Progressive Myoclonus Epilepsies: EPM1, EPM2A, EPM2B
Elayne M. Chan*
Danielle M. Andrade*
Silvana Franceschetti†
Berge Minassian‡
*Program in Genetics and Genomic Biology, The Hospital for Sick Children, Toronto, Canada
†Department of Clinical Neurophysiology, Instituto Nazionale Neurologico Carlo Besta, Milan, Italy
‡Division of Neurology, The Hospital for Sick Children, Toronto, Canada
Introduction
The differential diagnosis of progressive myoclonus epilepsy (PME) with onset between late childhood and late adolescence includes neuronal ceroid lipofuscinosis, type I sialidosis, and myoclonic epilepsy with ragged red fibers. However, the two most common forms of PME in this age group are Lafora disease and Unverricht–Lundborg disease (ULD). This chapter reviews the clinical and pathologic features, molecular genetics, and animal models of these two disorders. As will be seen, in both, the promise of great insights following disease gene discovery has indeed materialized, but with it has also the realization that much work lays ahead. It is hoped that a clear understanding of pathophysiology will uncover therapeutic entry points to allow better treatments, or cures, for these disorders.
Unverricht–Lundborg Disease
Clinical Characteristics and Neurophysiology
Unverricht–Lundborg disease (ULD) is an autosomal recessive PME. Onset is between ages 6 and 15 years, after a period of apparent normal development. The disease is principally characterized by stimulus-sensitive myoclonus (1,2,3,4). These are sudden, brief, shocklike muscle contractions that become generalized and interfere with routine activities such as writing, swallowing, speaking, and walking. They can be precipitated by the simple intention to move. Tonic-clonic and myoclonic seizures also appear early in the clinical picture, but other types of seizures may present later. The disease progresses slowly, with ataxia, action tremor, and emotional lability, and patients demonstrate a slow decline in intelligence.
In recent years, it has become evident that the clinical course is greatly influenced by antiepileptic medications. Phenytoin is exquisitely neurotoxic to these patients and appears to have been a major contributor to the severe progressive myoclonus and cerebellar ataxia documented in previous years. In correctly managed patients, dementia can be averted, myoclonus and seizures can be minimized, and life span can be normal (5). Valproic acid, zonisamide, and levetiracetam are preferred anticonvulsants, and piracetam is helpful against the myoclonus. N-Acetylcysteine (NAC) improves tremor, gait, and myoclonus in some patients (6). Its mechanism of action is poorly understood and has been related to protection against oxidative stress. However, NAC is also antiapoptotic in cultured neurons deprived of nerve growth factor (7) and may be protective against the apoptotis recently shown to characterize ULD neurodegeneration (see the following section).
Electroencephalographic (EEG) recordings are abnormal in ULD, even before symptom onset (4). Background activity is disorganized and slow, EEG paroxysms of fast spike-waves or polyspike-waves with larger amplitudes in central regions are present, and photosensitivity is prominent. The frequency of EEG paroxysms diminishes with rational antiepileptic treatment (8). The stimulus sensitive myoclonus lacks a visible EEG correlate, but backaveraging analysis demonstrates time-locked cortical spikes preceding the myoclonic jerks (9). Giant somatosensory evoked potentials (SEPs) and enhanced long-loop (cortical) reflex (LLR) (9) further implicate cortical hyperexcitability in the myoclonus of ULD. Additional observations derived from comparisons with the neurophysiology of Lafora disease are discussed next.
EPM1, the ULD Gene
The gene responsible for ULD was identified using a positional cloning approach. A genome-wide search for linkage was undertaken in 12 families localizing the gene to 21q22.3 (10). Linkage disequilibrium analysis followed by a systematic search for genes resulted in the identification of the gene for a previously known but unmapped cysteine protease inhibitor called cystatin B (CSTB). Ultimately, the identification of two point mutations in this gene proved it to be responsible for ULD (11). EPM1, the CSTB gene, is composed of three exons and is 674bp in length. To date, a total of six different mutations have been identified in the coding region of EPM1. Three mutations, 1926-1G→C, 2027G→A, and 2355-2A→G affect conserved splice-site sequences and predict severe splicing defects. Two other mutations in exon 3, consisting of a nonsense codon (R68X) or a frameshift (2400 delTC), predict a truncated protein. The sixth mutation is a transversion in exon 1 that results in the substitution of a highly conserved glycine by an arginine at amino acid position 4 (G4R)(11,12,13).
Interestingly, only 10% of EPM1 alleles contain mutations within the transcriptional unit. Southern blot analysis of PstI-digested genomic DNA revealed the presence of abnormally large EPM1-containing fragments in ULD patients. PCR amplification and sequence analysis demonstrated the expansion to be composed of a CCCCGCCCCGCG dodecamer repeat 175bp upstream from the translation initiation codon of EPM1. Normal alleles contain two to three tandem copies of this dodecamer (although rare normal alleles containing 12–17 repeats have also been reported). ULD patients have 30 to 150 copies, resulting in dramatically reduced, although not completely absent, expression of EPM1 mRNA (13,14,15,16,17).
Preliminary analysis revealed no correlation between the number of repeats and clinical severity of the disease or age of onset (13,14,15,16,17). It is suggested that once the dodecamer repeat expands beyond a critical threshold, EPM1 gene expression is reduced in certain cells with pathological consequences. In vitro studies have demonstrated that altered spacing of transcription factor-binding sites upstream of the dodecamer repeat contribute to the reduction in downstream gene expression (18). However, the two- to fourfold reduction in transcriptional activity observed in this study was not as dramatic as that observed in ULD patients. Further studies are required to determine additional causes for the low expression of EPM1 associated with the dodecamer expansion.
Cystatin B and the Cystatin B-Deficient Mouse
The cystatin superfamily encompasses proteins that contain multiple cystatin-like sequences. Some of the members are active cysteine protease inhibitors, whereas others do not have this inhibitory activity. There are three inhibitory families in the superfamily, including the type 1 cystatins (stefins), type 2 cystatins, and kininogens. CSTB is a stefin that functions as an intracellular protease inhibitor. CSTB is able to inhibit papain and cathepsins B, H, L, and S in vitro (19). This protein is thought to play a role in protecting cellular structures against lysosomal proteases in vivo.
Previous histopathological studies of patients with ULD demonstrated cerebellar granular and Purkinje cell loss, gliosis, and neuronal degeneration of the medial and reticular nuclei of the thalamus. Such studies also reported degenerative changes in the cortex, striatum, mammillary bodies, anterior and lateral thalamic nuclei, multiple brain stem nuclei, and ventral gray matter of the spinal cord (3,20,21). However, until recently it was not clear if these pathological findings were the consequence of a genetic mutation, leading to reduced levels of CSTB, or the result of neurotoxicity from phenytoin, the antiepileptic drug used for many years to treat ULD. This issue was resolved in 1998, when Pennacchio and colleagues reported histopathological findings in EPM1 knockout mice that did not receive phenytoin treatment (22). Targeted disruption of EPM1 caused these animals to develop a phenotype that resembles ULD, including myoclonic seizures and ataxia. Histopathological analysis revealed apoptosis of cerebellar granular and Purkinje cells. A more detailed study with nontreated EPM1 knockout mice demonstrated not only apoptosis of cells in the cerebellum, but also cortical and striatal gliosis and atrophy of cortical neurons (23).
A recent study analyzed transcript levels of brain-expressed genes in CSTB-deficient mice by using modified differential display oligonucleotide microarray hybridization and quantitative reverse transcriptase polymerase chain reaction. Interestingly, levels of the protease cathepsin S were consistently elevated in vivo, whereas alterations in the levels of cathepsins B, H, and L were inconsistent (24). Protease activity could be entirely regulated at the level of uninhibited protein availability, but another possibility is that there are feedback loops that alter the levels of protease transcripts in response to the lack of the protease inhibitor CSTB. In this case, the finding of consistently elevated levels of cathepsin S suggests that proteolysis by this protein may be a key trigger event in the initiation of apoptosis in ULD.
Another interesting finding in the study of Lieuallen and colleagues is the elevated mRNA levels of five other genes in the brain of EPM1-deficient mice when compared to controls. These genes, encoding for β2-microglobulin, apolipoprotein D, fibronectin 1, Gfap, and C1qB, may have transcript levels elevated as a result of glial activation (24). These gene products have been reported to be increased in reactive astrocytes (β2-microglobulin and apolipoprotein D), secreted by glial cells and involved in astroglial proliferation (fibronectin 1), and expressed in microglia (C1qB and Gfap). Reactive fibrillary astrocytes, capable of producing microglial scars (gliosis) create a physical barrier between damaged and healthy neurons, possibly as a form of adaptation to protect the remaining healthy cells. Glial activation has also been reported in other neurodegenerative diseases including Alzheimer’s disease and Creuzfeldt–Jakob’s disease (25,26), where it is also thought to be a biological response to neuronal damage.
One more indication that CSTB has a neuroprotective role comes from the study of D’Amato and colleagues (27), who demonstrate that a series of focal and generalized seizures induced by kindling brain stimulation in wild-type mice lead to a transient elevation of EPM1 mRNA accompanied by transient elevation of CSTB levels in some areas of the brain. It is believed that this is an endogenous neuroprotective mechanism that acts to limit neuronal apoptosis caused by the electric insult.
Currently the greatest challenge in ULD research is the question of whether the known CSTB effect on cathepsins is the pathophysiologically relevant function, or whether alternate CSTB functions exist, yet to be revealed. Paradoxically, in contrast to Lafora disease (LD), discussed next, the absence of genetic heterogeneity in ULD does not allow the genetic unraveling of a second pathway, whose intersection with the CSTB pathway(s) would help establish the biological underpinnings of the disease.
Lafora Disease
Clinical Characteristics
LD is an autosomal recessive PME. Onset is between ages 9 and 18 years, after a period of apparent normal development (28). In retrospect, however, many families describe a certain “slowness” in childhood years compared to their unaffected children. In fact, a subphenotype with learning disabilities in childhood associated with particular mutations has been delineated (29). In addition, febrile and rare afebrile seizures in childhood are more common in LD than in the general population (28).
The LD patient is brought to clinical attention following the appearance of one of five symptoms: myoclonic jerks, a generalized seizure, a decline in school performance, behavioral changes including depression, apathy, disinhibition or delusions, or visual (occipital ictal) hallucinations. At this point, EEG shows slowing of the background with irregular spike-wave discharges, raising the specter of a progressive disease. The disease then accelerates, with worsening of all the above manifestations and additional seizure types. Proper antiepileptic medications have a major effect against generalized convulsions, sometimes resulting in freedom from seizure for many months at a time. In fact, generalized convulsions are rare in treated patients even years after disease onset. Myoclonus also diminishes initially with treatment, but this response is not maintained.
The clinical picture within 2 years of diagnosis is as follows. Myoclonus is frequent during wakeful hours, especially in the early morning, and ceases with sleep. It affects walking, and with cerebellar ataxia and drop attacks, and soon results in wheelchair dependence. It is stimulus sensitive, provoked by movement, intention, light changes, and is exacerbated by emotion. Myoclonus, ataxia, and dysarthria combine with cognitive decline, emotional lability, visual hallucinations, delusions, and disinhibited behavior (with pursuant social rejection) to devastate the young person. Within 5 to 10 years, the patient is bed bound, usually tube fed, and in almost constant myoclonus and ictal confusion. Through the long years in this state, periods of semilucidity and responsiveness to family are maintained, which renders letting go extremely difficult for some parents. Death usually results from status epilepticus and aspiration pneumonia.
The traditional antiepileptic treatment for LD is valproic acid. In recent years, zonisamide has been shown to have a significant effect on seizures and myoclonus (30,31), and is presently the authors’ first-line choice. Piracetam and levetiracetam (32) are temporarily helpful against the myoclonus. The ketogenic diet is an important therapeutic modality. The Lafora bodies, which occupy vast numbers of dendrites in LD brain, are carbohydrate accumulations and might diminish in the absence of carbohydrate intake. We have been placing our newly diagnosed cases on the diet. Our observations so far suggest an important slowing of disease progression. Controlled experiments of the diet in animal models are being initiated.
Neurophysiology
The EEG background activity in LD is slow, showing dominant theta-delta components interrupted by recurrent posterior or diffuse multiple spikes discharges (33). Generalized or segmental spontaneous myoclonus, associated with clear EEG paroxysms, is a prominent feature, and the presence of negative myoclonus, associated with polyspike-wave discharges, is common and characteristic (34).
The large amplitude of cortical SEPs components found in LD (and ULD) indicates that in both disorders an aberrant integration of somatosensory stimuli occurs and can subsequently involve motor cortex. In agreement, protocols investigating motor-evoked potentials demonstrated that somatosensory afferent stimuli delivered prior to transcranial magnetic stimulation are capable of abnormally facilitating motor cortex excitability in PME case series (35,36). However, SEPs can be normal in some patients (35,37), or “normalized” by antiepileptic treatment, but action myoclonus usually persists, suggesting that motor cortex “per se” is primarily hyperexcitable. In agreement, enhanced LLR, and central spikes time locked with action myoclonus may occur also in patients with “normal” SEPs.
To better investigate the hyperexcitability of motor cortex, spectral analyses have been applied as a method for detecting the relationship between rhythmic or quasirhythmic myoclonic events and EEG (or magneto-EEG) activity (37,38). The data indicate that highly coherent beta–gamma oscillations occur between motor cortex and muscle during movement-activated myoclonic discharges, and that pathologically exaggerated fast rhythms probably drive the generation of cortical myoclonus. In a recent study (39), performed by applying autoregressive models to the analysis of short EEG–electromyogram (EMG) epochs, a coherent beta activity coupling motor neocortex and contralateral muscles involved in action myoclonus was found in both LD and ULD. However, the coherence profile was more heterogeneous in LD patients, including multiple coherence peaks in subjects with more severe disease progression. This could suggest that a complex distortion of corticosubcortical or corticocortical interaction occurs in LD, probably accounting for the greater heterogeneity of the myoclonus, including spontaneous generalized and focal (positive or negative) negative myoclonus.
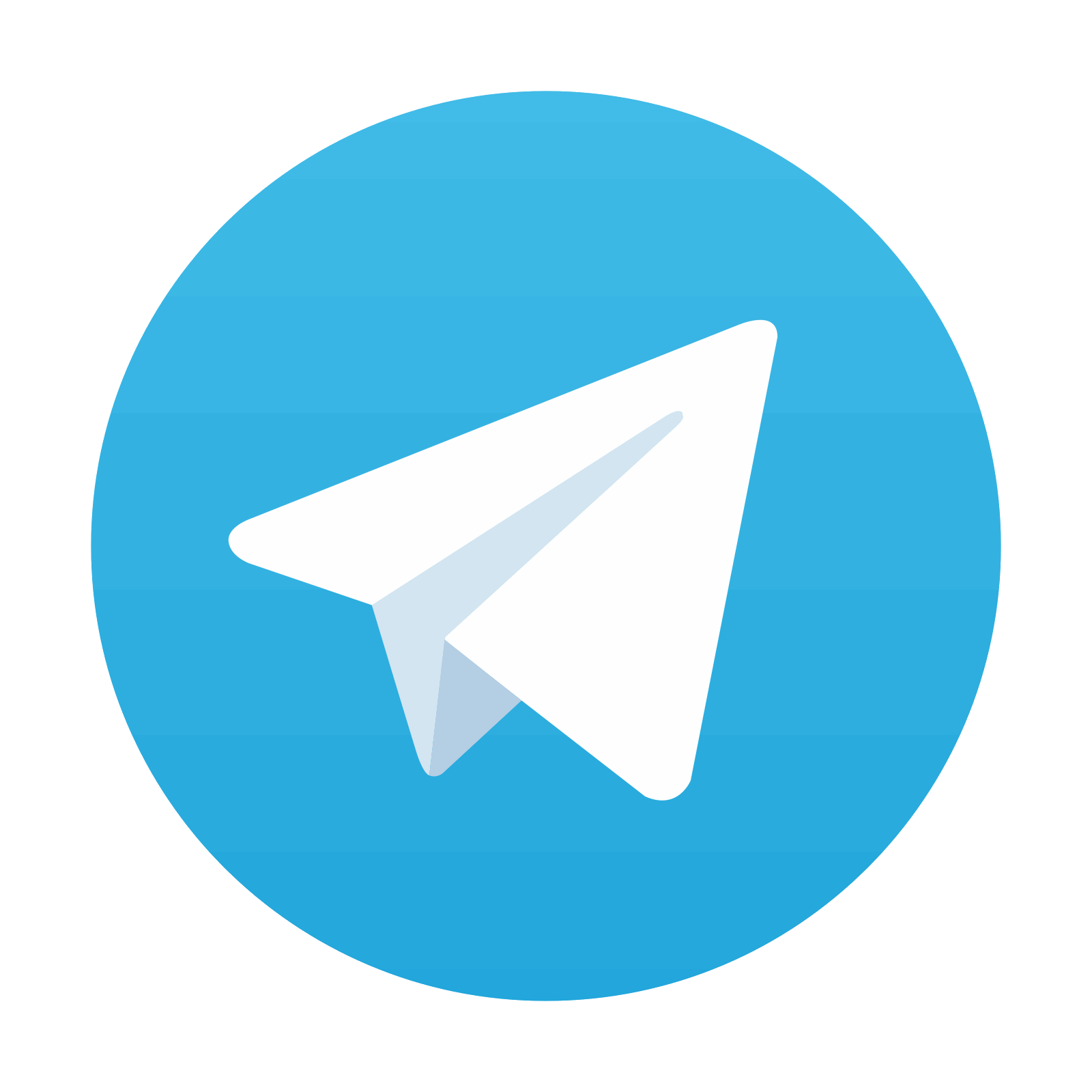
Stay updated, free articles. Join our Telegram channel

Full access? Get Clinical Tree
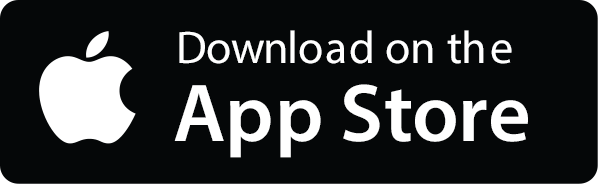
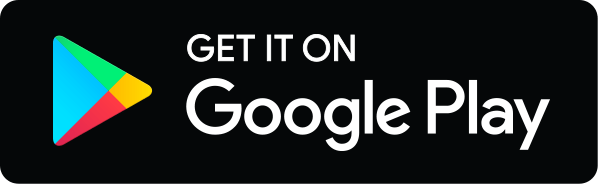