Score
Cerebral performance category (CPC) [2]
Glasgow outcome scale (GOS) [3]
Modified Rankin score (mRS) [4]
0
No symptoms at all
1
Good cerebral performance: conscious, alert, able to work, and lead a normal life. Might have minor psychological or neurological deficits (mild dysphasia, non-incapacitating hemiparesis, or minor cranial nerve abnormalities)
Death
No significant disability despite symptoms: able to carry out all usual duties and activities
2
Moderate cerebral disability: conscious, sufficient cerebral function for part-time work in sheltered environment or independent activities of daily life (dress, travel by public transportation, food preparation). Such patients may have hemiplegia, seizures, ataxia, dysarthria, dysphasia, or permanent memory or mental changes
Persistent vegetative state
Slight disability: unable to carry out all previous activities but able to look after own affairs without assistance
3
Severe cerebral disability: conscious, patient dependent on others for daily support (in an institution or at home with exceptional family effort), because of impaired brain function. Has at least limited cognition. This category includes a wide range of cerebral abnormalities, from patients who are ambulatory but have severe memory disturbance or dementia precluding independent existence, to those who are paralyzed and can communicate only with their eyes, as in the locked-in syndrome
Severe disability (conscious but disabled): dependent for daily support by reason of mental or physical disability, usually a combination of both
Moderate disability: requiring some help but able to walk without assistance
4
Coma/vegetative state: not conscious, unaware of surroundings, no cognition. No verbal and/or psychological interaction with environment
Moderate disability (disabled but independent): independence to a greater degree than merely activities of daily living
Moderately severe disability: unable to walk without assistance and unable to attend to own bodily needs without assistance
5
Brain death: certified brain dead or dead by traditional criteria
Good recovery: resumption of normal life, despite minor neurological and psychological deficits
Severe disability: bedridden, incontinent, and requiring constant nursing care and attention
6
Dead
Continuous Electroencephalography (cEEG)
Widespread use of continuously recorded EEG (cEEG) has led to evolving insights into the function of the brain after injury and the use of EEG for prognosis. cEEG is noninvasive and has excellent time resolution, which can be used to capture brief periods of reactivity over seconds, nonconvulsive seizures (NCSz) over minutes, or trends in variability over hours or even days. Historically, EEG has been qualitatively “graded” in order to be used for prognostic purposes based on intermittent EEG recordings over time after diffuse brain injuries [6, 7]. With the recognition of the high incidence of nonconvulsive seizures [8] and the standardization of terminology used for critical care EEG [9], the past decade has seen a shift away from using cEEG to provide a definitive prognosis in non-anoxic brain injury and toward a more descriptive approach to evaluating the cEEG and its evolution over time.
Status Epilepticus
Generalized Convulsive Status Epilepticus
cEEG plays a crucial role in the management and prognosis of patients who present with generalized convulsive status epilepticus (GCSE). After GCSE has been treated, cEEG is indicated after 20 min if there has been no improvement in neurological exam and after 60 min if the patient has not returned to baseline [8, 10]. Nearly half of patients undergoing cEEG after GCSE will continue to have nonconvulsive seizures, and 14 % have nonconvulsive status epilepticus (NCSE) [11]. NCSE after convulsive status epilepticus in one study was associated with an OR 1.66 for poor outcome defined as death or dependency within 30 days after cessation of SE, even after controlling for age and etiology [11]. In patients with “subtle status epilepticus,” an electromechanical dissociation resulting in NCSE specifically after prolonged GCSE, 30-day mortality has been found to be substantially higher than in those who initially present with GCSE (65 % vs 27 %). cEEG is required to make the diagnosis of NCSE, and successful treatment with an initial agent reduces mortality by half [12].
Nonconvulsive Status Epilepticus
The duration of NCSE and delays to its diagnosis have been significantly associated with mortality at hospital discharge in critically ill patients, even after controlling for etiology, age, and length of stay [13]. In the adult neurological ICU, 11/12 patients with subarachnoid hemorrhage (SAH) who developed NCSE had poor outcome, defined as mRS >3 at 3 months [14]. Patients with traumatic brain injury (TBI) who experience NCSE have higher average intracranial pressures and periods of metabolic crisis [15]. While functional outcomes in one small cohort were similar between patients with and without NCSE after TBI as measured by the extended GOS and the disability rating scale (DRS), hippocampal atrophy on MRI developed at 6 months in those with NCSE [16].
In patients admitted to the surgical ICU, NCSE and nonconvulsive seizures (NCSz) together was “found” to have an OR 10.4 for poor outcome, defined as a GOS 1–3 at discharge. The presence of NCSE was associated with coma and prior clinical seizure and was independently associated with outcome once age and etiology were controlled; two-thirds in the cohort had sepsis [17]. In the medical ICU, 8 % of patients with coma had NCSE in a retrospective analysis, but no mortality differences were seen between those with and without NCSE [18]. A more recent prospective observational study in the medical ICU demonstrated that 11 % of those with severe sepsis, three-quarters of whom were comatose, developed NCSE. In that cohort, NCSE did not appear to independently impact mortality or functional and cognitive outcome measures at 1 year [19]. In children admitted to a general ICU, the probability for neurological decline by hospital discharge increased by 1.13 for each 1 % increase in seizure burden, the number of minutes of electrographic seizure activity per hour [20]. To date, studies that have examined NCSE in adults have been largely underpowered to adequately detect changes in functional or cognitive outcomes based on sample size.
Nonconvulsive Seizures
NCSz refer to discrete electrographic seizures. A precise definition of what constitutes NCSE vs NCSz is not well established, and some studies merge patients with NCSz and NCSE; others merge NCSz with ictal–interictal patterns, described in the next section. Despite this, there is clear evidence to suggest that isolated NCSz, even those that occur in a small region of the brain recorded only using intracortical recordings, result in increases in heart rate and blood pressure and may result in increased intracranial pressure and metabolic demand with reciprocal changes in brain tissue oxygen and regional cerebral blood flow [21]. After SAH, NCSz have been independently associated with poor functional outcome defined as mRS 4–6 at 3 months, even after controlling for age, bleed severity, delayed cerebral ischemia, and inflammatory response [22]. After intracerebral hemorrhage (ICH), NCSz appeared to worsen neurological function and increase midline shift, although overall functional outcome at hospital discharge was not affected [23, 24]. Interestingly, nonconvulsive seizures appear to occur in patients with an intermediate-severity brain injury; while patients with seizures are at higher risk for poor outcome in general, they tend to do better than those who have markedly severe background attenuation or lack reactivity in patients with SAH or with severe sepsis [19, 22].
The Ictal–Interictal Continuum
Critically ill patients may exhibit a variety of periodic or rhythmic patterns during cEEG that do not fulfill typical definitions of NCSz [25]. The interactions between neuronal injury and metabolic dysfunction manifest as abnormal patterns on cEEG which cannot be easily classified, falling somewhere on an ictal–interictal continuum [26]. Periodic discharges (PDs) are commonly associated with acute brain injury and may be acute, prolonged [27], or even chronic [28]. PDs are clearly associated with electrographic seizures [27, 29–31], but their relationship with outcome is less clear. In the medical ICU, the presence of PDs or NCSz has retrospectively been associated with poor outcome defined as GOS 1–3 at hospital discharge [32], although in a prospective study of medical ICU patients with severe sepsis, PDs or NCSz had no impact on 1-year survival or functional and cognitive outcomes [19]. In the setting of central nervous system infection, 48% had either PDs or NCSz with sixfold odds increase for poor outcome at discharge, defined as severe disability or worse [33]. In the surgical ICU, PDs were not independently associated with hospital discharge outcome, although 97 % of patients with PDs lasting longer than 24 h had poor outcome compared with 58 % of patients with transient PDs [17]. In comatose neurological ICU patients, however, prolonged PDs lasting more than 5 days in a row were found to have no impact on mortality [27] compared to intermittent or no PDs.
Of the PDs, lateralized periodic discharges (LPDs) are the best studied. Case series have demonstrated instances of increased regional glucose metabolism or blood flow in the region of LPDs, mirroring changes seen during seizures. After GCSE, LPDs on cEEG have been associated with poor outcome: more than half of those with LPDs died or were dependent at discharge [34]. After intracranial hemorrhage and SAH, LPDs were found to be independent predictors of poor outcome, defined as GOS 1–2 at discharge or mRS 4–6 at 3 months, respectively [14, 24] (see Fig. 1). Although overall mortality rates in patients with LPDs are between 25 and 41 %, much of the mortality associated with LPDs appears to be related to the underlying etiology, and case–control studies are lacking.


Fig. 1
Stimulus-induced lateralized periodic discharges after poor-grade subarachnoid hemorrhage. A 61-year-old comatose woman post-bleed day 8 after modified Fisher 2, Hunt–Hess 4 subarachnoid hemorrhage requiring craniotomy and clipping of a right posterior communicating artery aneurysm. With stimulation, the focal delta frequency slowing seen over the right hemisphere (pictured in a) becomes periodic, with frontally predominant blunted sharp waves at 1Hz (SI-LPDs; pictured in b). Compressed spectral array (at the bottom of each EEG left hemisphere is above the right hemisphere) demonstrates increased delta power over the right (bottom half) more than the left (top half) after stimulation multiple times throughout the 24-hr period. (c) Extent of the subarachnoid hemorrhage. The patient remained in a persistent state of unresponsive wakefulness and was eventually sent to hospice care 6 weeks after discharge from the hospital
Generalized periodic discharges (GPDs), which include triphasic waves, are associated with mortality rates between 30 and 47 %, but when age, etiology, and level of arousal were controlled, there was no association with poor outcome, was found defined as death or vegetative state at hospital discharge [29]. Bilateral independent periodic discharges (BIPDs) have been highly associated with poor outcome, with a mortality of 61 % compared to 29 % in patients with LPDs. Nearly three-quarters of patients with BIPDs were comatose (vs 24 % of patients with LPDs), and with such a small sample size, it is likely that injury severity accounted for much of the mortality [31]. Further studies of BIPDs are needed to fully assess their prognostic importance.
Burst Suppression
Burst suppression (BS) has long been associated with diffuse brain injuries and is caused by a loss of normal cortical inhibition with hyperexcitable bursting interrupted by refractory periods [35]. Prognosis is strongly dependent on etiology: traditionally, BS has been associated with poor outcome after cardiac arrest but in other cases may be seen transiently during deep anesthesia. Asymmetric bursts in particular may point to structural lesions in the corpus callosum [36, 37]. Background suppression, on the other hand, refers to either a low-voltage record of predominantly theta or delta frequencies without reactivity or an isoelectric recording [38]. The prognosis associated with this pattern is typically poor once the effects of sedative medications or metabolic dysfunction (e.g., severe sepsis, uremia, hypercalcemia, thyroid or adrenal failure, hypoglycemia) are ruled out [39]. However, much of the literature on burst suppression and background suppression is focused on anoxic brain injury, and the majority are from the pre-hypothermia era.
Burst suppression may be induced therapeutically, as in refractory intracranial pressure or refractory status epilepticus. The prognosis in these cases is usually considered poor as a result of the underlying etiology, although maintenance of BS induced for treatment of refractory status epilepticus for >72 h may help improve overall seizure control and perhaps even survival [40, 41]. In one series of patients requiring pentobarbital for refractory status epilepticus, 90 % of whom had BS on cEEG, one in ten had minimal or no disability at 1 year [41], and in a series of patients with TBI requiring pentobarbital titrated to BS for refractory intracranial pressure, 24 % survived to good recovery defined as GOS 4–5 at 1 year [42].
Spontaneous BS may be observed in critically ill patients, many of whom require sedation and analgesia as part of their critical care. In neurological ICU patients with ICH, the presence of BS does not significantly portend poor outcome, defined as GOS 1–2 at hospital discharge, once clinical and radiologic predictors are controlled [24]. This appears to be true across neurological ICU patients, regardless of etiology [43]. In the operating room and in some ICUs, BIS monitoring (Covidien; Boulder, CO) is performed via a device designed to process the crossed bispectrum of raw frontal EEG into a simple-to-read number between 0 and 100. Using the BIS, anesthesiologists have reported on a large cohort of patients from two prior clinical trials, 28 % of whom experienced > 5 min of anesthesia-related BS during elective surgery under general anesthesia. Patients who experienced BS were older and had higher preoperative comorbidity. After controlling for these factors, those with both anesthesia-related BS and intraoperative hypotension had three times the risk for 90-day postoperative mortality [44]. Another study found that each hour spent in BS intraoperatively increased mortality at 1 year by 24 %, and each minute spent with a systolic blood pressure < 80 mmHg increased mortality at 1 year by 4 % [45]. Among medical ICU patients, 62 % of whom had sepsis, those with BS had double the hazard ratio for 6-month mortality than those without BS after controlling for age, comorbidities, and illness severity [46].
EEG Background: Variability and Reactivity
The background frequency content of the cEEG is typically reported descriptively: attenuated delta frequency slowing, predominant theta/delta frequencies, organized posterior dominant alpha, etc. Some frequency changes are important for the interpretation of cEEG in the critically ill, such as declining faster frequencies in the setting of inadequate cerebral perfusion, and particularly focal findings such as focal delta frequencies or asymmetry in the EEG related to stroke. Quantitative analysis of the frequency spectra provides useful objective information [47] and may have a role in predicting certain outcomes, as described in Chapter 15. However, the overall background frequency mix is largely nonspecific. Prior attempts to develop grading systems to reflect prognosis after diffuse brain injury have used overall background frequency patterns and their reactivity [6, 7]. However, with the use of continuously recorded EEG, the importance of dynamic parameters such as reactivity and state changes has been more heavily emphasized. As an example, the prognosis of frequency-dominant patterns such as alpha or theta coma, best studied after hypoxia, is predicated on their reactivity [48] and frequently evolves over several days to different patterns altogether [49].
Reactivity refers to a reproducible change in the EEG background as a result of stimulation (see Fig. 2). Although reactivity has received attention as a prognostic marker after cardiac arrest, the reproducibility of this finding is confounded by a lack of standardization of stimulus type and stimulus strength, inter-rater agreement on EEG changes, the effects of confounders such as sedation, and the timing of stimulation during the sleep–wake cycle. The kappa between expert raters for background reactivity in one study of routine EEG segments from patients with a clear “awake” state ranged broadly 0.33–0.95 [50]. Of practical importance in the ICU, the method of stimulation for a given patient needs to take into account other injuries, for example, hearing impairment from canal obstruction following trauma or surgery or sensory loss after cervical spinal cord injury. Caution should be used when applying background EEG reactivity to prognostic decision-making in the clinical setting. Within 48–72 h of TBI, one study used auditory clicks and nasal septum stimulation to elicit one of several categories of EEG reactivity: slow waves, attenuation, no reactivity, and uncertain. Increasing slow-wave activity (so-called paradoxical reactivity) was associated with GOS 4–5 (good recovery) at 18 months post-injury in 90 %. In those without EEG reactivity, 93 % had severe disability or worse; notably, 78 % with uncertain reactivity had good recovery or moderate disability [51]. After SAH, all patients with a poor outcome, defined as mRS >3 at 3 months, lacked EEG reactivity to any alerting stimuli; however, more than half of patients’ reactivity was not reported [14]. After ICH, reactivity was not found to be an independent predictor of outcome in multivariate analysis [24]. In patients with severe sepsis, a standardized stimulation protocol was used to define reactivity, which was absent in patients with continuous sedation, circulatory shock, and coma. Patients without reactivity had a mean survival time of 3 months compared with 8 months for those with EEG reactivity, although 13 % of survivors at 1 year had lacked reactivity in the ICU, and no differences were seen in functional outcome between those with and without EEG reactivity [19].


Fig. 2
Reactivity in a comatose patient with toxic metabolic encephalopathy. A 55-year-old woman in coma 4 days after presenting with ethylene glycol toxicity. cEEG demonstrated severe diffuse background slowing. Stimulation, as marked, produced an attenuation of delta amplitude and an increase in theta frequencies. Termed desynchronization, this is a form of reactivity. The patient regained consciousness several days later and was discharged with mild disability (Glasgow Outcome Scale score 5)
In a special case of reactivity, stimulation may produce ictal–interictal discharges. These stimulus-induced rhythmic, periodic, or ictal discharges (SIRPIDs) have been described in 22 % of patients undergoing cEEG on an inpatient neurology service [52]. SIRPIDs are thought to be related to dysregulated afferent input into hyperexcitable cortex and appear to be associated with the development of seizures. They are seen significantly more frequently after ICH, convulsive status epilepticus, and TBI [52, 53]. After ICH, focal SIRPIDs are independently associated with poor outcome, defined as GOS 1–2 at hospital discharge [24]; after SAH, SIRPIDs do not appear to be predictive of outcome [14]. The impact of SIRPIDs on outcome in general has not been adequately studied.
Variability refers to spontaneous changes in EEG over time, as opposed to reactive changes to a stimulus. A lack of variability – a “monotonous” EEG – has been described after brain injury using raw EEG [54] and using compressed digital spectral array [55] in association with poor outcome defined as death or unresponsive wakefulness. The presence of variability may refer to a simple biphasic EEG that alternates from one abnormal pattern to another over time, to more complex and definitive state changes with a behavioral correlate, or to variability in specific background frequencies during EEG. In a foundational study of states in comatose critically ill patients, all patients with a biphasic or invariant nighttime recording died [54]. In patients with TBI, variability has been quantified using the percentage of 6–14 Hz frequencies in the background EEG. The degree of variability in the first 72 h after TBI significantly correlated with discharge and 6-month GOS, and when added to a prognostic model of outcome that included standard clinical covariates, the alpha variability significantly improved the predictive ability of the model [56, 57]. After SAH, the presence or absence of state changes or spontaneous variability does not appear to independently predict outcome [14]. However, the alpha variability declines in patients with SAH a mean of 2.9 days prior to the onset of vasospasm [58].
Cyclic alternating patterns (CAP) are a specific form of variability described after brain injury. CAP refers to periodic bursts of delta frequencies in the absence of stimulation (hence, not reactivity). These patterns mimic normal sleep microarchitecture and appear independent of other alternating physiologic patterns, such as Cheyne–Stokes breathing. In one series of patients with CAP, 7 of 11 patients experienced good outcome (complete recovery or minimal dependency) after a follow-up period of several months [59] (see Fig. 3). In another cohort 1–2 weeks after traumatic injury, CAP was described in six patients, none of whom had a good outcome. This may have been related to the persistence or delayed presentation of CAP or a lack of overlying sleep transients which were not reported in the former series [60].


Fig. 3
Cyclic alternating patterns and sleep transients in a comatose patient after traumatic brain injury. A 24-year-old man post-trauma day 2 from a high-speed motor vehicle collision. Injuries included subarachnoid hemorrhage and Barrow A (direct) left carotid–cavernous fistula. Initial Glasgow Coma Scale score was 5 on scene with unilateral pupillary nonreactivity on arrival to the neurological intensive care unit. Multiple intracranial pressure crises required sedation and limited examination. (a) Admission CT with traumatic subarachnoid hemorrhage. (b) Clear K-complex; diffuse background beta frequencies in the setting of propofol sedation makes distinguishing clear sleep spindles impossible. (c) A cyclic alternating pattern with paradoxical bursts of high-amplitude delta slowing (denoted by brackets), not apparently related to specific stimulation. At follow-up 5 months post-injury, Glasgow Outcome Scale score was 5
Sleep
Sleep is frequently abnormal in critically ill patients with or without brain injury [61, 62]. In one series, only 30 % of critically ill patients had normal sleep at any point during cEEG [61]. The majority of patients in the ICU have state changes that do not fit the standard definitions of sleep as defined by the American Academy of Sleep Medicine. The term “atypical sleep” has been used to describe periods of behavioral or electrographic sleep-like states in these patients, who lack typical sleep transients such as K-complexes or sleep spindles [63]. After TBI, patients with typical sleep features have favorable prognosis: the presence of K-complexes or sleep spindles had a positive predictive value of 86 % for no or only mild disability at more than 1-year follow-up [60], and increasing spindles have been seen prior to recovery of consciousness and have been correlated to the duration of coma [54].
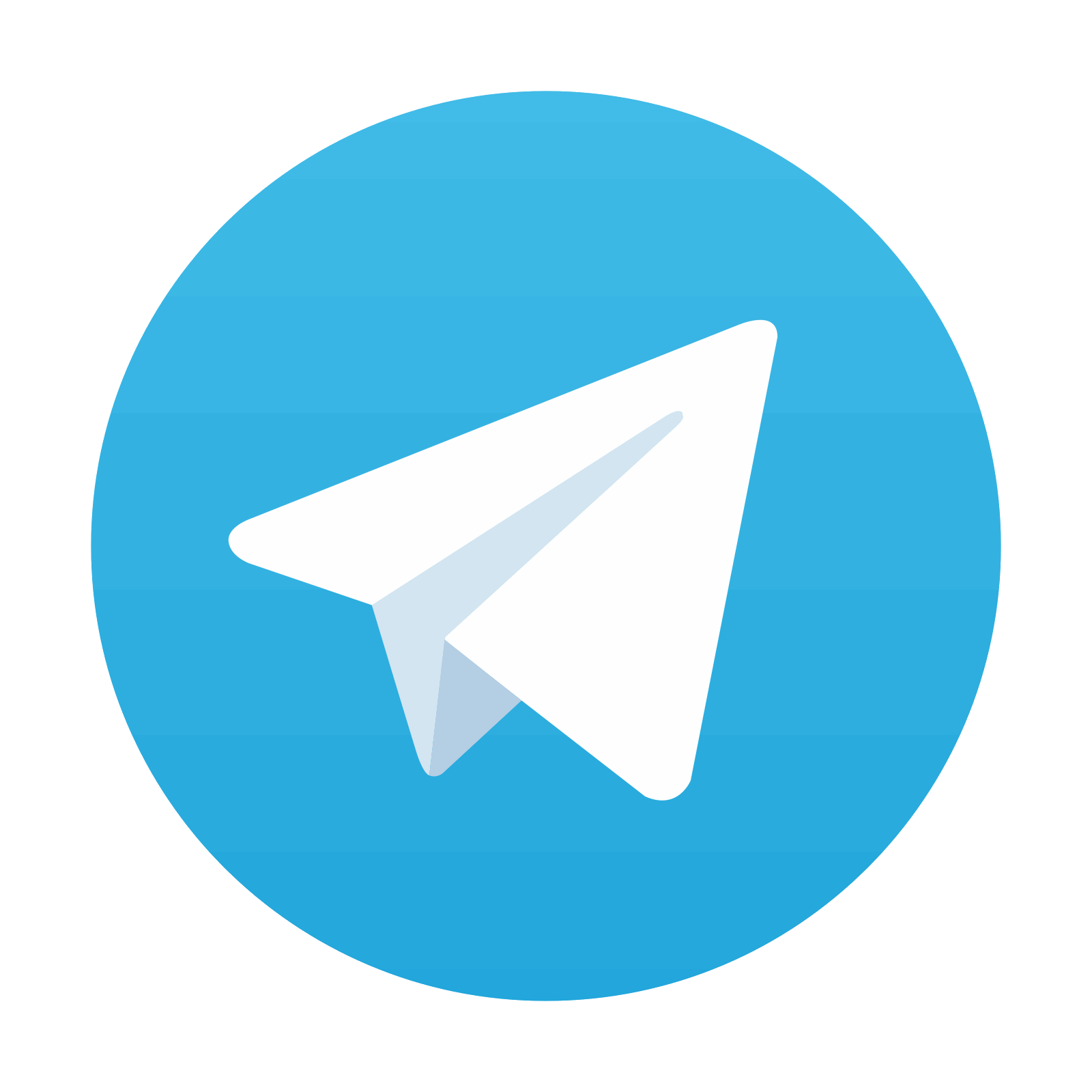
Stay updated, free articles. Join our Telegram channel

Full access? Get Clinical Tree
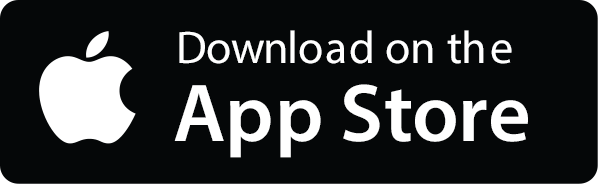
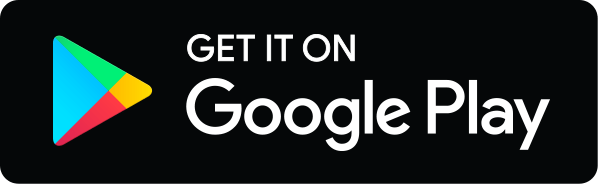