Chapter contents
Gravitational influences and muscles
Good posture and ‘asymmetrical normality’
Exteroceptive and proprioceptive postural controls
Common causes of postural imbalance and retraining options
Kuchera & Kuchera (1997) define the topic very simply: ‘Posture is distribution of body mass in relation to gravity over a base of support. The base of support includes all structures from the feet to the base of the skull’. They add that the efficiency with which weight is distributed over the base of support depends on the levels of energy needed to maintain equilibrium (homeostasis), as well as on the status of the musculoligamentous structures of the body. These factors – weight distribution, energy availability and musculoligamentous condition – interact with the (usually) multiple adaptations and compensations that take place below the base of the skull, all of which can influence the visual and balance functions of the body.
This chapter focuses on static as well as active postural features and how to assess some of these. Implicit in the evaluation of posture (and of gait, which forms the focal point of Chapter 3) is the way in which the body achieves and maintains its sense of balance, equilibrium and poise.
The CNS and brain receive an unceasing flood of data deriving from reporting stations. How this information is processed, and the instructions that flow to the tissues as a result, form the focus of the latter segment of this chapter. These issues are also more deeply examined in Volume 1, Chapter 3.
Static and dynamic posture
Posture (from positus, to place) is a word that is often used to describe a static state, the analysis of which is taken with the person remaining as still as possible. While this information undoubtedly offers inherent clinical value, when evaluating the individual the authors more often favor an approach in which dynamic, active, functional postural features are given priority, with the word ‘acture’ encompassing this concept (Hannon 2000a). Additionally, the use of several alternative and potentially insightful ways of assessing posture, many of which are discussed in greater depth later in this and other chapters, will add dimensions of information that simply cannot be gained from static evaluation alone. This point of view is not meant to detract from the extremely useful information gained from static observation, which can suggest to the practitioner the need for subsequent active movement and palpation assessments. An example of clues to further assessment that static postural observation offers will be found in Chapter 11.
Key postural influences
• Korr (1970) called the musculoskeletal system the ‘primary machinery of life’. By this he meant the functioning, ambulant, active features of the body, through which humans usually express themselves by doing, creating and generally functioning in the world, while interacting with society, the environment and with others. Korr distinguishes this ‘primary machinery of life’ (the locomotor system, the musculoskeletal system), in all its dynamic complexity, from the more (medically) glamorous ‘vital organs’, all of which subserve it, and allow it to act out the processes of being alive. Influences as diverse as gravity, emotion, visual integrity, central (brain) processing factors and the adaptations, which emerge as a result of the wear and tear of life, all influence the way this biomechanical marvel is carried and employed in space.
Kuchera (1997) states an osteopathic point of view when he highlights gravity as a key to understanding posture:
• A number of Kuchera’s insights are considered in this chapter.
• Latey (1996) discusses the patient’s presentation (or ‘image’) posture compared with the residual posture (as evidenced by palpation when he or she is resting quietly). He also draws attention to patterns of neuromuscular ‘tension’, which create postural modifications and which emerge from long-held emotional states, such as anxiety and depression. (Image posture and other Latey concepts are discussed later in this chapter.)
• Gagey & Gentaz (1996) offer insights as to neural input into the fine postural system and central integration of the virtually constant flow of information deriving from the eyes, the vestibular apparatus, the feet, etc. (The findings of Gagey & Gentaz in relation to proprioceptive input in particular, are expanded on later in this chapter.)
• Janda (1991) has shown that a central source of muscular imbalance (increased tension, for example, which inevitably results in postural changes) may result from limbic system dysfunction.
• The efficiency of postural balance depends (among other factors) on three sets of information reaching the brain for processing: visual, proprioceptive and vestibular. We automatically regulate how much importance to give one of these sources of information, over the others, when distorted data is being received – for example, when standing on an unstable surface that provides inaccurate visual information. Assessment as to visual efficiency can be achieved by testing balance with the eyes closed. (Redfern et al 2007).
• Redfern et al (2007) have also demonstrated that individuals with anxiety related disorders are most dependent on vision for balance, suggesting that reduced anxiety levels, potentially achievable via enhanced breathing patterns, relaxation methods, counseling or activities such as Tai Chi – might significantly improve balance (Gatts & Woollacott 2007).
• There appears to be a significant connection between cervical dysfunction, particularly involving the suboccipital extensor muscles (Gosselin et al 2004), and conditions such as osteoarthritis of the cervical spine (Boucher et al 2008), and disturbed balance.
• A variety of health conditions ranging from type 2 diabetes (with its tendency towards peripheral neuropathy) (Centomo et al 2007), and chronic low back pain (Lafond et al 2009), will affect balance negatively.
• Vleeming et al (1997, 2007) discuss ‘wear and tear’ – or ‘postural decay’ as they describe it – as the battle against gravity is slowly lost over a lifetime.
Gravitational influences and muscles
Kuchera (1997) cites Janda (1986) when he connects gravitational strain with changes of muscle function and structure, which lead predictably to observable postural modifications and functional limitations.
See Box 2.1 as well as Volume 1, Chapter 2 for a discussion of the postural and phasic muscles.
Box 2.1 Postural and phasic muscles
Among the more important postural muscles, which become hypertonic in response to dysfunction, are:
• trapezius (upper), sternocleidomastoid, levator scapula and upper aspects of pectoralis major, in the upper trunk, and the flexors of the arms
• quadratus lumborum, erector spinae, oblique abdominals and iliopsoas, in the lower trunk
• tensor fascia latae, rectus femoris, biceps femoris, adductors (longus, brevis and magnus), piriformis, hamstrings and semitendinosus in the pelvic and lower extremity region
Phasic muscles, which weaken in response to dysfunction (i.e. are inhibited), include:
• the paravertebral muscles (not erector spinae), scalenii and deep neck flexors, deltoid, the abdominal (or lower) aspects of pectoralis major, middle and inferior aspects of trapezius, the rhomboids, serratus anterior, rectus abdominis, gluteals, the peroneal muscles, vasti and the extensors of the arms
• muscle groups, such as the scalenii, are equivocal – although commonly listed as phasic muscles (this is how they start out in life), they can end up as postural ones if sufficient demands are made on them.
Richardson et al (1999) have published numerous study results showing which muscles are most involved in spinal postural stabilization:
Additionally, Hodges (1999) highlights the importance of the abdominal muscles as well as, perhaps surprisingly, the diaphragm in postural control. In a study (Hodges et al 1997) which measured activity of both the costal diaphragm and the crural portion of the diaphragm, as well as transversus abdominis, it was found that contraction occurred (in all these structures) when spinal stabilization was required (in this instance during shoulder flexion).
Disagreement
There is certainly no absolute agreement on which muscles provide ‘core stability’ (Figure 2.1). For example Grenier & McGill (2007), ask the question:
The involvement of the diaphragm in postural stabilization suggests that situations might easily occur where contradictory demands are evident – for example, where postural stabilizing control is required at the same time that respiratory functions create demands for diaphragmatic movement (shoveling snow, for example). Richardson et al (1999) state: ‘This is an area of ongoing research, but must involve eccentric/concentric phases of activation of the diaphragm.’
Dysfunction
Observable changes such as those illustrated in Figure 2.2A emerge through overuse, misuse, abuse and disuse of the postural system and demonstrate common dysfunctional postural patterns.
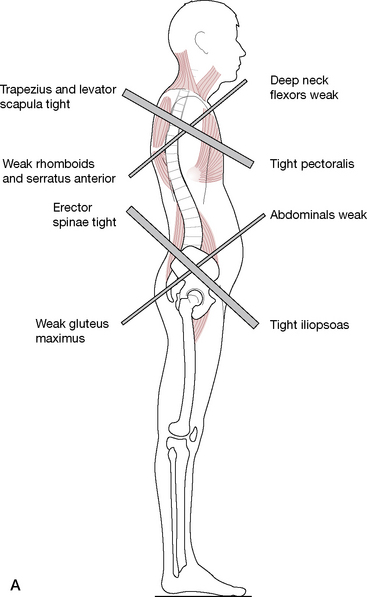
Figure 2.2 (A) The upper and lower crossed syndrome, as described by Janda
(adapted from Chaitow (1996)).
Variations on Janda’s original crossed patterns have been proposed by Key et al (2008), who have categorized the patterns based on characteristics. Two examples follow.
‘Posterior crossed pelvic pattern’ (see Fig. 2.2B)
• Pelvis – posterior shift + increased anterior sagittal tilt
• Trunk – anterior translation of thorax
Hypoactivity/lengthened muscles:
Hyperactivity/adaptive shortened muscles:
Trunk extension will be reduced; the thoracolumbar region will be hyperstabilized, leading to:
‘Anterior crossed pelvic pattern’ (see Fig. 2.2C)
• Pelvis – anterior shift + increased posterior tilt
• Trunk (thorax) backward loaded + lumbar spine flexed
• Hips in extension – tight posterior hip structures
• Atrophied buttocks, head forward, kyphosis, knees extended.
Hypoactivity/lengthened muscles:
• Lower abdominal group and pelvic floor
• Lumbar multifidus – particularly over lower levels
• Diaphragm – reduced excursion ++
Hyperactivity/adaptive shortened muscles:
Flexor muscles tend to dominate leading to:
Observation and assessment, as described in this text, can help to identify these common patterns.
The above examples clearly show value of the expansion of the work of one author by others. However, with the additional value may also come some inherent risks of degradation or potential confusion. The use of terms postural muscle and phasic muscle is a prime example and requires some elaboration. Box 2.1 lists some of the postural and phasic muscles, any of which (when stressed) may significantly contribute to postural misalignment. Box 2.2, as well as the following text, offers discussion of some of the confusing terms used to categorize these muscle types. An international nomenclature standard for postural terminology as well as for symbols used to record findings needs to be developed, published and adopted in order to avoid the confusion that develops when numerous groups each develop their own style.
Box 2.2 The muscle debate
Norris (2000) explains his perspective on the use of terms such as postural, phasic, stabilizer, mobilizer, etc. in categorizing muscles.
The terms postural and phasic used by Jull and Janda (1987) can be misleading. In their categorization, the hamstring muscles are placed in the postural grouping while the gluteals are placed in the phasic grouping. The reaction described for these muscles is that the postural group (represented by the hamstrings in this case) tend to tighten, are biarticular, have a lower irritability threshold and a tendency to develop trigger points. This type of action would suggest a phasic (as opposed to tonic) response and is typical of a muscle used to develop power and speed in sport for example, a task carried out by the hamstrings. The so-called ‘phasic group’ is said to lengthen, weaken and be uniarticular, a description perhaps better suited to the characteristics of a muscle used for postural holding. The description of the muscle responses described by Jull and Janda (1987) is accurate, but the terms postural and phasic do not seem to adequately describe the groupings. Although fiber type has been used as one factor to categorize muscles, its use clinically is limited as an invasive technique is required. It is therefore the functional characteristics of the muscle, which is of more use to the clinician. Stabilizing muscles show a tendency to laxity and an inability to maintain a contraction (endurance) at full inner range. Mobilizing muscles show a tendency to tightness through increased resting tone. The increased resting tone of the muscle leads to or co-exists with an inclination for preferential recruitment where the tight muscle tends to dominate a movement. The stabilizing muscle in parallel shows a tendency to reduced recruitment or inhibition as a result of pain or joint distension.
A further categorization of muscles has been used by Bergmark (1989) and expanded by Richardson et al (1999). They have used the nomenclature of local (central) and global (guy rope) muscles, the latter being compared to the ropes holding the mast of a ship. The central muscles are those that are deep or have deep portions attaching to the lumbar spine. These muscles are seen as capable of controlling the stiffness (resistance to bending) of the spine and of influencing intervertebral alignment. The global category includes larger more superficial muscles. Global muscles include the anterior portion of the internal oblique, the external oblique, the rectus abdominis, the lateral fibers of the quadratus lumborum and the more lateral portions of the erector spinae. …The local categorization includes the multifidus, intertransversarii, interspinales, transversus abdominis, the posterior portion of the internal oblique, the medial fibers of quadratus lumborum and the more central portion of the erector spinae. The global system moves the lumbar spine, but also balances/accommodates the forces imposed by an object acting on the spine.
Comerford & Mottram (2001a, b) have further refined the debate as to classification of muscles.
• Local stabilizers – are deep, monoarticular, maintain stability of joints in all ranges of movement; using local muscle stiffness to control excessive motion, particularly in neutral positions where capsular and ligamentous support is minimal. Local stabilizers include the deeper layer muscles which attach segmentally (i.e. spinally such as multifidi), and which increase activity before action to offer protection and support. Dysfunctionally there may be loss of efficient firing sequencing, with a tendency toward inhibition and loss of segmental control (for example, deep neck flexors). These muscles equate (more or less) with Janda’s phasic muscles. ‘Dysfunction of local stability muscles is due to alteration of normal motor recruitment contributing to a loss of segmental control.’ Therapeutic interventions should encourage and facilitate tonic activation and strength.
• Global stabilizers – are also monoarticular, more superficial than the local stabilizers, and lacking in segmental (spinal) attachments, inserting rather on the thorax or pelvis; they generate force and control ranges of motion orientation of which may be biased with functions relating torque; when dysfunctional there is likely to be reduced control of movement (for example, transversus abdominis). These equate (more or less) with Janda’s phasic muscles. ‘Dysfunction of the global stability muscles is due to an increase in functional muscle length or diminished low threshold recruitment.’ Therapeutic interventions should encourage and facilitate tonic activation and strength.
• Mobilizers are biarticular or multiarticular, superficial, provide long levers and are structured for speed and large movements. These equate with the postural muscles of Janda (for example, psoas). Dysfunctional patterns result in shortening (‘loss of myofascial extensibility’) and react to pain and pathology with spasm. ‘Dysfunction of the global mobility muscles is due to loss of functional muscle extensibility or overactive low threshold activity.’ Therapeutic interventions should encourage mobilization and lengthening.
Therapeutic objectives
Kuchera (1997) describes treatment goals which aim to establish attainable structural and functional goals and which need to be based ‘upon modifying underlying pathophysiology and biomechanical stressors’. Thus, when treatment incorporates therapeutic methods directed at local tissue biodynamics and when gravitational strain contributes to the underlying pathophysiology, strategies for systemic integration of postural alignment must also be incorporated.
Muscle categorizations
It is possible (to some degree) to categorize muscles by their primary functions, these being to maintain the body in a stable, posturally balanced state in its constant struggle with gravity, as well as providing the capacity for movement and action. Not only is the categorization of muscles useful when attempting to determine causes of dysfunction and in formulating a treatment and/or rehabilitation plan, it is also practical since there is a degree of predictability in the performance (and eventual pathophysiological response leading to dysfunction) of particular muscles when they are under stress (overuse, misuse, abuse, disuse). For instance, certain muscles tend to become weak when stressed (inhibited, hypomyotonic, ‘pseudoparetic’, hypotonic) while others will tend to develop a higher degree of tension (hypermyotonia, ‘tight’, hypertonic) and will ultimately shorten (Norris 2000).
Janda’s (1986) classification of muscles as ‘postural’ and ‘phasic’ (see Box 2.1) states that postural muscles become hypertonic (and subsequently shortened) in response to stress whereas phasic muscles become inhibited (‘weakened’, displaying what he terms ‘pseudo-paresis’) when similarly stressed. Janda’s classification of muscles has been challenged by some (for example, Norris 2000) who prefer descriptors such as ‘stabilizers’ and ‘mobilizers’ (where, somewhat confusingly, stabilizers are equated with the muscles which Janda classifies as phasic). Additional descriptors include ‘global’ and ‘local’, ‘superficial’ and ‘deep’, as well as monoarticular and polyarticular. Comerford & Mottram (2001a,b) have further refined muscle classification by defining particular muscles as local stabilizers, global stabilizers and global mobilizers.
While fully aware of the value of this debate regarding the pathophysiology of musculoskeletal structures and its potential to transform ideas and concepts, we have chosen to use Janda’s (1986, Jull & Janda 1987) descriptors (i.e. postural/phasic), which are (at this time) more widely familiar to readers and which are, as a result, probably less confusing. This decision to use Janda’s descriptors is not meant to deny the validity of other ways of classifying muscles nor to discourage of the expansion of Janda’s ideas by Norris and others. It is the authors’ belief that ultimately the names ascribed to the processes and structures involved are of less importance than the basic fact that, in response to stress (overuse, misuse, abuse, disuse), particular muscles have a tendency toward shortening – whatever the name or category given to them – while others have a tendency toward inhibition, weakness and sometimes lengthening. As this debate continues, it will be interesting to see what emerges when adequate research relating to muscle types, recruitment sequences and other details involving the pathophysiological responses of different muscles to the stresses of life provides critical data relevant to the ongoing controversy (Bullock-Saxton et al 2000).
Necessary assessments
• Muscular atrophy (for example, lumbar erector spinae, gluteus maximus).
• Hypertrophy (for example, thoracolumbar erector spinae, upper trapezius).
• Length (is shortening or lengthening apparent?).
• Strength (for example, gluteus medius in one-legged standing).
• Relative normality of muscular firing sequences (‘stereotypic movement patterns’ (Liebenson 2007)) when specific functions are performed (for example, hip extension and hip or shoulder abduction).
Static postural images
While ‘perfect posture’ is seldom seen in a clinical setting, an understanding of a standard or ‘ideal’ alignment is necessary in order to know when variations exist. Kendall et al (1993) note:
Petty (2006) has listed a variety of static postural patterns. These include the ‘ideal’ posture as described by Kendall et al (1993) and include:
• upper and lower crossed syndromes (Janda 1994a), in which particular muscles weaken and others shorten in response to stress (overuse, misuse, abuse, etc.), resulting in aberrant postural and use patterns that are easily recognized (Fig. 2.2A)
• kyphosis-lordosis posture (Kendall et al 1993) in which upper and lower crossed patterns (see Fig. 2.2A) are combined
• layer syndrome pattern (Jull & Janda 1987) in which patterns of weakness and shortness are viewed from a different perspective (Fig. 2.3)
• flat back and sway back postures (Kendall et al 1993), which have their own individual patterns of weakness and shortness, easily identified by tests and observation (Figs 2.4 & Fig. 2.5)
• handedness posture, which relates directly to being left- or right-handed, leading to particular overuse and underuse patterns (Fig. 2.6).
• Is it due to overuse? The patient’s history and descriptions of daily habits of use should provide information regarding this possibility.
• Or could there be reflexive activity due to joint blockage or other influences (such as viscerosomatic reflexes)? Careful evaluation of the history and symptoms, along with palpation and assessment, should provide evidence of joint restrictions and/or the likelihood of viscerosomatic influences.
• Or are trigger points active in these muscles or their synergists or antagonists? Careful evaluation of the symptom picture as well as neuromuscular evaluation and palpation for active triggers may confirm such a possibility.
• Or are neurological factors involved? Clinical evaluation, or referral to an appropriate practitioner may confirm or rule out such possibilities.
• Or is there a structural asymmetry (short leg, etc.) for which the soft tissue is compensating? Careful observation, palpation and assessment should offer answers to this question.
• Or is this apparently unbalanced adaptation caused by some ‘tight’ and some ‘loose’ musculature – the very best solution the body can find for habitual patterns of use (occupational or sporting demands, for example) or by congenital or acquired changes (short leg, arthritic change, etc.), which should be understood, rather than interfered with. Therapeutic solutions in such instances are often best addressed to the habits of use, rather than the adaptive changes.
• Despite not in itself providing clear answers to such questions, static postural assessment may provide indications that suggest the focus of further investigation. Static assessment forms an important part of postural evaluation and analysis and assists in the training and refining of vital observational skills.
Static postural assessment
While dynamic, moving postural assessment (e.g. gait analysis, observation of the body in action (acture), motion palpation, functional testing – see Chapter 3 in particular) has tremendous value, especially regarding functional movements and adaptation patterns, static postural assessment offers valuable information as well, primarily regarding structural alignment, shortened fascial planes, and balance. As each region of the body is assessed for its position, balance and ability to interface with other regions and the influences of existing dysfunctional patterns, a sense of the overall skeletal alignment as well as of soft tissue compensation patterns can offer insights as to possible causes of recurrent dysfunction and pain.
Tools of postural assessment
Postural grid
The patient stands in front of the grid during performance of the same type of basic postural analysis mentioned earlier (and discussed step by step within this chapter). This displays evidence of postural alignment in relation to the grid (anterior, posterior and lateral), which is photographically recorded or noted on a postural analysis form. A wall-mountable Postural Analysis Grid Chart has been developed by NMT practitioner David Kent (available for purchase*). A short version of a postural assessment protocol similar to the one described in this chapter is printed on the chart.
Computerized assessment methods
Digital videography, with analyzing software, also offers a sophisticated interpretation of findings. Multiple computer screen images can be viewed simultaneously, whether these are from several views at one session or from several sessions. Pretreatment and post-treatment views can be overlaid to emphasize changes in structural alignment deriving from the therapeutic intervention. An image of ‘before’ and ‘after’ standing or walking can be viewed side by side and can provide a powerful reinforcement for the patient of the value of treatment and rehabilitation strategies. See also discussion of Linn’s work (2000) with computerized images on p. 43.
Basic postural assessment
Although the movements are not actually for assessment purposes (and are not to be confused with stepping tests discussed on p. 48), the diversion of ‘doing something’ often distracts the patient sufficiently to allow a more relaxed posture to manifest. Foot positioning, such as occurs with habitual lateral rotation of the leg, often becomes more obvious after such movements. It should also be noted if, upon stopping, he then pulls the body ‘up’ into a better alignment, which might represent a conscious effort to ‘look good’ for the assessment.
Standing postural assessment (Fig. 2.7)
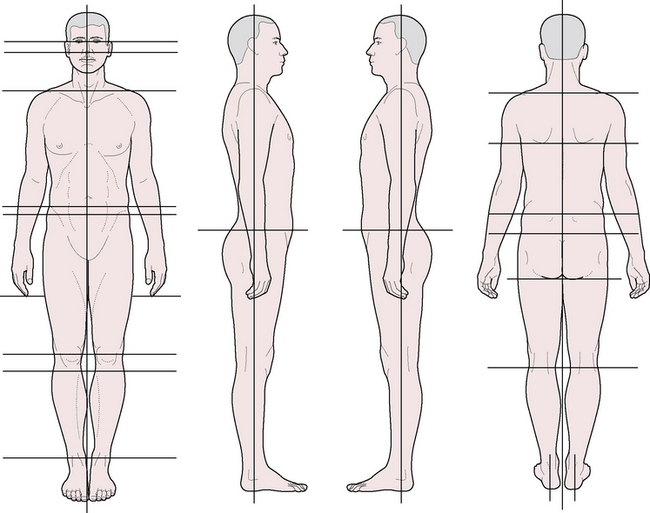
Figure 2.7 Postural evaluation recording form. This form may be photocopied for clinical or classroom use
(adapted from NMT Center lower extremity course manual (1994)).
Anterior view (Fig. 2.9)
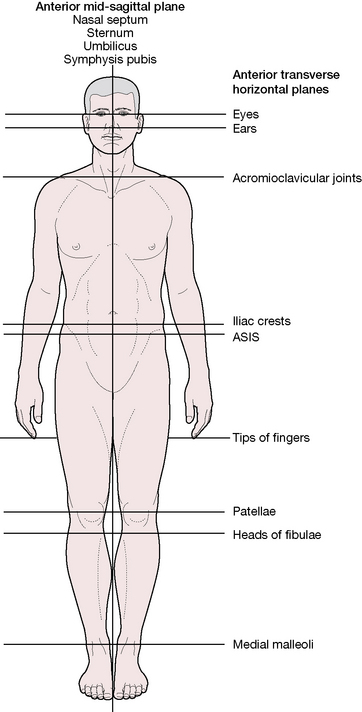
Figure 2.9 Primary landmarks evaluated in postural analysis, anterior view
(adapted from NMT Center lower extremity course manual (1994)).
Is the head held erect or does it tilt to one side or the other?
• If the head is off-set, pulling to one side or the other, the causes could relate to pelvic base unleveling (see Chapter 11), loss of planter arch integrity (see Chapter 14), compensation for spinal deviations or localized suboccipital/cervical/upper thoracic muscular imbalances
• Some degree of tilting may relate to asymmetrical occipital condyles, which is a common and normal occurrence
• Cranial or facial bone dysfunction (for example, involving dental malocclusion or TMJ problems) might lead to compensatory tilting of the head
• Visual or auditory imbalances/dysfunction can lead to an unconscious tendency to tilt or rotate the head.
• If one earlobe is lower than the other, the cause could be cranial distortion (particularly temporal bone) or head tilt (see previous question)
• If one earlobe is lower than the other, are heavy earrings customarily worn, especially in one ear only?
Does one ear, or do both ears, flair excessively from the head?
• If ears flare this might relate to cranial imbalance (involving external rotation of the temporal bones) (Upledger & Vredevoogd 1983).
Box 2.3 Cranial observation and assessment exercise (see Fig. 2.8)
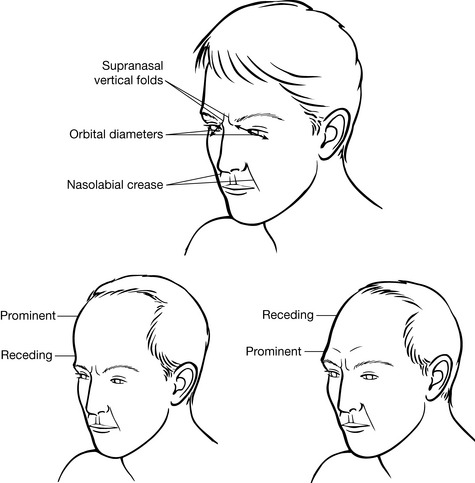
Figure 2.8 Examples of modifications of shape resulting from variations in cranial features
(reproduced, with permission, from Chaitow L 2000 Cranial Manipulation: Theory and Practice. Churchill Livingstone Edinburgh)
Observe the face of a patient for symmetry. Marked asymmetry may result from:
• cranial distortions involving the reciprocal tension membranes (e.g. falx cerebri, tentorium cerebelli) being distorted due to birthing difficulties such as forceps delivery
• physical trauma such as direct impact in motor accidents
• severe strains such as may occur with heavy dental extractions
• habitual one-sided chewing, often due to sensitive or missing teeth
• cranial imbalances, which may reflect generalized torsion patterns emerging from fascial stresses reflecting upwards from the lower body and trunk, into the cervical region and cranium (Upledger & Vredevoogd 1983).
• The orbit of the eye will be wider and the eye will be more prominent.
• The frontal bone will be more prominent.
• The nose may deviate to that side (but other factors including the status of facial bones, such as the maxillae, influence this).
General observation commonly reveals a range of asymmetries in the facial features, which may be interpreted as indicating underlying patterns of imbalance at the sutures of the skull, usually involving the intracranial fascial structures (reciprocal tension membranes). For a greater understanding of the underlying imbalances and their significance globally, texts by Milne (1995), Chaitow (2005) and Upledger & Vredevoogd (1983) are recommended. Features for which to observe include:
• relative narrowness or width of the head
• slope of the forehead – receding or prominent
• diameters and relative prominence of the orbits
• angle of deviation of the nose
• relative equality of width of nostrils
• relative equality of balance of cheekbones
• degree of flare or flatness of the ears to the head
• relative position of mastoid processes
• depth and angles of nasolabial creases and supranasal creases
• lack of centering of the upper and/or lower central incisors.
Are the eyes level and of similar dimensions?
Does palpation and/or observation of the cranium demonstrate any asymmetrical features?
Is the nose straight and centered, with symmetrical nasal apertures?
• If the contact point of the central incisors lies to one side of the mid-line this could indicate distortion of the maxillae as the intermaxillary suture (which lies between and above these two central teeth) should lie directly in the mid-line when the head is in neutral position.
• Distortion of the position of the maxillae could indicate other (possibly) more primary cranial distortions.
• This point represents the mid-line of the mandible and, if off center, could represent a cranial distortion or a deviated mandible due to disc displacement (TMJ), muscular imbalance of the masticatory muscles or trigger points within the masticatory muscles, including suprahyoid muscles.
• When considering such imbalances it is worth recalling that Janda (1994b) has shown that TMJ dysfunction can emerge as a result of overall postural imbalances commencing with the postural integrity of the feet, legs, pelvis and spine. Yuill & Howitt (2009) have associated TMJ pain and bilateral temporal headaches. They note that:
• Each of these muscles then provides tensional influences on the cranial bones, adding to further distortion.
• Lateral excursions of the mandible could indicate imbalances or trigger points within the masticatory muscles (including suprahyoid muscles, especially digastric), TMJ disc displacement or other intrajoint abnormality
• A non-smooth (jerky, clicking) opening pattern could indicate anterior articular disc displacement or other derangement, deformity of the articular disc, or the presence of trigger points or hypertonicity in masticatory muscles
• Any mandibular distortion could indicate a more primary distortion of other cranial bones (i.e. temporal bones into which the mandibular condyles seat).
Is the distance between the bottom of the earlobe and the top of the shoulder the same on each side?
• Cervical distortion due to biomechanical factors may result in such a deviation or an habitual head tilt might relate to visual or auditory imbalances
• An elevated shoulder could be due to postural compensation necessitated by a spinal scoliosis, pelvic distortion, leg length inequality, unilateral loss of the plantar arch or other structural deviation
• The apparently lower shoulder could be depressed by shortening or hypertonia involving shoulder muscles, such as latissimus dorsi
• Apparently higher or lower shoulder could be related to habitual unilateral loading of an upper extremity, such as when carrying a purse or luggage.
• If hypertrophy of upper trapezius exists (particularly if bilateral) this suggests the possibility of upper crossed syndrome imbalance with consequent inhibition of the lower fixators of the shoulder (Janda 1994a) (see Fig. 2.10 and discussion later in this chapter)
• Excessive bulk of the muscles of one shoulder may be due to habits, such as raising the shoulder to hold the phone to the ear or consistently carrying an object on that side
• Elevation of the first rib by hypertonic scalenii muscles could give the appearance of excessive trapezius bulk. This elevation might also impede lymphatic drainage, resulting in a ‘swollen’ appearance of the supraclavicular fossa region.
Are the acromioclavicular joints level with each other?
• The appearance of a ‘high shoulder’ could be due to excessive tension or trigger points of the ipsilateral trapezius or levator scapula
• The contralateral shoulder could be lowered by the latissimus dorsi or other shoulder muscles which are shortened or hypertonic
• This differential may also be a result of postural distortion or skeletal abnormality involving the lower extremity, pelvis or torso (scoliosis, fallen arch, pelvic obliquity, etc.)
• Assessment and treatment of acromioclavicular restrictions are covered in Volume 1, Chapter 13.
• If not, imbalance in the rotator cuff mechanism and/or global rotators of the shoulder (such as pectoralis major), and/or an imbalance between flexor and extensor muscle groups associated with the upper crossed syndrome (see later in this chapter) may be present (see Chapter 1 of this text and Volume 1, Chapter 4 for more details of this dysfunctional pattern).
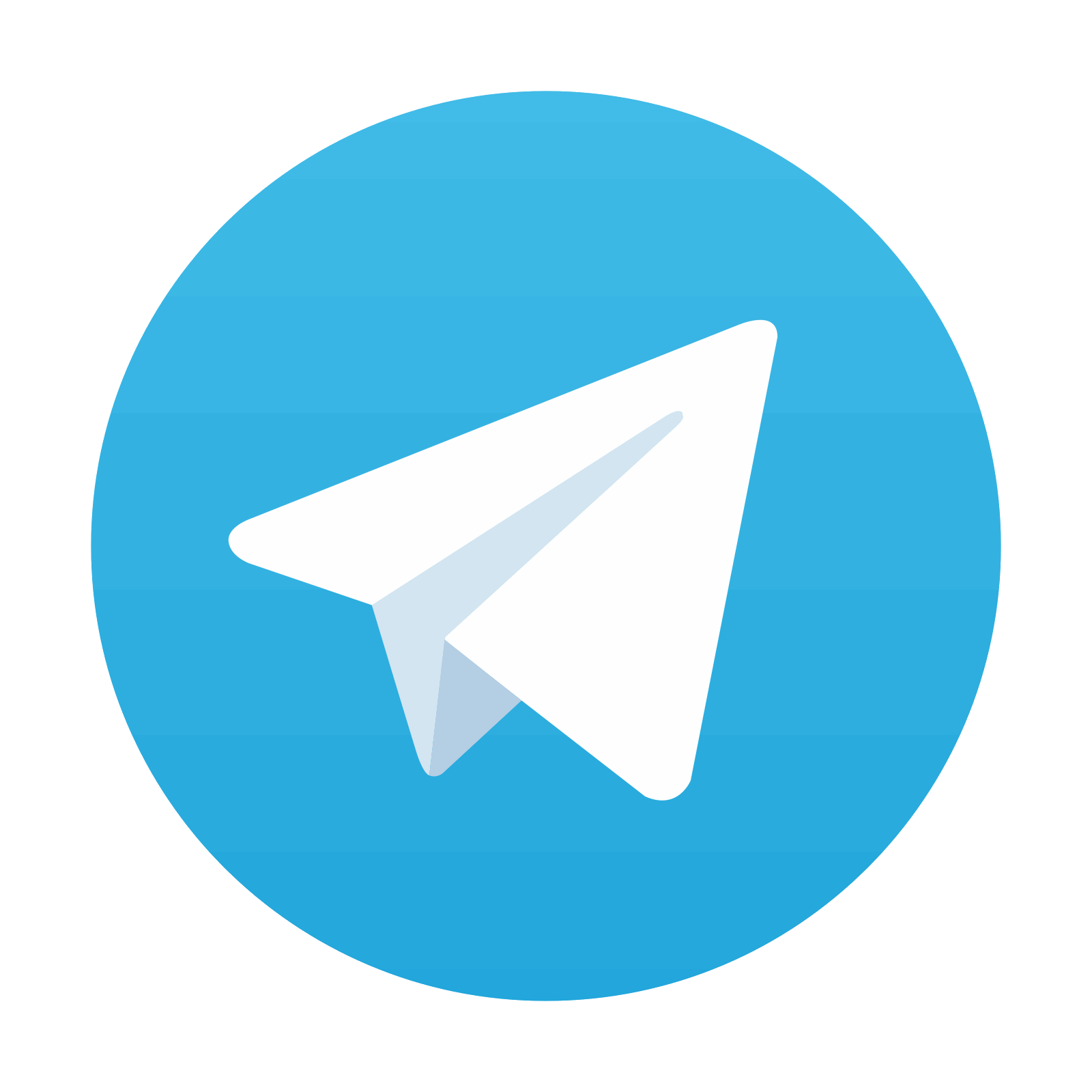
Stay updated, free articles. Join our Telegram channel

Full access? Get Clinical Tree
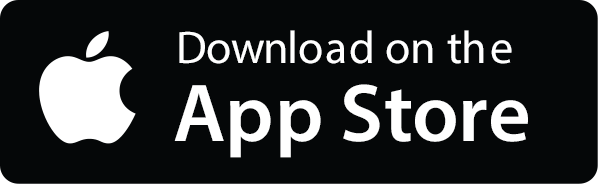
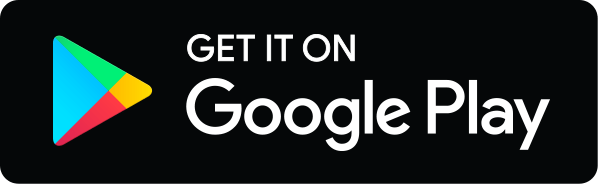
