Posture
Postural Equilibrium and Orientation Are Distinct Sensorimotor Processes
Postural Equilibrium Requires Control of the Body’s Center of Mass
Automatic Postural Responses Counteract Unexpected Disturbances
Automatic Postural Responses Adapt to Changes in the Requirements for Support
Anticipatory Postural Adjustments Compensate for Voluntary Movements
Somatosensory Afferents Are Important for Timing and Direction of Automatic Postural Responses
Vestibular Information Is Important for Balance on Unstable Surfaces and During Head Movements
The Postural Control System Uses a Body Schema that Incorporates Internal Models for Balance
Control of Posture Is Distributed in the Nervous System
Spinal Cord Circuits Are Sufficient for Maintaining Antigravity Support but Not Balance
The Brain Stem and Cerebellum Integrate Sensory Signals for Posture
The Spinocerebellum and Basal Ganglia Are Important in Adaptation of Posture
THE CONTROL OF POSTURE IS CRUCIAL for most tasks of daily living. The two components of posture, orientation and balance, require continual adjustment and involve several sensory systems.
To appreciate the complexity of maintaining balance and orientation, imagine that you are waiting tables on a tour boat. You have a tray full of drinks to be delivered to a table on the other side of the rolling deck. Even as your mind is occupied with remembering customer orders, unconscious processes allow you to move about in a smooth and coordinated manner.
The apparently simple task of delivering drinks is supported by a truly complex sensorimotor process for controlling postural orientation and balance. As you cross the deck your brain rapidly processes sensory information and adjusts motor output to maintain your balance, the upright orientation of your head and trunk, and stable arms supporting the tray of full glasses. Before you reach out to place a glass on the table, your nervous system makes anticipatory postural adjustments to maintain your balance. Sudden unexpected motions of the boat evoke automatic postural responses that prevent falls. Somatosensory, vestibular, and visual information is integrated to provide a coherent picture of the position and velocity of the body in space and to generate and update motor commands that maintain balance and orientation.
Postural Equilibrium and Orientation Are Distinct Sensorimotor Processes
Postural equilibrium, or balance, involves active resistance to external forces acting on the body. The dominant external force affecting equilibrium on earth is gravity. Postural orientation is the positioning of body segments with respect to each other and to the environment. Depending on the particular task or behavior, body segments may be aligned with respect to gravitational vertical, visual vertical, or the support surface.
The biomechanical requirements of postural control depend on anatomy and postural orientation and thus vary with the animal. Nevertheless, in a variety of species the control mechanisms for postural equilibrium and orientation have many common features. The sensorimotor mechanisms for postural control are quite similar in humans and quadrupedal mammals even though their habitual stance is different.
Postural Equilibrium Requires Control of the Body’s Center of Mass
With many segments linked by joints, the body is mechanically unstable. To maintain balance the nervous system must control the position and motion of the body’s center of mass as well as the body’s rotation about its center of mass. The center of mass is a point that represents the average position of the body’s total mass. In the standing cat, for example, the center of mass is located in the trunk just rostral to the midpoint between forelimbs and hind limbs.
Although gravity pulls on all body segments, the net effect on the body acts through the center of mass. The force of gravity is opposed by the ground reaction force, which pushes upward against each foot. The net ground reaction force occurs at an imaginary point on the ground called the center of pressure (Box 41–1).
The location of the center of mass in the body is not fixed but depends on postural orientation. When you are standing upright, for example, your center of mass is located in the abdomen approximately 20 mm in front of the second lumbar vertebra. When you flex at the hips, however, the center of mass moves forward to a position outside the body.
Maintaining balance while standing requires keeping the downward projection of the center of mass within the base of support, an imaginary area defined by those parts of the body in contact with the environment. For example, the four paws of a standing cat define a rectangular base of support (see Figure 41–1). When a standing person leans against a wall, the base of support extends from the ground under the feet to the contact point between the body and the wall. Because the body is always in motion, even during stable stance, the center of mass continually moves about with respect to the base of support. Postural instability is determined by how fast the center of mass is moving toward the boundary of its base of support and how close the downward projection of the body’s center of mass is to the boundary.
Balance During Stance Requires Muscle Activation
Upright stance requires two actions: (1) maintaining support against gravity (keeping the center of mass at some height) and (2) maintaining balance (controlling the trajectory of the center of mass in the horizontal plane). Balance and antigravity support are controlled separately by the nervous system and may be differentially affected in certain pathological conditions.
Antigravity support, or postural tone, represents the tonic activation of muscles that generate force against the ground to keep the limbs extended and the center of mass at the appropriate height. A cat stands with its limbs in a semiflexed posture (see Figure 41–1) and its extensor muscles are tonically activated to prevent the joints from collapsing into flexion. In humans much of the support against gravity is provided by passive bone-on-bone forces in joints such as the knees, which are fully extended during stance, and in stretched ligaments such as those at the front of the hips. Nevertheless, antigravity support in humans also requires active muscle contraction, for example in ankle, trunk, and neck extensors. Tonic activation of antigravity muscles is not sufficient, however, for maintaining balance.
Both bipeds and quadrupeds are inherently unstable, and their bodies sway during quiet stance. Actively contracting muscles exhibit a spring-like stiffness that helps to resist body sway, but muscle stiffness alone is insufficient for maintaining balance. Likewise, stiffening of the limbs through muscle co-contraction is not sufficient for balance control. Instead, complex patterns of muscle activation produce direction-specific forces to control the body’s center of mass. Body sway caused by even subtle movements, such as the motion of the chest during breathing, is actively counteracted by the posture control system.
Automatic Postural Responses Counteract Unexpected Disturbances
When a sudden disturbance causes the body to sway, various motor strategies are used to maintain the center of mass within the base of support. In one strategy the base of support remains fixed relative to the support surface. While the feet remain in place the body rotates about the ankles back to the upright position (Figure 41–2A). In other strategies the base of support is moved or enlarged, for example by taking a step or by grabbing a support with the hand (Figure 41-2B).
Figure 41-2 Automatic postural responses keep the downward projection of the center of mass within the boundaries of the base of support.
A. One strategy for regaining balance is to bring the center of mass back to its origin on the base of support. When the platform on which a subject is standing is suddenly moved backward, the body sways forward and the projection of the center of mass moves toward the toes. During recovery the body actively rotates about the ankles, bringing the center of mass back to the original position with respect to the feet.
B. An alternative strategy enlarges the base of support to keep the center of mass within the base. A disturbance causes the subject to sway forward and the center of mass moves toward the boundary of the base of support. The base can be enlarged in two ways: taking a step and placing the foot in front of the center of mass to decelerate the body’s motion, or grabbing a support and thereby extending the base to include the contact point between the hand and support.
The center of pressure is defined as the origin of the ground reaction force vector on the support surface. For the body to be in static equilibrium, the force caused by gravity and the ground reaction force must be equal and opposite, and the center of pressure must be directly under the center of mass.
Misalignment of the center of pressure and center of mass causes motion of the center of mass. If the center of pressure is behind and to the left of the center of mass projection onto the base of support, for example, the body will sway forward and to the right (Figure 41–1).
Figure 41-1 The center of mass moves during stance but remains within the base of support. The base of support of the standing cat is defined by the points of contact of the paws on the support surface. The force caused by gravity passes through the center of mass in the trunk. The surface exerts an upward force against each paw, such that the ground reaction force vector originates in the center of pressure on the support surface. Although the paws remain in place, the centers of pressure and mass are always in motion as the cat sways.
When no external forces other than gravity are present, the center of pressure and ground reaction force reflect the net effect of muscles activated by the postural system to actively control the center of mass position and therefore balance.
Standing is never truly static. The center of pressure and center of mass are continually in motion and are rarely aligned, although when averaged over time during quiet stance they are coincident. The actual sway of the body during quiet stance is described by the trajectory of the center of mass, not the center of pressure.
Older views of motor control focused on trunk and proximal limb muscles as the main postural effectors. Recent behavioral studies show that any group of muscles from the neck and trunk, legs and arms, or feet and hands can act as postural muscles depending on the body parts in contact with the environment and the biomechanical requirements of equilibrium.
When studying the posture control system, scientists disrupt balance in a controlled manner to determine the subject’s automatic postural response. This response is described by the ground reaction force vector under each foot, the motion of the center of pressure, and the movements of the body segments. The electrical activity of many muscles is recorded by electromyography (EMG), which reflects the firing of alpha motor neurons that innervate skeletal muscle and thus provides a window into the nervous system’s output for balance control. The combination of all these measurements allows investigators to infer the active neural processes underlying balance control.
An automatic postural response to a sudden disturbance is not a simple reflex but rather the synergistic activation of a group of muscles in a characteristic sequence with the goal of maintaining equilibrium. The recruitment of a muscle during a postural response reflects the requirements of equilibrium rather than the change in the muscle’s length caused by the disturbance. For example, when the surface under a person is rotated in the toes-up direction, the ankle extensor (gastrocnemius) is lengthened and a small stretch reflex may occur. The postural response for balance recruits the antagonist ankle flexor (tibialis anterior), which itself is shortened by the surface rotation, while suppressing the stretch response in the gastrocnemius. In contrast, when the platform is moved backward the gastrocnemius is again lengthened but now it is recruited for the postural response, as evidenced by a second burst of EMG activity after the stretch reflex. Thus the initial change in length of a muscle induced by perturbation does not determine whether that muscle is recruited for postural control, and stretch reflexes are not the basis for postural control.
Automatic postural responses to sudden disturbances have characteristic temporal and spatial features. A postural response in muscles must be recruited rapidly following the onset of a disturbance. Sudden movement of the support surface under a standing cat evokes EMG activity within 40–60 ms (Figure 41–3). Humans have longer latencies of postural response (80–120 ms); the increased delay is attributed to the larger body size of humans and thus the greater signal conduction distances from sensory receptors to the central nervous system and thence to leg muscles. The latency of automatic postural responses is shorter than voluntary reaction time but longer than the stretch reflex.
Figure 41-3 Automatic postural responses have stereotypical temporal characteristics. Electromyographic (EMG) activity has a characteristic latency. Anterior motion of the platform evokes an EMG response in the hip extensor muscle (anterior biceps femoris) approximately 40 ms after the onset of platform acceleration. This latency is stereotyped and repeatable across subjects and is approximately four times as long as that of the monosynaptic stretch reflex. As the platform moves, the paws are carried forward and the trunk remains behind owing to inertia, causing the center of mass of the cat to move backward with increasing velocity with respect to the platform. The velocity of the center of mass peaks and then decreases as the horizontal component of the ground reaction force (GRFh) increases following muscle activation. The delay of approximately 30 ms between the onset of EMG activity and the onset of the active response reflects excitation-contraction coupling and body compliance. The automatic postural response extends the hind limb, propelling the trunk forward and restoring the position of the center of mass with respect to the paws.
Postural responses involving a change in support base, such as stepping, have longer latencies than those that occur when the feet remain in place. The longer time presumably affords greater flexibility in the response, for example the choice of foot to begin the step, the direction of the step, and the path of the step around obstacles.
Activation of postural muscles results in contraction and the development of force in the muscles, leading to torque (rotational force) at the joints. The net result is an active response, the ground reaction force, that restores the center of mass to its original position over the base of support (Figure 41–3). The delay between EMG activation and the active response, approximately 30 ms in the cat, reflects the excitation-contraction coupling time of each muscle as well as the compliance of the musculoskeletal system.
The amplitude of EMG activity in a particular muscle depends on both the speed and direction of postural disturbance. The amplitude increases as the speed of a platform under a standing human or cat increases, and it varies in a monotonic fashion as the direction of platform motion is varied systematically. Each muscle responds to a limited set of perturbation directions with a characteristic tuning curve (Figure 41–4).
Figure 41-4 Automatic postural responses have stereotypical directional characteristics. (Adapted, with permission, from Macpherson 1988.)
A. The gluteus medius muscle in the cat, a hip extensor and abductor, responds to a range of directions of motion in the horizontal plane. The EMG records shown here are from a cat standing on a platform that was moved in the horizontal plane in each of 16 evenly spaced directions. The gluteus medius muscle of the left hind limb was activated by motion in several directions (pink) and inhibited in the remaining directions (gray). The dashed vertical lines indicate the onset of platform acceleration. In the center is a polar plot of the amplitude of EMG activity during the automatic postural response versus the direction of motion; it represents a directional tuning curve for the muscle. EMG amplitude was computed from the area under the curve during the first 80 ms of the response.
B. Every muscle has a characteristic directional tuning curve that differs from that of other muscles, even if they have similar actions. The middle biceps femoris and cranial semimembranosus, for example, are both extensors of the hip.
Although individual muscles have unique directional tuning curves, muscles are not activated independently but instead are coactivated in synergies. The muscles within a synergy receive a common command signal during postural responses. In this way the many muscles of the body are controlled by just a few signals, reducing the time needed to compute the appropriate postural response (Box 41–2).
Automatic Postural Responses Adapt to Changes in the Requirements for Support
The set of muscles recruited in a postural response to a disturbance depends on the body’s initial stance. The same disturbance elicits very different postural responses in someone standing unaided, standing while grasping a stable support, or crouching on all four limbs. For example, forward sway activates muscles at the back of the legs and trunk during upright free stance. When the subject is holding onto a stable support, muscles of the arms rather than those of the legs are activated. When the subject is crouched on toes and fingers, muscles at the front of the legs and in the arms are activated (Figure 41–6A).
Figure 41-6 Automatic postural responses change with biomechanical conditions.
A. The backward movement of a platform activates different groups of muscles depending on initial stance. Gray stick figures show initial positions (upright unsupported, quadrupedal, or upright supported). The muscles activated in each postural response are shown in red. (Adapted, with permission, from Dunbar et al. 1986.)
B. When a subject stands on a narrow beam that is abruptly moved backward, the anterior muscles—abdominals (ABD) and quadriceps (QUAD)—are recruited to flex the trunk and extend the ankles, moving the hips backward (the hip strategy). When the subject instead stands on a wide platform that is moved backward, his posterior muscles—paraspinals (PSP), hamstrings (HAM), and gastrocnemius (GAS)—are activated to bring the body back to the erect position by rotating at the ankles (the ankle strategy). Muscles representative of different postural responses are highlighted in color. Dashed vertical line indicates onset of platform (or beam) acceleration.
C. Postural strategy adapts after the subject moves from the narrow beam onto the wide platform. On the beam the quadriceps are activated and the hamstrings are silent; after adaptation to the wide platform the reverse is observed. The transition from quadriceps to hamstrings occurs over a series of trials; the quadriceps activity gradually decreases in amplitude, whereas the hamstrings are activated earlier and earlier, until by trial eight quadriceps activity disappears altogether. Ankle and trunk muscles show similar patterns of adaptation. (Adapted, with permission, from Horak and Nashner 1986.)
Because postural responses are influenced by recent experience, they adapt only gradually to new biomechanical conditions. When forward sway is induced by backward motion of a platform on which a subject is standing, the posterior muscles of the ankle, knee, and hip are activated in sequence beginning 90 ms after the platform starts moving. This postural response, the ankle strategy, restores balance primarily by rotating the body about the ankle joints. However, when forward sway is induced by backward motion of a narrow beam, the anterior muscles of the hip and trunk are activated. This postural pattern, the hip strategy, restores the body’s center of mass by bending forward at the hip joints and counter-rotating at the ankles (Figure 41-6B).
When a subject moves from the wide platform to the narrow beam, he persists in using the ankle strategy in the first few trials. This strategy does not work when standing on the beam, and the subject falls. He then gradually, over several trials, switches to the hip strategy. Similarly, moving from the beam back to the platform requires several trials to adapt the postural response (Figure 41-6C).
Although sensory stimulation changes immediately after subjects move from the beam to the floor, the postural response adjusts gradually as it is tuned for optimal behavior by trial and error. Trial-to-trial changes in postural behavior generally occur at the subconscious level and involve updating of the body schema.
Anticipatory Postural Adjustments Compensate for Voluntary Movements
Voluntary movements themselves can destabilize postural orientation and equilibrium. Rapidly lifting the arms forward while standing, for example, produces forces that extend the hips, flex the knees, and dorsiflex the ankles, moving the body’s center of mass forward relative to the feet. The nervous system has advance knowledge of the effects of voluntary movement on postural alignment and stability and activates anticipatory postural adjustments, often in advance of the primary movement (Figure 41–7A).
Figure 41-7 Anticipatory postural adjustments precede voluntary movement.
A. The postural component of a voluntary arm pull increases in amplitude and lead time as the pulling force increases. In this experiment subjects were asked to pull on a handle attached to the wall by a wire. Subjects stood on a force plate and, at a signal, pulled rapidly on the handle to reach a specified peak force varying between 5% and 95% of maximum pulling force. Each pull was preceded by leg-muscle activation that produced a rotational force, or torque, about the ankle joints. The larger the pulling force, the larger and earlier was the ankle torque. Traces are aligned at the onset of the pulling force on the handle at time zero. (MPF, maximum pulling force.) (Adapted, with permission, from Lee, Michaels, and Pai 1990.)
B. Postural adjustments accompany voluntary movement only when needed. As in part A subjects were asked to pull on a handle fixed to a wall. Electromyogram (EMG) traces are aligned at time zero, the onset of activity in the arm muscle, biceps brachii (BIC). During unsupported stance the leg muscles—hamstrings (HAM) and gastrocnemius (GAS)—are activated prior to the arm muscle to prevent the body from rotating forward during the arm pull. The red arrow shows the onset of gastrocnemius activation, the brown arrow that of the biceps brachii. When the subject was supported by a rigid bar at the shoulder, the leg muscle activity was not necessary because the body could not rotate forward. Shaded areas indicate anticipatory postural responses and the initial arm muscle activation. (Adapted, with permission, from Cordo and Nashner 1982.)
C. During walking the trajectory of the center of mass (CoM) is controlled by foot placement. The body’s center of mass is between the feet, moving forward and from side to side as the subject walks forward. When the body is supported by only one leg, the center of mass is outside the base of support and moves toward the lifting limb. People do not fall while walking because the placement of the foot on the next step decelerates the center of mass and propels it back toward the midline. (Adapted, with permission, from MacKinnon and Winter 1993.)
Anticipatory postural adjustments are specific to biomechanical conditions. When a freely standing subject rapidly pulls on a handle fixed to the wall, the leg muscles (gastrocnemius and hamstrings) are activated before the arm muscles (Figure 41-7B). When the subject performs the same pull while his shoulders are propped against a rigid bar, no anticipatory leg muscle activity occurs because the nervous system relies on the support of the bar to prevent the body from moving forward. When the handle is pulled in response to an external cue, the arm muscles are activated faster in the supported condition than in the freestanding condition. Thus voluntary arm muscle activation is normally delayed when the task requires active postural stability.
Another common preparatory postural adjustment occurs when one begins to walk. The center of mass is accelerated forward and laterally by the unweighting of one leg. This postural adjustment appears to be independent of the stepping program that underlies ongoing locomotion. Similarly, a forward shift of the center of mass precedes the act of standing on the toes. A subject is unable to remain standing on his toes if he simply activates the calf muscles without moving his center of mass forward; he rises onto his toes only momentarily before gravity restores a flat-footed stance. Moving the center of mass forward over the toes before activating the calf muscles aligns it over the anticipated base of support and thus stabilizes the toe stance.
Locomotion, too, has an important postural component. During walking and running the body is in a constant state of falling as the center of mass moves forward and laterally toward the leg that is in the swing phase. The center of mass is within the base of support during walking only when both feet are on the ground, the double stance phase, and not at all during running. When one foot is supporting the body, the center of mass moves forward in front of the foot, always medial to the base of support. Falling is prevented during walking and running by moving the base of support forward and laterally under the falling center of mass. Postural equilibrium during gait relies on the appropriate placement of each step to control the speed and trajectory of the center of mass (Figure 41-7C). The nervous system plans foot placement several steps in advance using visual information about the terrain and surrounding environment (see Chapter 36).
Postural equilibrium during voluntary movement requires control not only of the position and motion of the body’s center of mass but also of the angular momentum about the center of mass. A diver can perform elaborate rolls and twists of the body about the center of mass while airborne although the trajectory of his center of mass is fixed once he leaves the board. During swimming and flying the water or air currents in addition to the body’s own movements may cause the body to pitch or roll about the center of mass. During voluntary movements postural adjustments control the body’s angular momentum by anticipating rotational forces.
Postural Orientation Is Important for Optimizing Execution of Tasks, Interpreting Sensations, and Anticipating Disturbances to Balance
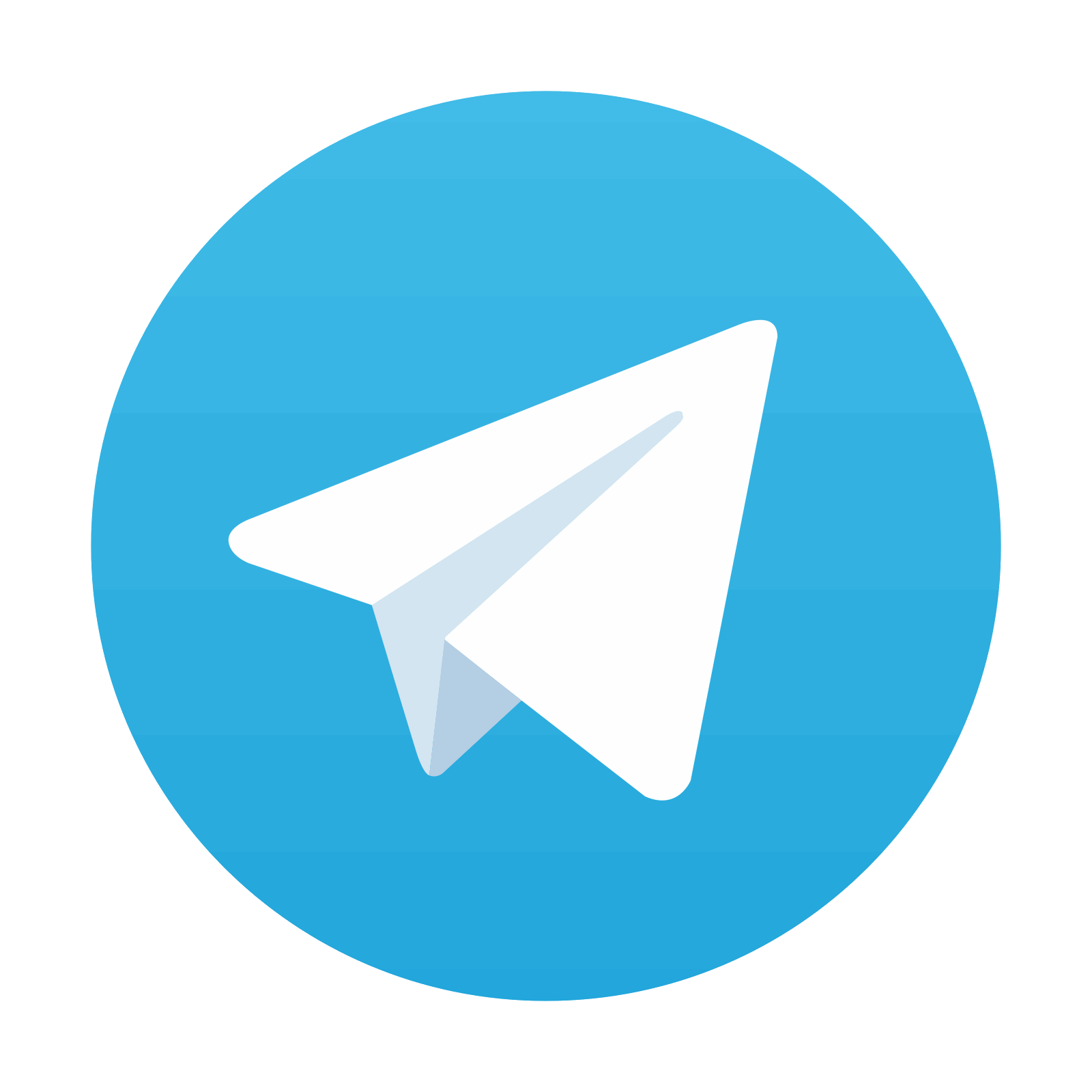
Stay updated, free articles. Join our Telegram channel

Full access? Get Clinical Tree
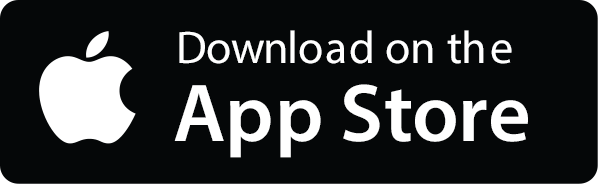
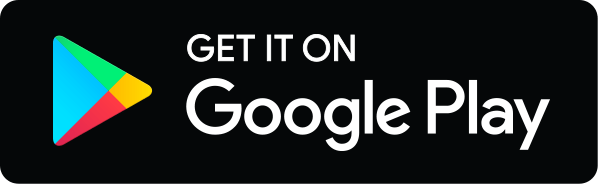