Fig. 6.1
A simplified scheme of the Sonic Hedgehog signaling pathway. PTCH patched, SHH Sonic Hedgehog protein, SMO smoothened, SUFU suppressor of fused
Aberrant activity of the Shh signaling pathway has been implicated in the pathogenesis of at least a subset of medulloblastomas [52]. Approximately 25 % of medulloblastomas carry mutations in genes of the Hedgehog pathway, and inappropriate activation of Hedgehog signaling occurs in approximately 60 % of tumors. Overactivity of Hedgehog signaling during GCP development may be a primary event in medulloblastoma pathogenesis [52]. The association between the Hedgehog signaling pathway and medulloblastomas is also supported by animal models in which mice with PTCH gene mutations exhibit features of Gorlin syndrome and 14 % of them develop medulloblastoma [68, 69].
Medulloblastomas, especially of the desmoplastic subtype, are known to have loss of heterozygosity (LOH) on chromosome 9q, a region where the gene PTCH1 is located [70]. On the other hand, subsequent studies of sporadic medulloblastomas have shown that mutations of PTCH1 are not common among the sporadic tumors [71–73]. Sporadic medulloblastomas carrying LOH on chromosome 9q do not have readily detectable mutations of the other PTCH1 allele. Moreover, medulloblastomas from PTCH +/− mice retain the wild-type allele. These findings indicate that the lost of one allele of PTCH1 may be sufficient to promote medulloblastoma formation [72, 74].
Activating mutations of SMO have been detected in approximately 5 % of sporadic medulloblastomas [75]. Germline and somatic mutations in the gene, SUFU, were found in approximately 9 % of children with medulloblastoma [76] and within the desmoplastic subtype in particular.
6.3.2 The Wnt Signaling Pathway
The Wnt signaling pathway is composed of a highly conserved group of more than 20 secreted proteins that play a role during embryonic development, stem cell regulation, and cell differentiation [51]. The Wnt signaling pathway can be divided into four subtypes: the canonical or Wnt/β-catenin pathway and three noncanonical pathways, Wnt/Ca++, planar cell polarity, and Wnt/protein kinase A pathways [77].
The Wnt/β-catenin pathway has been well studied among these pathways, as sporadic colon cancer has shown to be frequently associated with mutations in this pathway [78]. Wnt protein is a secreted ligand that binds to its receptor, the seven-transmembrane protein Frizzled (FRZ) [79]. FRZ signaling is mediated by Dishevelled to a multiprotein complex composed of APC protein, axin protein, glycogen synthase kinase (GSK)-3β, and β-catenin. In the absence of Wnt signaling, the APC/axin/GSK-3β complex phosphorylates the amino-terminal residues of β-catenin, thereby leading to degradation of β-catenin by the ubiquitin-proteosome pathway. As a consequence, the level of cytoplasmic β-catenin is downregulated. The binding of Wnt protein to the receptor complex inhibits the kinase activity of this protein complex, leading to β-catenin accumulation in the cytoplasm. When β-catenin accumulates in the cytoplasm, it can translocate to the nucleus where it binds to transcription factors thereby inducing the expression of known oncogenes, such as c–myc and cyclin D [80–82] (Fig. 6.2). Accumulation of β-catenin in cells with APC mutations has been shown to result in strong overexpression of the c–myc gene [80]. The protein SUFU, already described as responsible for nuclear exportation of Glis in Hedgehog signaling, is also responsible for nuclear exportation of β-catenin in the Wnt signaling pathway. Thus, it plays a significant role in two important pathways of medulloblastoma formation [51, 66, 83] (Fig. 6.2).


Fig. 6.2
A simplified scheme of the Wingless (Wnt) signaling pathway. APC adenomatous polyposis coli, DSH Dishevelled, FRZ frizzled, GSK3β glycogen synthase kinase-3β, SUFU suppressor of fused
The identification of mutations of the tumor suppressor gene APC in the context of Turcot syndrome led to the recognition of the Wnt signaling pathway associated with medulloblastoma formation. Since then, several proteins related to this pathway have been studied to evaluate their participation in sporadic medulloblastomas [20, 55].
Immunohistochemical study showed that nuclear β-catenin was positive in 18 % of medulloblastomas, suggesting that Wnt signaling is overactive in about one-fifth of sporadic medulloblastomas [84]. β-Catenin was found to be overexpressed in the nucleus of medulloblastomas cells in 18–22 % of cases [85]. Activating mutations of β-catenin were also found in 3 of 67 sporadic medulloblastomas [86]. These data suggest that overactivity of the Wnt signaling pathway may contribute to the pathogenesis of sporadic medulloblastoma.
Although APC germline mutations are associated with medulloblastoma in Turcot syndrome, previous studies have failed to show LOH at the locus of APC and any somatic mutations in sporadic medulloblastomas [73, 87]. These results contrast with those found in sporadic colon cancer, where mutations of APC occur in almost 80 % of the cases. However, more resent study has shown missense mutations of APC gene in 2 of 46 sporadic medulloblastomas [88].
Mutational analysis of the axin gene has shown mutations in seven of 97 medulloblastomas including 86 sporadic cases. These mutations were primarily located at the APC-binding domain, possibly decreasing the ability of axin to bind APC, an interaction which is required to downregulate β-catenin [89]. Although GSK-3β also acts as a downregulator of the Wnt signaling pathway, mutational analysis has failed to show any mutations of this gene in a series of medulloblastomas [86].
6.3.3 The Notch Signaling Pathway
The Notch signaling pathway plays a general role in regulating cell fate choices and differentiation in several cells and tissues during development. In mammals, five ligands (Delta-like-1, Delta-like-3, and Delta-like-4 and Jagged-1 and Jagged-2) and four transmembrane receptors (Notch1 to 4) have been identified in this signaling pathway [20, 56, 90].
The Notch receptor is a large single-pass transmembrane protein consisting of an extracellular domain responsible for ligand binding and a cytoplasmic domain. When activated by the binding of the ligand which is displayed on an adjacent cell, the Notch receptor undergoes two proteolytic cleavage processes to function. The first cleavage occurs at the extracellular portion mediated by an extracellular protease. The second cleavage is mediated by a γ-secretase complex at the transmembrane site, releasing the cytoplasmic domain of the receptor from the membrane, that is, the intracellular form of Notch (icN). The icN translocates to the nucleus where it forms a complex with the ubiquitously expressed transcription factor CSL (CBF/Suppressor of Hairless/LAG-1) and activates the transcription of target genes (such as p21, cyclin D1 and Hes) and regulators of apoptosis [90] (Fig. 6.3).


Fig. 6.3
A simplified scheme of the Notch signaling pathway. CSL CBF/Suppressor of Hairless/LAG-1 transcription factor, icN intracellular Notch, TACE TNF α converting enzyme-like protease
Unlike the Shh and Wnt signaling pathways, no hereditary syndromes have been described associated with the Notch signaling pathway. Thus, its participation in medulloblastoma pathogenesis has not been well defined. However, recent analysis of embryonal brain tumor cell lines including medulloblastomas has shown that Notch2 was amplified in 15 % of cases [91]. In contrast, another study of medulloblastomas showed Notch1 was overexpressed in a majority of tumors, whereas Notch2 expression was elevated only in a small number of cases [92]. The expression of the Notch target genes Hes1 and Hes5 has also been shown to be elevated in medulloblastomas [92]. Furthermore, Notch signaling inhibition with γ-secretase inhibitors induced a marked reduction of tumor cell growth [92]. Further studies are needed to elucidate the exact role of the Notch signaling pathway in medulloblastoma formation, but these studies indicate that dysregulation of the Notch signaling pathway may contribute to the pathogenesis of medulloblastoma.
6.3.4 The EGFR Signaling Pathway
The epidermal growth factor receptor (EGFR) signaling pathway, comprising the EGFR family of receptor tyrosine kinases and their cognate ligands, is an evolutionarily conserved pathway that plays a crucial role in cell proliferation, survival, migration, and differentiation. Aberrant activation of this signaling pathway, in turn, leads to increased cell proliferation and altered cell migration through the activation of transcription factor target proteins.
The EGFR family are composed of four distinct transmembrane receptors; ErbB1, ErbB2, ErbB3, and ErbB4 (also known as EGFR, HER2, HER3, and HER4, respectively). These receptors consist of an extracellular domain responsible for ligand binding and an intracellular domain which possesses enzymatic activity [93]. The binding of ligands to the receptor induces receptor dimerization and cross-phosphorylation leading to activation of downstream signaling cascades such as phosphoinositide 3-kinase (PI3K), mitogen-activated protein kinase (MAPK), and protein kinase B (Akt) [94] (Fig. 6.4).


Fig. 6.4
A simplified scheme of the hepatocyte growth factor (HGF)/cMET and the epidermal growth factor (EGF) signaling pathways. AKT protein kinase B, ERK extracellular signal-regulated kinase, HGFA HGF activator, PI3K phosphoinositide 3-kinase
This EGFR signaling pathway has recently been implicated in the development and growth of several types of tumors including medulloblastoma. According to an immunohistochemical study, overexpression of the ErbB2 receptor was seen in 28 % of medulloblastomas [95], and coexpression of ErbB2 and ErbB4 was linked to unfavorable prognosis in the pediatric population [96]. Expression of ErbB2 and ErbB4 in association with a ligand of neureglin 1-β has been implicated in the metastatic behavior of medulloblastoma [97]. In addition, mutations of ErbB4 and upregulation of Akt and MAPK have also been described in association with medulloblastomas [98–100].
6.3.5 The HGF/cMET Signaling Pathway
The hepatocyte growth factor (HGF)/cMET signaling pathway plays a crucial role in diverse processes including development and tissue hemostasis. It promotes mitogenesis, morphogenesis, and cell motility in a variety of cell types including neurons [101]. HGF is a widely expressed multifunctional growth factor and serves as a ligand in this signaling pathway. HGF is produced in an inactive proform and then cleaved into its active heterodimer by the serine protease HGF activator (HGFA) [102, 103]. cMET is a transmembrane protein and the only receptor for HGF. The cytoplasmic domain of cMET has tyrosine kinase activity and is responsible for downstream signaling. The cMET receptor is normally activated by the binding of HGF. Upon binding of HGF, cMET undergoes autophosphorylation and recruits intracellular adapters which leads to the activation of downstream signaling such as Ras/MAPK, PI3K/Akt, and STAT pathways [101, 104–108] (Fig. 6.4).
Precise regulation of the HGF/cMET signaling is crucial for normal development. Several different mechanisms are involved in appropriate spatiotemporal expression of the cMET receptor along with HGF. In addition, the activity of HGFA which mediates HGF to activate is also tightly regulated. To date, two inhibitors of HGFA have been identified: SPINT1 and SPINT2 [109, 110]. These inhibitors prevent the HGF ligand from being activated, consequently leading to repression of the HGF/cMET signaling. Consequently, loss of inhibition due to reduced SPINT1 or SPINT2 expression may result in aberrant activation of the HGF/cMET signaling [111]. Aberrant overexpression of ligand or receptor may, in turn, upregulate HGF/cMET signaling [101]. Receptor amplification, activating mutations, or gene rearrangements may also increase HGF/cMET signaling [101, 111, 112].
Aberrant activation of HGF/cMET signaling has been implicated in promoting tumorigenesis and the metastatic phenotype, and several cMET activating mutations have been identified in a variety of cancers [111]. A recent study which examined primary medulloblastomas by comparative genomic hybridization (CGH) has detected cMET gene copy number gains in 38.5 % of their cases [113]. High expression of cMET has been correlated with reduced overall survival [114]. Treatment of medulloblastoma cell lines with HGF increased cell proliferation and anchorage-independent growth and reduced apoptosis [114]. Following HGF treatment, the medulloblastoma cell lines exhibited a more malignant phenotype than the parental cell line [114]. Given these results, it is reasonable to hypothesize that the HGF/cMET signaling pathway may be involved in the pathogenesis of medulloblastoma.
6.4 Molecular Genetics of the Posterior Fossa Tumors in Children Other Than Medulloblastomas
6.4.1 Ependymomas
Ependymomas are the third most common pediatric brain tumor and account for 6.4 % of primary brain tumors in children aged 0–14 years and for 30 % of the tumors in children younger than 3 years [115]. Ependymomas can arise in three distinct locations: the spinal cord, supratentorial compartment, and the posterior fossa. Over 90 % of pediatric ependymomas arise intracranially with approximately 70 % of cases occurring in the posterior fossa. Interestingly, ependymomas located in these different anatomic sites are histologically indistinguishable. However, it is now known that different molecular genetic alterations occur in ependymomas depending on their site of origin.
Early cytogenetic and CGH studies which identified specific chromosomal aberrations exclusively in specific anatomical locations gave some indication that this might not be the case [116–118]. These reports were limited by the low resolution of techniques employed and small number of tumors analyzed from different locations and age of tumor onset. More recent studies on a larger number of tumor samples at higher resolution have demonstrated that ependymomas arising in different anatomical compartments are characterized by distinct copy-number alterations and genetic signatures [119]. Chromosome 22 loss has been reported to be the most common copy-number alteration in both sporadic intracranial and spinal ependymomas. LOH and gene mutation point to NF2 as a likely candidate in spinal ependymomas, but a chromosome 22 tumor suppressor gene, important in the case of pediatric intracranial ependymomas, remains unclear. The other frequent chromosomal abnormalities include losses on chromosomes 6q, 17p, and 22q and gains on 1q and 9q. Less frequent genetic alterations involve losses on chromosomes 7p, 9p, 10q, 13q, and 16q and gains on 4q, 5, 7, 8, 12q, 15q, 18, and 20. Mutational analysis used to find pathogenetically significant genes within regions of genetic alteration has yet to uncover causal targets, as somatic mutations in NF2 (22q), TP53 (17p), and PTEN (10q) are rare in childhood intracranial ependymoma [120, 121].
Recently, several publications have identified previously unknown focal amplifications of MYCN, EGFR, NOTCH1, NOTCH4 VAV1, and YAP1 and deletion of CDKN2A and SULT4A1 [119, 122–124]. Importantly, most pediatric ependymomas occur in the posterior fossa, and half of these cases demonstrated a balanced genomic profile with few detectable chromosomal abnormalities [121]. Modena et al. identified frequent deletion of chromosome 22q13.3 harboring a downregulated SULT4A1 and amplification and overexpression of YAP1 on chromosome 11q22 [122]. More recently, chromosome 9q33–34 was shown to be gained frequently in 30 % of ependymomas. Within this gene-rich locus, NOTCH1 was shown to be a candidate gene and found to be overexpressed and mutated in a subset of ependymomas. These mutations occur in approximately 8.3 % of cases exclusively in ependymomas of the posterior fossa. Taylor et al. demonstrated that approximately 12 % of the genes amplified in ependymoma were overexpressed, compounding the difficulty in identifying potential oncogenes [119].
Despite the above limitations, our understanding of the genetic basis of pediatric ependymomas has advanced remarkably through gene expression profiling. By analyzing a cohort of ependymomas of different anatomic origin, Taylor et al. found that histologically identical, but genetically distinct, ependymomas exhibit patterns of gene expression that recapitulate those of radial glia cells in the corresponding region of the central nervous system [119]. Cancer stem cells isolated from ependymomas displayed a radial glia phenotype and formed tumors when transplanted into the intracranial compartment in mice. These findings suggest that ependymomas might arise within the radial gial cell lineage, and identify restricted populations of radial glia cells as candidate stem cells of the different subgroups of ependymoma. They also support a hypothesis that subgroups of the same histological tumor type are generated by different populations of progenitor cells in the tissues of origin.
6.4.2 Pilocytic Astrocytomas
PA is the most common cerebellar neoplasm in childhood. Most of these tumors arise in the cerebellum and generally are associated with a favorable outcome except tumors close to brainstem [125]. Patients with autosomal dominantly inherited neurofibromatosis type 1 (NF1) are at higher risk of developing low-grade astrocytomas than the general population, often along the optic pathways [126]. Neurofibromin, the gene product of the NF1 gene, physiologically contributes to growth arrest of astrocytic cells and neuronal differentiation by downregulation of the MAPK signaling pathway via its GTPase-activating domain. Loss of neurofibromin expression conversely leads to increased Ras activity and astrocyte proliferation [127]. Similar activation of the MAPK pathway has been confirmed by gene expression profiles of both NF1-associated and sporadic PAs, suggesting activated MAPK signaling in most astrocytomas [128]. Even though activating mutations in KRAS was previously identified and those mutations results in activation of MAPK signaling, in some sporadic PAs, alternative mechanisms of oncogenic MAPK activation in PA have not been clear yet [129].
Most PAs have a normal karyotype, and cytogenetic abnormalities may be more common in adult comparing to pediatric PAs. The presence of cytogenetic mutations may indicate tumor progression. Among the few recurrent aberrations, trisomy of chromosomes 5 and 7 and gain of 7q were the most consistent findings [130, 131]. Other chromosome aberrations are extremely rare, and cytogenetic abnormalities of chromosomes 6, 8, 11, 12, 17, 19, and 22 were described in only a few cases [130, 132–134]. With increased resolution of array-based DNA copy-number analysis in recent years, it has become possible to identify more discrete aberrations in PA, such as duplication at chromosome band 7q34.
Recently, Pfister et al. identified BRAF as a centrally important oncogene in the pathogenesis of pediatric PAs [134]. More than 50 % of PAs displayed a tandem duplication of the BRAF locus (7q34), and approximately 6 % of the remaining nonduplicated tumors showed an activating mutation of BRAF at codon 600 in exon 15, indicating that different mechanisms of oncogenic BRAF activation exist in these tumors. Several successive studies found a similar or even higher frequency of BRAF duplications in PA [133, 135–137].
Breakpoints in the BRAF gene and a previously uncharacterized gene, KIAA1549, led to the formation of an oncogenic fusion transcript incorporating the BRAF kinase domain and lacking the autoinhibitory domain of wild-type BRAF, thus rendering it constitutively active [136, 137].
More recently, another mechanism of MAPK activation in PA was described, namely, tandem duplications at 3p25 in a small percentage (2 %) of these tumors, leading to an in-frame oncogenic fusion between SRGAP3 and RAF1, which shares high sequence homology with BRAF [132, 138]. In addition, one of the groups described a novel insertion at codon 598 in BRAF that mirrors the hotspot V600E mutation, resulting in a transforming, constitutively active BRAF kinase [138]. Using combined molecular analysis of the BRAF-KIAA1549 fusion and mutational analysis of IDH1, PA can be sensitively and very specifically differentiated from diffuse astrocytoma. BRAF fusion is identified in at least 70 % of PAS, but neither IDH1 nor IDH2 mutations are present in PAs, whereas BRAF fusion occurs rarely in pediatric diffuse astrocytoma [134] and has never been found in adult diffuse astrocytoma [139].
6.4.3 AT/RTs
AT/RTs are aggressive embryonic tumors that usually are diagnosed in children under the age of 2 years. Approximately 30 % of AT/RTs occur infratentorially, and these tumors are also seen in the kidney and less commonly in a variety of locations in the soft tissue. The prognosis is significantly poor.
Rhabdoid tumors have a similar molecular origin regardless of their anatomical locations. Mutation or deletion of both copies of the hSNF5/INI1 gene that maps to chromosome 22q11.2 is observed in approximately 70 % of primary tumors. Another 25 % of tumors have reduced expression at the RNA or protein level, indicative of a loss-of-function event. Deletions on chromosome 11 have also been found in rhabdoid tumors [140–145].
Versteege et al. identified the rhabdoid tumor suppressor gene on chromosome 22q11 as the hSNF5/INI1 (human sucrose non–fermenting 5) gene. This gene was biallelically inactivated by deletions and/or truncating mutations in a series of renal rhabdoid tumors [146]. This gene is highly conserved from humans to yeast, where it affects the yeast cell’s ability to metabolize sucrose when mutated. Biegel and colleagues showed somatic deletions and/or truncating mutations of hSNF5/INI1 in CNS AT/RTs. Subsequently, hSNF5/INI1 mutations were also found in choroid plexus carcinomas (CPCs) and possibly some primitive neuroectodermal tumors and medulloblastomas [147, 148]. Most mutations were found in tumors from very young children (age <2 years).
Some children with rhabdoid tumors have de novo germline mutations of hSNF5/INI1, suggesting that single mutation in the hSNF5/INI1 gene was predisposed to develop rhabdoid tumors. In some families with an elevated incidence of rhabdoid tumors and/or CPCs, there may be an inherited germline mutation of the hSNF5/INI1 gene that predisposes affected family members to cancer [149]. Subsequent generation of SNF5/INI1 knockout mice by homologous recombination has shown that SNF5/INI1 is important for development as hSNF5/INI1 −/− embryos die at embryonic day 7 [150, 151]. Furthermore, heterozygous SNF5/INI1 +/− mice develop tumors reminiscent of rhabdoid tumors, highly suggesting that it is also a tumor suppressor gene in mice, as well as providing an excellent model of the disease.
hSNF5/INI1 is almost ubiquitously expressed in both time and space in most tissues. It functions to regulate the structure of DNA to either allow or deny access of transcription factors to their respective promoters. hSNF5/INI1 can promote expression of some genes while repressing others [152]. hSNF5/INI1 interacts with the well-known proto-oncogene c-myc and may be involved in the c-myc mediated activation of apoptotic genes [153]. hSNF5/INI1 may also be involved with regulation of the highly important tumor suppressor gene RB1 (retinoblastoma susceptibility gene).
The INI1 protein is a component of the SWI/SNF chromatin-remodeling complex. The complex is recruited to promoters of a large variety of genes involved in cell signaling, growth, and differentiation [145, 154, 155]. It is uncertain why loss of function of this highly conserved, ubiquitously expressed protein would induce the specific phenotypes in both humans and mice. Understanding the role of INI1 as a member of the SWI/SNF complex in tumorigenesis will be important for identifying downstream target genes [145].
6.4.4 Choroid Plexus Papillomas
Primary CP tumors are the most common brain tumors in the first year of life. According to the 2007 WHO classification of tumors of the nervous system, these tumors are classified as CP papilloma (CPP, WHO grade I), atypical CPP (grade II), and CP carcinoma (CPC, grade III). While the patients with a CPP can be usually cured by surgical resection only, the prognosis of patients with a CPC is very poor.
CP tumors have been reported in families with germline mutations of TP53 or hSNF5/INI1, while TP53 mutations in sporadic CPCs are rare [149, 156–159]. Constitutional duplication of chromosome 9p is a rare anomaly with some of the patients reported having proliferative disorders of the CP [160]. Investigation of sporadic CP tumors showed extra copies of 9p further implicating genes on this chromosome arm in the pathogenesis of CP tumors. Overexpression of the SV40 Large T antigen oncogene in transgenic mice results in the formation of intracranial CP tumors [161, 162]. Carlotti et al. have shown overexpression of cell cycle gene products, such as pRB, cyclins, and E2F-1, in CPCs vs. CPPs. Furthermore, the MIB-1 labeling index of CPCs was significantly higher than that of the CPPs [163]. CP tumors were induced by the introduction of constitutively active Notch3 into periventricular cells of embryonic day 9.5 mice. These findings suggest that Notch signaling is important in this experimental model [164], while the significance of Notch signaling in human CP tumors remains unclear.
6.5 Conclusions
Recent technological advances and increased knowledge of molecular biology have brought us a better understanding of the molecular genetics of brain tumors in children as well as those in adults. As some examples, molecular genetic analyses of hereditary syndromes have revealed several specific gene mutations and signaling pathways involved in the pathogenesis of certain pediatric brain tumors, especially medulloblastoma. Many sporadic tumors exhibit frequent, nonrandom cytogenetic alterations which are coming into greater focus given the widespread availability of advanced genetic sequencing techniques. These molecular genetic approaches have provided new insights into the major genes and pathways that appear to initiate a wide variety of pediatric brain tumors.
Although the translation of our understanding of the molecular genetics of brain tumors into a tangible benefit to the individual child is still some distance away, in some cases, our knowledge of the genetics of pediatric brain tumors is beginning to assist us in categorizing stages of disease, prognosticating outcome after therapy, and suggesting new targets for future therapy all the time. We anticipate that personalized therapies based of the molecular diversity of each patient’s tumor will be feasible in the near future, which may have the potential to improve the outcome of a child with a malignant brain tumor.
Acknowledgements
This work was supported by b.r.a.i.n.child, the Wiley Fund, and the Pediatric Brain Tumor Foundation of the United States.
References
1.
Knudson AG Jr (1971) Mutation and cancer: statistical study of retinoblastoma. Proc Natl Acad Sci U S A 68:820–823PubMedCentralPubMed
2.
Cavenee WK, Hansen MF, Nordenskjold M, Kock E, Maumenee I, Squire JA, Phillips RA, Gallie BL (1985) Genetic origin of mutations predisposing to retinoblastoma. Science 228:501–503PubMed
3.
Dunn JM, Phillips RA, Becker AJ, Gallie BL (1988) Identification of germline and somatic mutations affecting the retinoblastoma gene. Science 241:1797–1800PubMed
4.
Raffel C (2004) Medulloblastoma: molecular genetics and animal models. Neoplasia 6:310–322PubMedCentralPubMed
5.
Taylor MD, Mainprize TG, Rutka JT (2000) Molecular insight into medulloblastoma and central nervous system primitive neuroectodermal tumor biology from hereditary syndromes: a review. Neurosurgery 47:888–901PubMed
6.
Strahm B, Malkin D (2006) Hereditary cancer predisposition in children: genetic basis and clinical implications. Int J Cancer 119:2001–2006PubMed
7.
Ng D, Stavrou T, Liu L, Taylor MD, Gold B, Dean M, Kelley MJ, Dubovsky EC, Vezina G, Nicholson HS, Byrne J, Rutka JT, Hogg D, Reaman GH, Goldstein AM (2005) Retrospective family study of childhood medulloblastoma. Am J Med Genet A 134:399–403PubMed
8.
Taggard DA, Menezes AH (2000) Three choroid plexus papillomas in a patient with Aicardi syndrome. A case report. Pediatr Neurosurg 33:219–223PubMed
9.
Hamano K, Matsubara T, Shibata S, Hirano C, Ito Z, Ase Y, Kusakari J, Takita H (1991) Aicardi syndrome accompanied by auditory disturbance and multiple brain tumors. Brain Dev 13:438–441PubMed
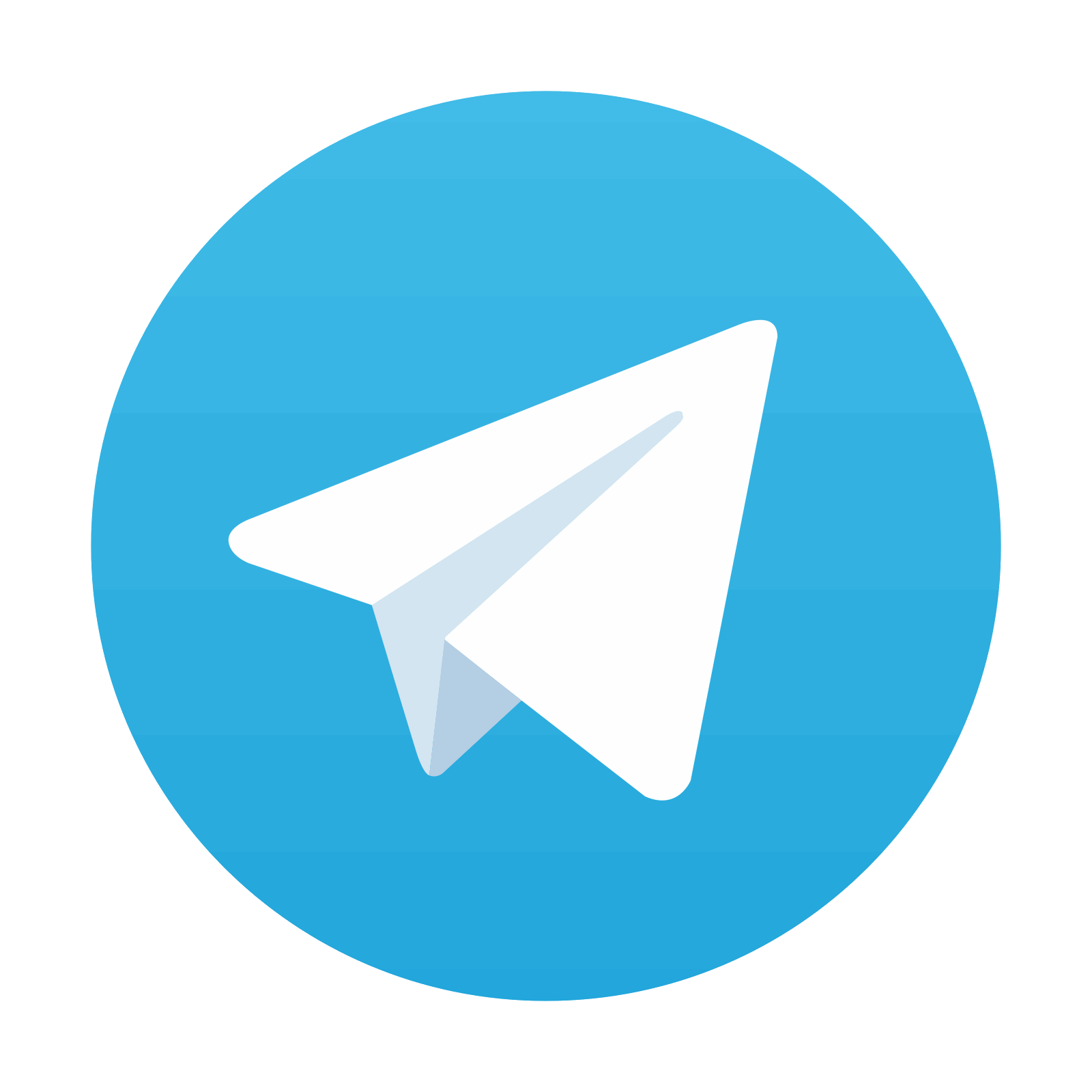
Stay updated, free articles. Join our Telegram channel

Full access? Get Clinical Tree
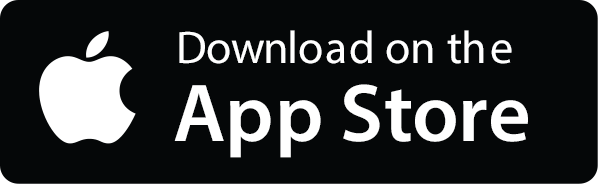
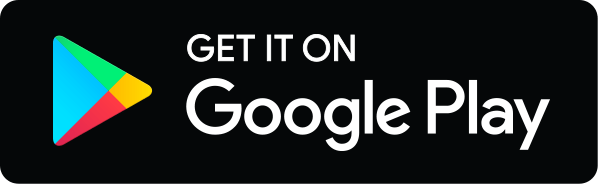