No patients
Histology
Extent of resection
Median overall survival by group (months)
Supportive
care (mo)
Chemoa (mo)
Radiationb (mo)
ChemoRTa (mo)
BTSG 69–01 [13]
(1978)
303
90% GBM, 9% AA, 1% other HGG
5% biopsy only; otherwise varied
3.2
4.2
8.1
8.0
BTSG 72–01 [14]
(1980)
467
84% GBM, 11% AA, 5% other HGG
“Definitive surgical intervention”
–
5.5
8.3
9.7 (semust.)
11.7 (BCNU)
SGSG [12]
(1981)
118
Grades III and IV astrocytoma
“Large tumor resection”
5.2
–
10.8
10.8
University Hospital, Lund, Sweden [16] (1991)
171
53% Grade III, 42% Grade III–IV, 4% Grade IV
35% gross total resection
–
10.5
–
15.5
In addition to RT, adjuvant temozolomide (TMZ) has become standard of care based on a landmark study by Stupp et al. [20] demonstrating a median survival benefit of 2.5 months with the addition of TMZ concomitant with and adjuvant to RT after debulking surgery for glioblastoma. Median overall survival at two years was also improved from 10 to 26%. The benefit of TMZ is most pronounced in patients with MGMT promoter methylation; in this patient population, median survival improved from 15.3 months (95% CI, 13.0–20.9) to 21.7 months (95% CI, 17.4–30.4) (p = 0.007) [21]. The MGMT gene encodes a DNA-repair protein that removes alkyl groups from the O6 position of guanine, an important site of DNA alkylation. Chemotherapy-induced lesions, especially O6-methylguanine, trigger cytotoxicity and apoptosis if left unrepaired. High levels of MGMT activity in cancer cells can decrease the therapeutic efficacy of alkylating agents [21]. However, promoter methylation leads to silencing of this gene, which prevents DNA damage repair. TMZ improves overall survival even for those patients with unmethylated MGMT albeit to a much smaller degree than in patients harboring MGMT promoter methylation [22]. MGMT promoter methylation has also been shown to be an independent favorable prognostic factor. Induction TMZ was proposed for patients with newly diagnosed glioblastoma given these data demonstrating the significant benefit of TMZ. A phase II trial by Chinot et al. [23] gave patients with inoperable newly diagnosed glioblastoma TMZ in a 7-day on/7-day off regimen for 28 days for up to 4 cycles prior to delivery of conventional RT. At a median follow-up of 6 months, median progression-free and overall survival was shorter than other published reports [20]. Based on these data, the standard of care for GBM remains maximal safe surgical resection followed by adjuvant concurrent chemoradiation then adjuvant chemotherapy.
Molecular analyses may play an increasingly important role in treatment recommendations for patients with glioblastoma. This is particularly relevant given recent studies that demonstrate that gliomas can be classified based on TERT promoter mutations, IDH mutations, and 1p/19q codeletion. This classification method identifies groups with distinct ages at diagnosis, overall survival, and association with germ line variants [24]. This methodology is particularly relevant given poor histopathologic reproducibility among pathologists when classifying gliomas, particularly for tumors with oligodendroglial components [25].
Management of Malignant Glioma in the Elderly
Management of high-grade glioma, specifically glioblastoma, in the elderly and in patients with poor performance status is a specific research focus. This research is motivated by a concern that long courses of RT or combined modality therapy may not be tolerable in this patient population. Keime-Guibert et al. [26] examined patients randomized to either fractionated RT (50 Gy in 28 fractions) and supportive care or supportive care only, which consisted of treatment with corticosteroids and anticonvulsant agents, physical and psychological support, and management by a palliative care team. Patients were 70 years of age or older with a newly diagnosed anaplastic astrocytoma or glioblastoma and a KPS 70 or greater. Eighty-five patients were enrolled, and the trial was discontinued at the first interim analysis based on results demonstrating superiority of RT and supportive care when compared to supportive care alone. At a median follow-up of 21 weeks, the median survival was 29.1 weeks (95% confidence interval (CI), 25.4–34.9 weeks) with RT and supportive care compared to 16.9 weeks (95% CI, 13.4–21.4 weeks) with supportive care alone (p = 0.002). Importantly, the investigators also examined differences in KPS, health-related quality of life, and cognition and did not find significant differences between the two treatment arms. These results demonstrate the benefit of RT for anaplastic astrocytomas or GBM in an elderly patient population with good performance status.
Two studies examined shorter-course RT in elderly patients or those with worse performance status. One Canadian study by Roa et al. [27] randomized patients to standard (60 Gy in 30 fractions) versus hypofractionated (40 Gy in 15 fractions) RT in 100 patients equal to or older than 60 years old with histologically confirmed GBM and a KPS of 50 or greater. The investigators did not see a statistically significant difference in overall survival between the two regimens with a similar median survival of 5.1 months in the standard RT arm and 5.6 months in the hypofractionated arm (p = 0.57). Another prospective study by the Nordic Clinical Brain Tumour Study Group randomized patients older than age 60 to one of three regimens: six monthly cycles of TMZ, standard fractionated RT (60 Gy in 30 fractions), or hypofractioned RT (34 Gy in 10 fractions).[28] For patients ages 60–70, there was no difference in overall survival. However, for patients older than 70, treatment with either TMZ or hypofractionated RT resulted in improved overall survival when compared with standard fractionation RT. Temozolomide alone was shown to be non-inferior compared to standard fractionation RT in patients older than 65 with glioblastoma or anaplastic astrocytoma in NOA-08, a phase 3 randomized trial [29]. MGMT promoter methylation was independently associated with improved overall survival. Event-free survival was dependent on MGMT promoter methylation status; those patients with promoter methylation had improved overall survival if they were randomized to TMZ compared to RT; the inverse was also true for those without promoter methylation. These results together suggest that for elderly patients or patients with a poor performance status with newly diagnosed glioblastoma or anaplastic astrocytoma, hypofractionated radiation therapy or temozolomide alone may be considered. MGMT status can help guide the choice between TMZ and RT monotherapy.
Anaplastic Astrocytoma
Three histologic subtypes of WHO grade III anaplastic gliomas are described by the WHO 2007 classification system: anaplastic astrocytoma (AA), anaplastic oligodendroglioma (AO), and anaplastic oligoastrocytoma/mixed anaplastic glioma (AOA). MGMT promoter methylation has been identified as a prognostic marker in patients with grade III gliomas; it is unclear whether it is also a predictive factor as it is in patients with glioblastoma [30]. As astrocytic tumors tend to behave more aggressively than oligodendroglial tumors, the recommended approach to AA is maximum safe resection typically followed by adjuvant radiation therapy, with or without concurrent TMZ. This approach is extrapolated from the management of GBM. Although patients with AA were only a small proportion of the total numbers of patients, the trials from the 1970s and 1980s described above that demonstrated a survival benefit with the addition of adjuvant RT following surgery as compared to chemotherapy or supportive care alone support the use of RT in this patient population [13, 14]. Although there are no data to guide the optimal dose and/or RT schedule in treating patients with grade III gliomas, many radiation oncologists choose to use a dose of 59.4 Gy in 1.8 Gy fractions for grade III tumors in comparison to 60 Gy in 2 Gy fractions like for glioblastoma. The rationale is that the biologic dose reduction and smaller fraction size may lead to reduced late normal tissue toxicity for patients with more favorable prognoses.
The role for adjuvant chemotherapy for patients with AA is less definitive than in patients with glioblastomas. Often recommendations are made based on molecular features that suggest the tumor will behave more like a lower or higher grade tumor. Before the introduction of TMZ, procarbazine, lomustine, and vincristine (PCV) chemotherapy was most often prescribed. Recently presented results from NRG Oncology/Radiation Therapy Oncology Group (RTOG) 9813, a randomized phase III study, demonstrate similar overall survival but decreased toxicity, most related to bone marrow toxicity, when utilizing radiation therapy and TMZ as compared to radiation therapy and nitrosourea-based chemotherapy for patients with AA [31].
The recently closed CATNON trial is a randomized Phase III trial of anaplastic glioma without 1p/19q loss of heterozygosity (Clinicaltrials.gov, NCT00626990). The purpose of the trial is to study the impact of temozolomide concomitant with and adjuvant to RT in this patient population. This study will help elucidate the optimal treatment of anaplastic glioma by taking into account both histology and genotype. Until these results are available, knowledge of clinical and molecular prognostic factors and extrapolation from data from studies of higher and lower grade tumors will help guide the optimal therapy in patients with AA.
Anaplastic Oligodendroglioma
Anaplastic oligodendrogliomas and mixed anaplastic oligoastrocytomas generally have a better prognosis than AA, with a median survival of at least 3–15 years compared with 2–3 years, respectively. Based on molecular analysis, these tumors have been characterized by a greater likelihood of harboring specific molecular markers, including co-deletion of 1p/19q chromosomes, which is a favorable prognostic feature associated with improved response to treatment and better survival [32]. These tumors are also more likely to show mutations in IDH.
RTOG 9402, a phase III trial randomizing patients with pure AO or AOA to induction PCV chemotherapy plus RT versus RT alone, showed no difference in overall survival for the entire cohort. However, the median survival of those with co-deleted 1p/19q tumors treated with PCV and RT was twice as long as patients receiving RT alone (14.7 vs. 7.3 years; p = 0.03) [33]. European Organization for the Research and Treatment of Cancer (EORTC) 26951 similarly randomized patients with AO or AOA to RT with or without adjuvant PCV. Overall survival in the RT/PCV treatment arm was significantly longer for all patients (42.0 vs. 30.6 months) and there was a trend toward greater benefit from adjuvant PCV in the patients with a 1p/19q codeletion [34].
At most centers, similarly to anaplastic astrocytoma and glioblastoma, TMZ has replaced PCV chemotherapy secondary to reduced toxicity and ease of administration; there are no randomized trials comparing these two agents. Further complicating the sequencing of therapy is that PCV chemotherapy cannot safely be given concurrently with RT; therefore, it was given before or after RT in the published trials. Therefore, if the decision is made to extrapolate these data and use TMZ as an alternative chemotherapy, the question arises whether TMZ should be given prior to RT, following RT, or concurrent to and following RT. The optimal sequence of RT and chemotherapy remains unknown; the most common approach is RT with concurrent and adjuvant TMZ. For patients with mixed oligodendroglial and astrocytic components, often the presence or absence of the 1p/19q co-deletion and MGMT status are used to guide the decision on whether to include chemotherapy with RT.
Given the concerns about the neurocognitive toxicities of radiation therapy and the chemosensitivity of anaplastic oligodendrogliomas to chemotherapy, some practitioners have chosen to utilize chemotherapy alone in the post-operative adjuvant setting and utilize RT only as salvage therapy [35]. The NOA-04 trial randomized patients with grade III anaplastic gliomas to PCV chemotherapy versus RT versus TMZ following surgical resection; if unacceptable toxicity or disease progression occurred, patients who had previously received RT received chemotherapy whereas those who had only received chemotherapy received RT [30]. Patients randomized to upfront RT or chemotherapy had similar outcomes with regard to median time to treatment failure. Long-term results that were presented recently in abstract form confirm a similar efficacy of these different approaches [36]. The CODEL trial, which is sponsored by the Alliance for Clinical Trials in Oncology (Clinicaltrials.gov, NCT00887146), is currently accruing and randomizing patients to RT with concomitant and adjuvant TMZ, RT with adjuvant PCV chemotherapy, or TMZ alone for patients with 1p/19q co-deleted anaplastic glioma or low-grade glioma. Initially, RT alone was one of the randomized treatment protocols; however, based on the results from RTOG 9402 and EORTC 26951, this arm was discontinued. The results from this trial will help elucidate the optimal treatment approach for patients with 1p/19q co-deleted tumors.
Low-Grade Gliomas
Low-grade gliomas are a heterogeneous group of intracranial and spinal tumors that occur primarily in children and young adults. As defined by the WHO grading system, these tumors are grades I-II/IV. Molecular and genetic analyses are increasingly helpful in understanding the clinical behavior of these tumors. The known negative prognostic factors include age greater than 40, astrocytoma histology, persistent neurologic symptoms, new symptoms after an asymptomatic period, high Ki-67 (>3%), and crossing the midline [37, 38]. This section will only address the role for radiation therapy in adults with grade II gliomas, including astrocytomas, oligodendrogliomas, and mixed oligoastrocytomas given the breadth of management approaches to low-grade gliomas across histologies and patient populations.
The role for immediate post-operative radiation therapy in grade II gliomas is less clear than in patients with higher grade gliomas. EORTC 22845 was a phase 3 trial that randomized 311 patients to early RT (54 Gy in 1.8 Gy daily fractions), defined as a maximum of eight weeks between surgical resection and the start of RT, or RT at the time of clinical and/or radiographic progression [39]. All patients underwent maximal debulking surgery at diagnosis. While progression-free survival was improved with early RT (55% vs. 35%, p <0.0001), median overall survival was similar across the arms (7.2 vs. 7.4 years in the early versus no early RT, p = 0.9). Importantly, early RT did not significantly increase the risk of transformation to high-grade glioma. Seizures were better controlled in the early radiotherapy group but a full quality of life analysis was not performed so the impact of RT on other measures is unknown. This trial is often used as justification to postpone RT until disease progression.
If adjuvant RT is recommended, two trials examined the impact of dose escalation on outcomes. EORTC 22844 randomized 379 patients with supratentorial low-grade glioma to either 45 Gy or 59.4 Gy of RT post-operatively. There was no significant difference in overall survival or disease-free survival between the 2 arms; however, patients in the high-dose arm reported significantly worse levels of functioning and more symptom burden following RT [40, 41]. An Intergroup trial conduced by the National Central Cancer Treatment Group (NCCTG)/RTOG/Eastern Cooperative Oncology Group (ECOG) randomized 203 patients with low-grade glioma to either 50.4 Gy or 64.8 Gy post-operative RT [42]. Similar to EORTC 22844, this study showed no overall survival or disease-free survival benefit and increased toxicity with increased RT dose. Grade 3–5 radionecrosis was more common in the high-dose arm (5% vs. 2.5%). Taken together, the EORTC 22844, EORTC 22845, and the Intergroup trials suggest that the decision to recommend adjuvant RT should be made after review of a patient’s risk factors, clinical status, and discussion with the patient about the risks and benefits. For patients with more favorable risk factors, initial observation after surgery with RT reserved for salvage therapy may be reasonable. For older patients with unresected disease or unfavorable features, immediate adjuvant therapy should be considered. In those patients in whom adjuvant radiation therapy is recommended, most radiation oncologists recommend 50.4–54 Gy in 1.8 Gy daily fractions to balance efficacy and toxicity.
With regard to the addition of chemotherapy to early RT, RTOG 9802 randomized patients with grade 2 gliomas with KPS 50 or greater and high-risk features, defined as age 40 years old or older or 18–39 with a less than gross total resection, to adjuvant RT alone versus RT followed by six cycles of PCV [43]. The first published report demonstrated that there was a trend toward improved progression-free survival patients with the addition of PCV chemotherapy (5-year progression-free survival: 63% vs. 46%, p = 0.06); 5-year overall survival was not significantly different (72% vs. 63%, p = 0.13). However, on post hoc analysis, for 2-year survivors the addition of PCV to RT did confer a survival advantage. More recent results presented in abstract form demonstrate improved median survival (13.3 vs. 7.8 years, p = 0.03) and progression-free survival (10.4 years vs. 4.0 years, p = 0.002) at a median follow-up of 11.9 years with the addition of PCV [44]. The role for chemotherapy alone was investigated in EORTC 22033, which randomized high-risk low-grade gliomas to either 12 cycles of post-operative dose-intense TMZ or RT (50.4 Gy) [45]. Preliminary results at a median follow-up of 3.8 years suggest no difference in progression-free survival between the two arms. 1p deletion was a positive prognostic factor irrespective of treatment. Patients with 1p-intact tumors showed a trend toward worse progression-free survival with TMZ alone (30 vs. 41 months, p = 0.06). Final results from this study as well as the CODEL trial discussed above will hopefully help elucidate the best initial adjuvant therapy for patients with 1p/19q co-deleted tumors.
Radiation Planning, Treatment Fields, and Dose
Quality magnetic resonance imaging (MRI) is critical to radiation treatment planning. Although computed tomography (CT) can visualize the tumor, MRI is significantly more sensitive to the extent of the tumor as well as its associated findings, including peri-tumoral edema. The most useful sequences on MRI are T1- and T2-weighed images. Glioblastomas are nearly always heterogeneously enhancing on T1-weighted images following administration of gadolinium contrast; anaplastic gliomas are more variably enhancing. T2-weighted fluid attenuation inversion recovery (FLAIR) images are the most useful series for full visualization of tumor-associated edema for high-grade gliomas. Low-grade gliomas most often are non-enhancing, thus, the FLAIR sequence is often the best sequence by which to visualize the tumor. Ideally, the extent of tumor resection and determination of residual disease should be assessed by performing a post-operative MRI within 24–48 h of surgery. By performing this study within this time period, residual tumor can be distinguished from post-surgical effects.
In the pre-CT and MRI era, whole brain irradiation was usually employed as adjuvant RT for malignant glioma. However, studies demonstrated that the majority of recurrences were within a few centimeters of the pre-surgical tumor [46, 47]. With the added impetus to reduce treatment-related toxicity of whole brain radiation therapy (WBRT), subsequent trials confirmed no benefit to whole brain irradiation compared to more focal irradiation [48]. Additionally, with the development of image-based RT planning, more localized RT was feasible. Utilization of both MRI and CT data for treatment planning is now standard of care [49]. Based on the tendency for malignant gliomas to infiltrate adjacent brain tissue, potential expansion across the midline via the corpus callosum should be considered when defining treatment volumes. The value of utilizing F-18 fluorodeoxyglucose (FDG) PET for definition of treatment volumes, particularly the boost volume, has been of recent interest. However, studies have not demonstrated improved survival with the use of FDG PET compared to historical controls [50, 51]. The value of PET for patients with glioblastoma continues to be investigated, particularly with regard to its potential role in assessing early treatment response or recurrence.
A typical RT plan involves an initial course of targeting the T2 hyperintense volume plus a 1–2 cm margin to encompass potential microscopic disease and treating this target volume to 45–50 Gy. This first course is followed by a boost to the T1 post-contrast tumor plus a 2–2.5 cm margin to a total dose of 60 Gy using 30 fractions of 2 Gy. Alternatively, the T1 enhancing and T2 hyperintense disease can be treated as a single volume with a margin to 60 Gy (see Fig. 14.1). A total dose of 60 Gy is the standard of care based on several studies that showed improved survival with dose escalation to 60 Gy [52–54].


Fig. 14.1
Clinical target volume (CTV) is outlined (pink) on representative slices at the same level from the planning computed tomography (CT), T1-weighted post-gadolinium, and T2-weighted FLAIR magnetic resonance imaging (MRI) series, respectively, from left to right. The planning CT is fused with the pre- and post-operative MRI images to aid with contouring of the target volume and neighboring critical structures. The lenses, retinae, optic nerves, optic chiasm, and brainstem are seen on this representative slice
Several other studies examined the utility of dose escalation beyond 60 Gy for glioblastoma using a variety of techniques including 3D conformal RT, [55] stereotactic radiosurgery, [56] brachytherapy, [57, 58] and particle therapy [59, 60]. However, even up to doses as high as 90 Gy, tumor recurrence often occurs in-field [59, 61] and dose escalation with brachytherapy failed to show a survival benefit in a randomized trial [57]. Radiation necrosis often proves the limiting factor preventing dose escalation to a larger volume and/or to a boost volume. Given the technical complexity of brachytherapy and the emergence of stereotactic techniques, interest in brachytherapy has declined in favor of stereotactic radiosurgery (SRS) or stereotactic radiation therapy (SRT). SRS and SRT will be discussed in more detail in the next section. Given continued poor outcomes with conventional radiation therapy and lack of efficacy of dose escalation, several other approaches have been studied, including hyperfractionation, [62, 63] without evidence of improved survival with altered fractionation. The doses for anaplastic gliomas are extrapolated from the glioblastoma trials and most often are 59.4 Gy in 1.8 Gy per fraction. An acceptable dose range for low-grade gliomas is 45–54 Gy; the most often prescribed dose is 54 Gy in 30 fractions.
The use of 3D conformal RT versus intensity-modulated RT (IMRT) is often decided upon based on tumor location. IMRT is an advanced type of radiation therapy that modulates the intensity of the radiation of each beam during treatment by utilizing computer algorithms to simultaneously optimize the tumor dose and minimize dose to normal structures. Dosimetric planning studies have shown superior target coverage and increased dose sparing to neighboring normal structures with the use of IMRT [64, 65]. IMRT is most beneficial when the tumor abuts critical radiation-sensitive structures such as the optic chiasm, optic nerves, pituitary gland, and/or brainstem. Figures 14.2 and 14.3 demonstrate the capacity of IMRT to increase the dose homogeneity and reduce the dose to critical normal structures. However, level I evidence examining clinical endpoints is lacking to support the use of IMRT over 3D conformal radiation therapy.



Fig. 14.2
A comparison of intensity-modulated radiation therapy (IMRT) and 3-dimensional conformal radiation therapy (3DCRT) for the same patient as in Fig. 1 with a resected glioblastoma. The top row is the IMRT plan as seen on the computed tomography (CT), T1-weighted post-gadolinium and T2-weighted FLAIR magnetic resonance images, respectively, from left to right. The bottom row is the corresponding 3DCRT plan. The clinical target volume (blue) was expanded by 3 mm to create the planning target volume (PTV) seen in pink. The PTV was prescribed 60 Gy in 30 fractions. The area receiving 62 Gy, also known as the “hot spot,” is visibly larger in the 3DCRT plan

Fig. 14.3
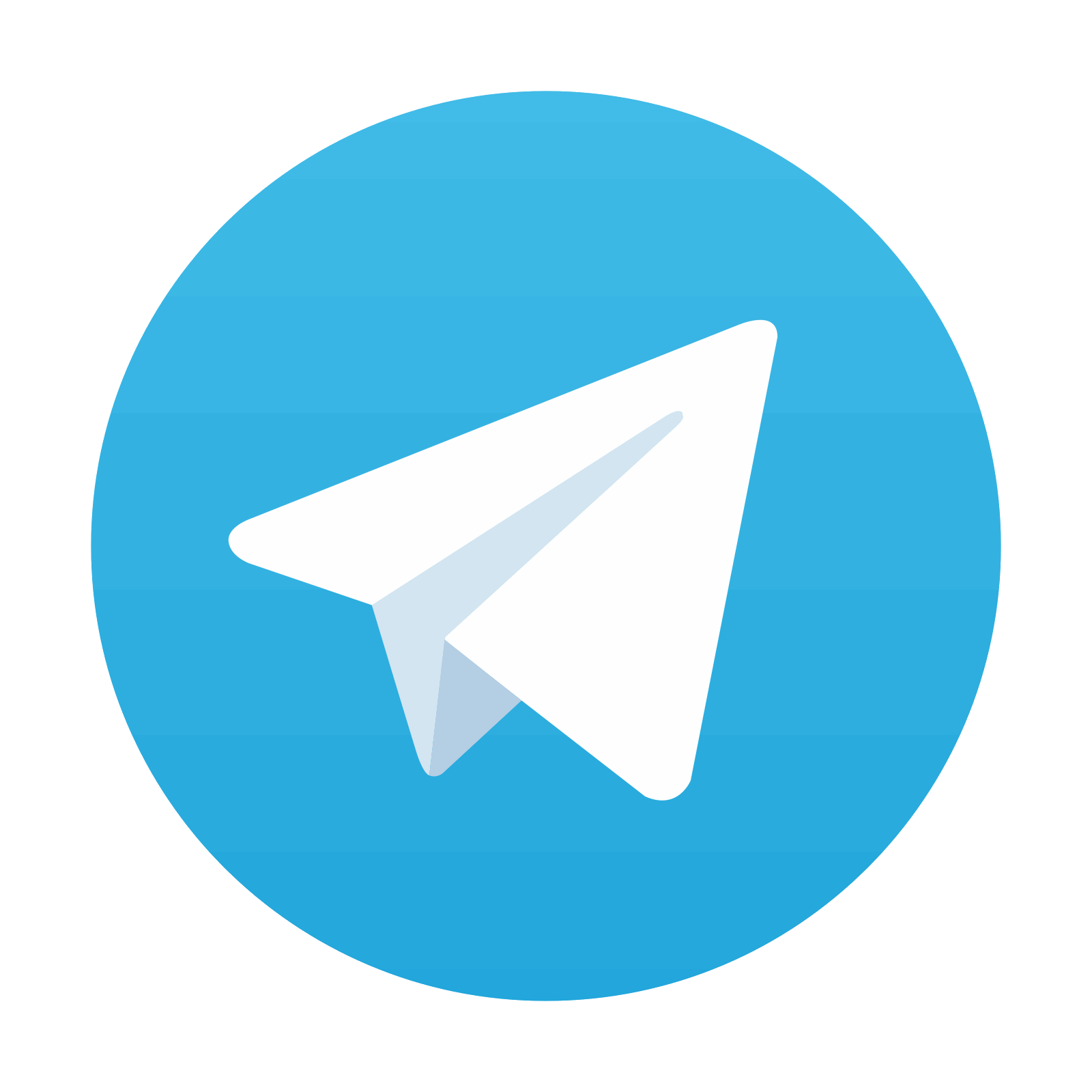
A dose–volume histogram (DVH) allows for evaluation of the dose received by the target volumes and critical normal structures. This dose–volume histogram compares the doses delivered by an intensity-modulated radiation therapy plan (IMRT, solid lines) as compared to a three-dimensional conformation radiation therapy plan (3DCRT, dashed lines). The vertical axis represents the proportion (%) of the total volume of a structure receiving a particular dose, which is represented on the horizontal axis. Coverage of the clinical target volume (CTV), and planning target volume (PTV) is improved and doses to the critical normal structures are lower with use of IMRT
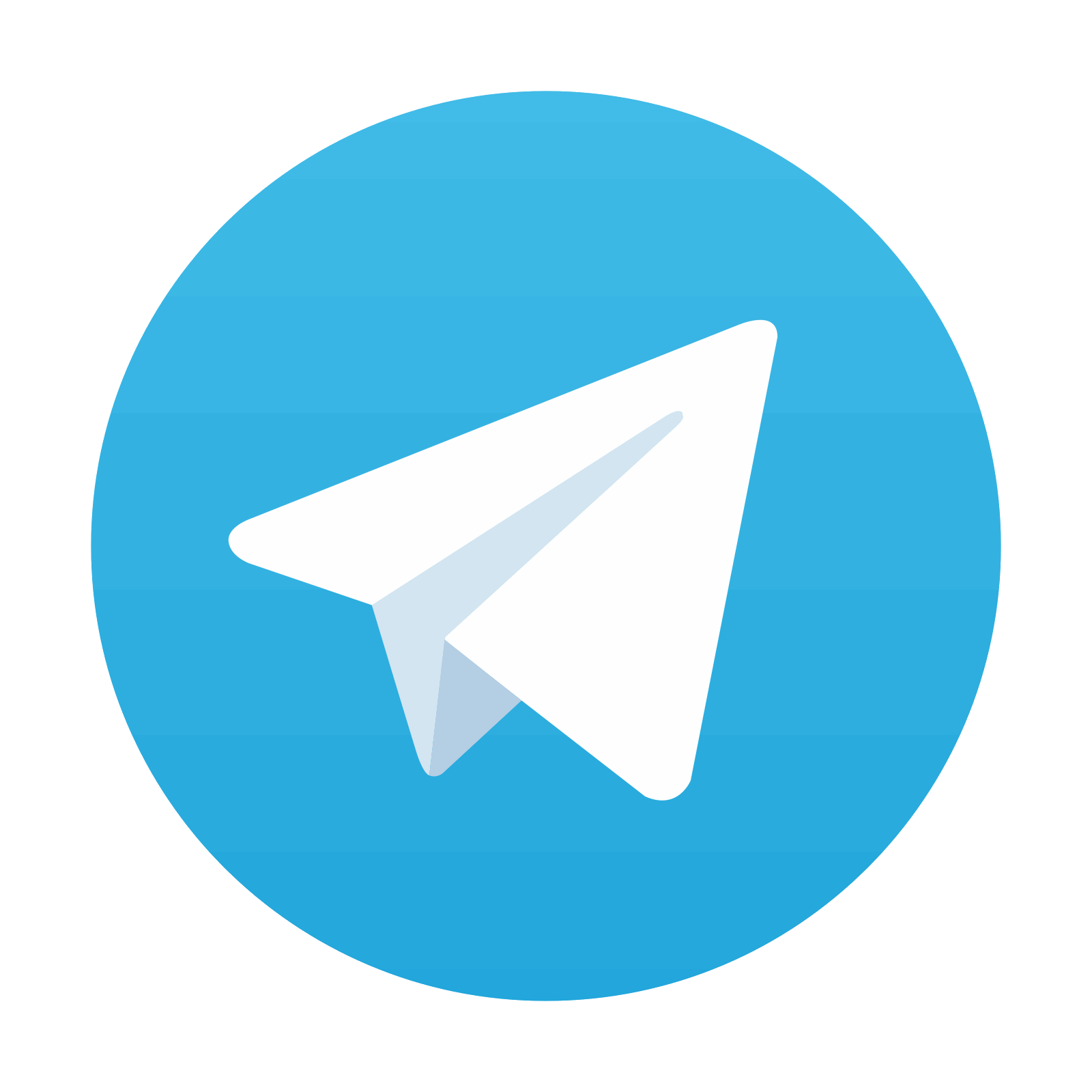
Stay updated, free articles. Join our Telegram channel

Full access? Get Clinical Tree
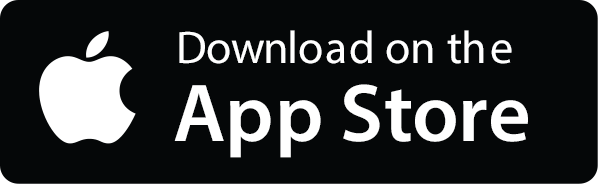
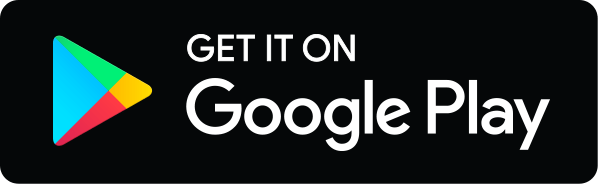
