Postchemotherapy Morbidity
For many decades the standard of care for brain tumors was surgery and radiotherapy. Chemotherapy for the treatment of leukemia and solid, non–central nervous system (CNS) tumors was well established by the 1970s, but it was only in the late 1980s and the early 1990s that trials involving chemotherapy for brain tumors began to appear, so that information on their long-term quality of survival remains sparse ( Table 46.1 ).1 The childhood cancer survivor study, for example, had 1,607 participants, but these included only 26 (1.6%) of survivors treated with surgery plus chemotherapy alone.2
Numerous studies have suggested that the use of chemotherapy is associated with a reduction in tumor size and an increase in event-free survival rates in children with medulloblastomas, low-grade gliomas, and germ cell tumors. Some infants with a variety of brain tumors have benefited from chemotherapy that was introduced in an attempt to improve their chances of survival and, more importantly, to delay or reduce the use of radiotherapy, which is damaging to the maturing brain. In only very few studies, however, is it the case that participants have been randomly assigned to radiation therapy with chemotherapy versus radiation therapy without chemotherapy or treatment with chemotherapy versus treatment with radiation therapy. Attempts to assess whether chemotherapy interacts with radiation therapy to increase its morbidity are therefore confounded by factors other than the addition of chemotherapy.
Over half of survivors of childhood brain tumor having moderate or severe disability.2,3 Age, tumor location, complications of surgery, and radiotherapy are recognized to explain much of the variance in disability, but chemotherapy undoubtedly also contributes to it. This chapter is not intended be an exhaustive account of all known side effects of chemotherapy. Rather, this chapter discusses the neurocognitive and long-term morbidity attributable to chemotherapy and the well-known and anticipated side effects encountered during and after chemotherapy administration, as follows:
Morbidity affecting the central and peripheral nervous system
Acute encephalopathy, including reversible posterior encephalopathy and stroke
Chronic leukoencephalopathy and other morbidity on imaging
Neurocognitive impairment
Myelopathy and peripheral neuropathies
Hearing loss
Renal morbidity
Gonadotoxicity and other hormonal morbidity
Second cancers after brain tumors
Side Effects of Chemotherapy
Common, infrequent, and late unwanted effects of chemotherapy drugs established for use in brain tumors are listed in Table 46.2.
Morbidity Affecting the Central and Peripheral Nervous System
An excellent review by Soussain and colleagues4 of CNS complications of radiotherapy and chemotherapy in patients with cancer was published in 2009.4
Acute Encephalopathy, Including Reversible Posterior Encephalopathy and Stroke
Headache and seizures can occur with most chemotherapeutic agents. Seizures may be caused either directly or indirectly by overhydration, vincristine-induced inappropriate antidiuretic secretion, or renal toxicity (e.g., cisplatin). Five to 30 percent of patients receiving ifosfamide chemotherapy may develop CNS toxicity, including seizures, visual disturbances, auditory and visual hallucinations, acute confusional state, mutism, extrapyramidal signs, and, rarely, progressive irreversible coma.5 Symptoms and signs are transient, although fatalities have occurred.6 The neurotoxicity is probably related to dose and rate of administration, may be exacerbated by phenobarbitone and previous platinum chemotherapy, and a previous event predicts recurrence. Ifosfamide encephalopathy may be prevented or treated with methylene blue and benzodiazepines.7,8
Tumor pseudoprogression occurring 6 weeks to 3 months after radiotherapy and mimicking tumor recurrence is due to early radionecrosis or chemonecrosis and is seen more often with combined treatment than with either modality alone.9 White matter lesions occur subacutely within months of combined radiation therapy and high-dose chemotherapy for medulloblastoma, and in some cases are associated with long-term neurocognitive deficits.10 The treatment for this acute and sub-acute morbidity is steroids and supportive care.
Cisplatin, cytarabine, cyclosporins, cyclophosphamide, methotrexate, rituximab, and paclitaxel are among a growing list of chemotherapeutic agents that are closely associated with reversible posterior leukoencephalopathy.11 This syndrome may present with headache, nausea and vomiting, seizures, visual disturbances, altered sensorium, and occasionally focal neurologic deficit.12 It is often associated with hypertension or hypotension, either primary or induced by treatment for hypertension. Magnetic resonance imaging (MRI) typically shows symmetrical hyperintense lesions on T2 sequences. Lesions particularly involve the posterior parietal and occipital lobes, and may spread to the basal ganglia, brainstem, and cerebellum. The pathogenesis is a matter of continuing debate, but partial or complete clinical recovery usually follows withdrawal of the implicated chemotherapeutic agent or establishment of normotension.13
Cerebrovascular disease is markedly increased in survivors of childhood brain tumors, Hodgkin′s lymphoma, and acute lymphoblastic leukemia.14 Although radiotherapy is the commonest risk factor, chemotherapy also contributes to the risk. Venous sinus thrombosis can complicate use of asparaginase, mitomycin predisposes to thrombotic microangiopathy, and anti–vascular endothelial growth factor (VEGF) drugs can cause both hemorrhagic and thrombotic stroke.15,16
Chronic Leukoencephalopathy and Other Morbidity on Imaging
Chronic leukoencephalopathy is a demyelinating process with focal or diffuse areas of white matter necrosis (cortical gray matter is spared), microangiopathy, dystrophic calcification, and oligodendroglial and glial cell damage or loss. It commonly occurs after radiotherapy but is also seen as a consequence of chemotherapy protocols that include high-dose methotrexate given intravenously or intrathecally.
On computed tomography (CT) scan, characteristic features include calcification, often seen in the basal ganglia, hypodense lesions, and widened subarachnoid spaces. The MRI features have been graded by Zimmerman et al17 ( Table 46.3 ). The clinical and imaging findings have also been incorporated into the National Cancer Institute Common Terminology Criteria for Adverse Events.18 The clinical features of leukoencephalopathy may include focal motor signs, seizures, ataxia, cognitive abnormalities (including memory loss and dementia), and death ( Table 46.4 ).
Treatment-related leukoencephalopathy was first identified in the population of children being treated for acute lymphoblastic leukemia. These children from the 1970s and until the late 1990s were being treated routinely with cranial irradiation. It was thought that methotrexate only caused leukoencephalopathy when administered in conjunction with cranial irradiation or when leukemia had entered the cerebrospinal fluid.19 However, a subsequent study by the Pediatric Oncology Group (POG 9005), which involved leukemic patients without CNS involvement and who received no cranial irradiation, found a significant number of patients with leukoencephalopathy, with more patients who received IV methotrexate being affected than those who received oral methotrexate.20
Methotrexate leukoencephalopathy probably occurs due to the cumulative amount of methotrexate, the frequency of administration, and the absence of leucovorin rescue in patients receiving intrathecal methotrexate. As these are situations that commonly arose in children being treated for brain tumors, it is not surprising that attention focused on the incidence of leukoencephalopathy in children with brain tumors receiving methotrexate.
Rutkowski et al21 described the German experience of treating children under the age of 3 years diagnosed with medulloblastoma. Their treatment was maximal surgery followed by three cycles of intravenous cyclophosphamide, vincristine, carboplatin, etoposide, and high-dose methotrexate. Thirty-six doses of intraventricular methotrexate were also given during this time. T2-weighted cranial MRIs were available for the evaluation of leukoencephalopathy during and after treatment in 23 of their 43 patients. Leukoencephalopathy was not detected in four patients. In 19 patients, all without related symptoms, leukoencephalopathy was classified as mild (spotted, circumscribed lesions in four children), moderate (patchy lesions in nine children), or severe (confluent lesions in six children). The maximal grade of leukoencephalopathy was observed 1 year after treatment. At further follow-up, the severity of leukoencephalopathy decreased to a lower grade in 10 patients and remained stable in eight patients. Correlation between the grade of leukoencephalopathy and cumulative dose of intraventricular methotrexate, but not intravenous methotrexate, was found. Although neuropsychological assessments were performed on some of the patients in the study, no firm conclusions could be drawn about the significance of the leukoencephalopathy for neuropsychological outcome.
Kellie et al22 described the Australian and New Zealand experience in children older than 3 years of age at the time of diagnosis of medulloblastoma.22 Eight of 12 patients had Common Terminology Criteria for Adverse Events (CTCAE) grade I leukoencephalopathy, and the other four had the grade II features on MRIs of survivors from a previous phase II study who were at least 4 years posttreatment.
Leukoencephalopathy is therefore a common occurrence in children with brain tumors receiving methotrexate intravenously or intraventricularly, with or without radiotherapy. Its clinical significance, particularly for long-term neuropsychological morbidity, needs further investigation.
More sophisticated analysis of imaging features in medulloblastoma survivors, reviewed by Palmer,23 has suggested that a decrease in the volume of normal-appearing white matter and impaired hippocampal neurogenesis, and lower fractional anisotropy of white matter on diffusion tensor MRI are associated with neurocognitive impairment.
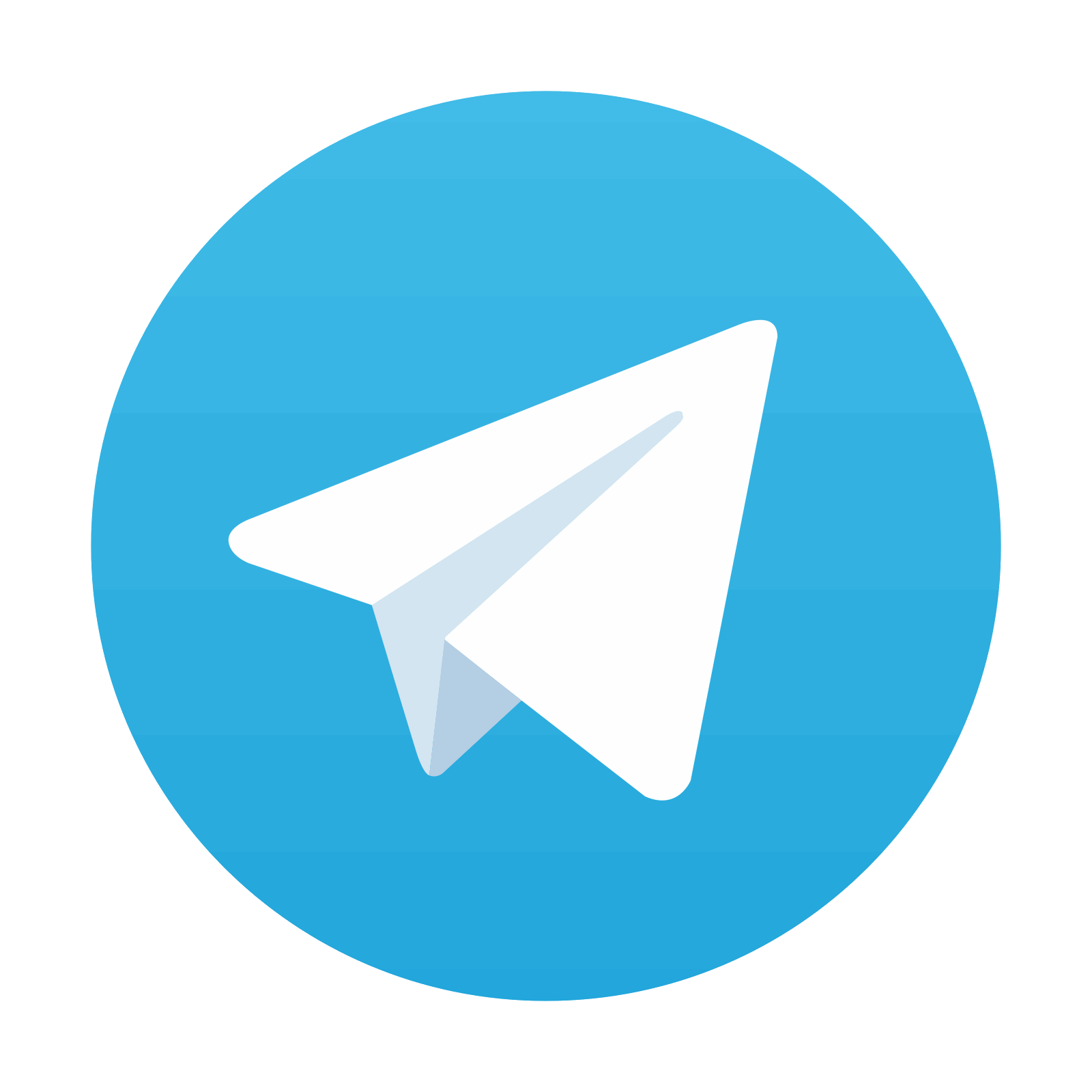
Stay updated, free articles. Join our Telegram channel

Full access? Get Clinical Tree
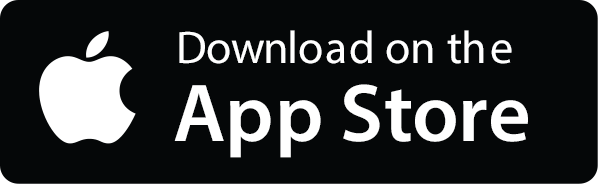
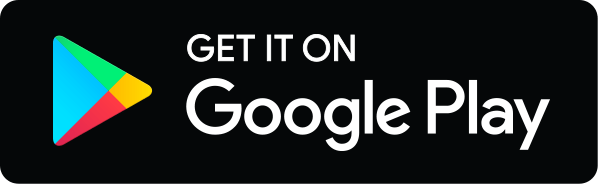
