ bursts. Non-identical bursts are more physiologic, with the content of bursts varying between each other. Identical bursts on the flip side can be viewed as an extreme form of invariance, where the brain is so dysfunctional that it is only capable of generating the exact same group of rhythms over and over again. Identical bursts are defined as follows: The presence of identical bursts in the post cardiac arrest setting may have a nearly universal poor outcome, though there are limited data on this to date. In addition, there is an important caveat that although the prognosis of many of the above patterns are termed dire, there are examples in the literature whereby patients with these patterns have a good outcome. There are even descriptions of patterns that are associated with good outcome in patients with postanoxic myoclonus, such as narrow vertex-maximal spikes time-locked to the jerks. It must be stressed that the clinical scenario is critical for prognostication and these life-or-death decisions should not be based on one test alone. The EEG is not usually helpful in patients in a vegetative or minimally conscious state as it can show a wide variety of degrees of diffuse dysfunction. EEG records cortical activity, not brainstem activity. Thus, in patients with primary brainstem injury, the EEG can appear remarkably ‘healthy’. A completely flat EEG (electrocerebral inactivity) is seen with severe diffuse injury, with or without loss of brainstem function, which must be determined by other means (primarily examination, including apnea testing). There are detailed, formal guidelines for performing an EEG in order to document electrocerebral inactivity, though now these are only used on rare occasions as part of the determination of brain death due to the limitations just mentioned. EEGs throughout this atlas have been shown with the following standard recording filters unless otherwise specified: LFF 1 Hz, HFF 70 Hz, notch filter off.
8
Post cardiac arrest patterns
8.1 Myoclonic status epilepticus
8.2 Prognostication
Figure list
Suggested reading
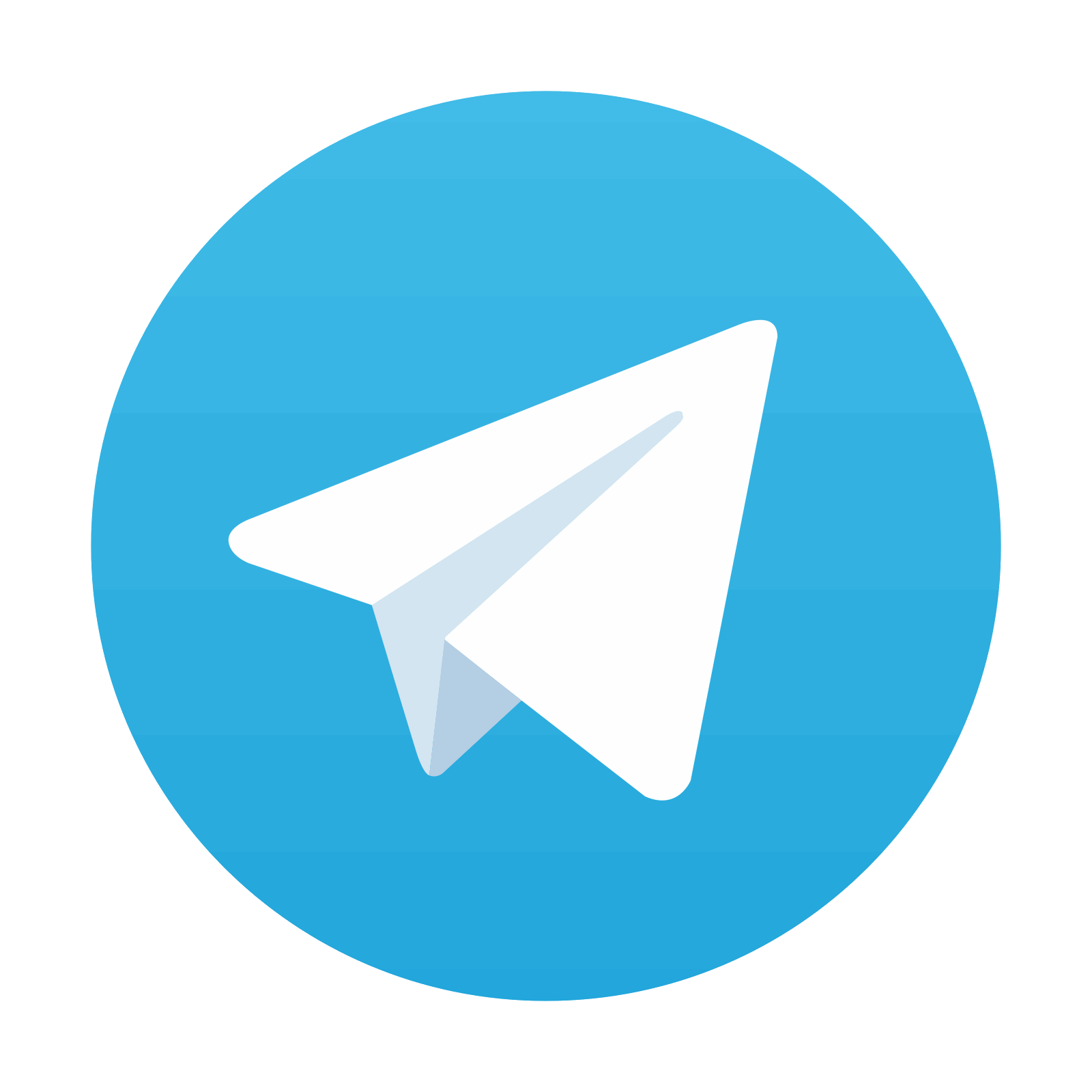
Stay updated, free articles. Join our Telegram channel

Full access? Get Clinical Tree
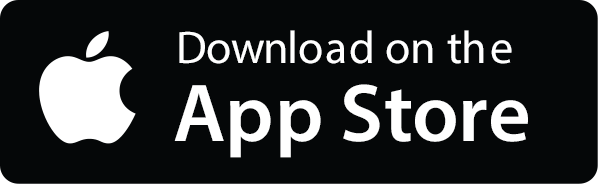
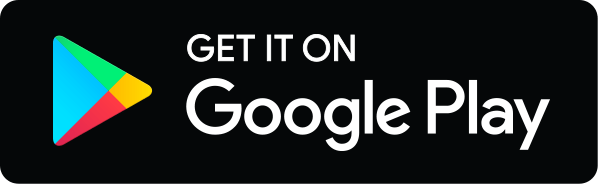