Radiation Therapy for Pediatric CNS Malignancies
Pediatric central nervous system (CNS) malignancies represent the most common solid malignancy of childhood and account for the greatest number of cancer-related deaths for children.1 Radiation therapy is a well-established and effective therapy for many brain tumors and is frequently indicated for cure, delay of tumor progression, or palliation. For most CNS malignancies, trials attempting to omit radiation for the treatment of brain tumors have led to increased rates of tumor relapse.2–4 Unfortunately, for long-term survivors of brain tumors, morbidity may be substantial, and, for some children, it is in large part attributable to radiation therapy.5–9 Despite the reported toxicities of radiation therapy to the brain, most of the literature is from an era when treatment was delivered without contemporary techniques. Major advances in imaging and delivery of radiation therapy have been achieved over the past few decades, and with more accurate targeting of the regions at risk for recurrence and greater conformity of radiation delivery, decreased morbidity with improved outcomes is anticipated. Advances in radiation in addition to surgery, chemotherapy, and molecular agents detailed elsewhere in this book confer reason for great optimism in providing cure for many children without denying them the ability to lead a normal life.
Methods of Radiation Damage
Similarly to chemotherapy, radiation therapy targets rapidly dividing cells. In contrast to chemotherapy, radiation is a local rather than systemic treatment and its penetration into the CNS is not limited by the blood–brain barrier. Ionizing radiation produces changes in biological material by interacting with molecules in the cells of tumors and normal tissues (direct damage) or through the production of free radicals (indirect damage). Radiation therapy has been shown through experimental data to induce tumor cell death mainly through DNA double-strand breaks.10 Although some damage may be repaired, tumor cells have decreased ability to repair damage, and much of this damage is lethal. Healthy critical tissues generally have a greater ability to repair DNA damage.11 In addition, the more rapid cell cycle of tumor cells leaves them in a vulnerable stage of the cell cycle more frequently. Radiation schedules are derived from these principles, experimental data, and clinical experience.12 Generally, radiation is delivered in a fractionated course over several weeks to take advantage of these differences between normal tissues. Dose is prescribed in units of gray (Gy) and generally delivered in fractions of 1.5 to 2 Gy daily for 20 to 30 treatments (4 to 6 weeks).
Radiation Modalities
Therapeutic radiation therapy has been available since the late 1800s. For decades, radiation treatment planning has been executed using plain X-ray films with little detail of individual anatomy and dose distribution in the path of the therapeutic beam. Due to lack of radiographic detail of the area of tumor involvement or region at risk in the brain, large fields were necessary to ensure adequate target volume coverage. Consequently, a large area of normal brain received radiation therapy. Improvements in diagnostic imaging including computed tomography (CT) scanning and magnetic resonance imaging (MRI) improved the ability to more appropriately target regions requiring radiation therapy, but it was not until these imaging modalities were translated into modern radiation therapy planning software that a radiation treatment plan could be devised and manipulated with precision to provide enhanced dose delivery for the given radiation modality (photons, electrons, protons, etc.). Further improvements in software have led to computer-developed treatment plans better optimized for a given radiation treatment, known as intensity modulation. In addition to advances in imaging and treatment planning, advances have been made in radiation delivery. High-energy photons were further researched and developed, offering the ability to treat to greater depths in the body. Charged particles, particularly protons, have been explored for several years, but more recent advances in delivery have led to greater recognition of their potential benefits, particularly for pediatric patients.
Three-Dimensional Conformal Radiation Therapy
Three-dimensional conformal radiation therapy (3D CRT) entails delivery of radiation from multiple beam directions using fields that are shaped to the target volume in the view of the beam direction. It is made possible through the use of 3D images for treatment planning, generally performed with CT. CT images are obtained in the position planned for treatment using an immobilization device such as a mask or body mold to ensure reproducibility. Permanent marks on the device or patient are placed at the time of planning to allow for accurate positioning for treatment. The physician may combine other imaging studies (MRI, positron emission tomography [PET]/CT, prechemotherapy or presurgical imaging) to assist with delineation of the tumor volume, the region at risk of recurrence, and normal structures. Generally, a gross tumor volume (GTV) is defined as the visible tumor on the scan or the surgical cavity, and a clinical tumor volume (CTV) adds a margin for potential microscopic disease. Any healthy tissues that should be spared from radiation are also contoured. Beams delivering uniform dose are aimed at the isocenter, which represents the geometrical intersection of the axes of rotation around the patient.

Intensity-Modulated Radiation Therapy
Intensity-modulated radiation therapy (IMRT) is the most advanced form of photon radiation currently available. This technique enablss radiation to conform to the target volumes while decreasing the high dose to specified structures that may have a more limited tolerance to radiation. Similar to 3D conformal photon radiation, multiple beam angles that have fields conforming to the shape of the tumor are used. In addition, IMRT allows for modulation of the dose given from each beam and, in essence, delivers treatment with multiple nonuniform small beams to achieve greater ability to conform the dose to the tumor. This is accomplished by sophisticated computer planning systems that perform multiple iterations of beam intensity until the plan closest to the desired parameters for target volumes and normal structures is achieved.13 An advantage of IMRT is the sparing of high dose to critical structures. One of the major disadvantages of IMRT is that, as a result of achieving this conformity, increased low-dose radiation is usually delivered to a larger amount of normal tissue ( Fig. 11.1 ).
Stereotactic Radiosurgery
Stereotactic radiosurgery (SRS) refers to the delivery of a single fraction of radiation to a small volume of tissue and is generally delivered with multiple small beams. Due to the precision required, immobilization is of paramount importance, and a rigid frame is generally fixed to the patient′s head for treatment. This therapy generally involves collaboration with a neurosurgeon and can be delivered through a variety of techniques, including linear accelerator based SRS, Gamma Knife™, CyberKnife™, Xknife™, or proton therapy. Stereotactic radiation treatment may also be delivered over several small fractions if the size of the tumor is large or adjacent to critical structures. Figure 11.2 demonstrates stereotactic treatment of a tumor in the midbrain.
Proton Therapy
Proton therapy is a method of external radiation using charged particles—protons. The benefit of protons, in contrast to photons, is derived from the physical properties of the proton particle itself. Protons are charged particles that enter tissue delivering a small and relatively constant dose until near the end of the proton range, where the majority of dose is delivered over the last ½ cm to 1 cm of the path.14 This distal portion of the proton beam is defined as the Bragg peak, beyond which no dose is deposited. Photons, on the other hand, continue to deliver dose until they exit the body. Protons have a defined range or depth in tissue that is directly proportional to the energy of the beam. A proton beam can be produced that to treat any depth up to 32 to 35 cm in water, allowing for the treatment of deep-seated as well as superficial tumors.14 Photon range is also proportional to energy, but the depth at which the maximal dose is delivered is limited to approximately 3 cm. For conformal proton treatment, Bragg peaks of different energies are stacked to form what is referred to as a spread-out Bragg peak (SOBP). This increases the entrance or skin dose, but still allows full sparing of all tissues distal to the tumor volume, and the dose proximal to the target is still generally lower than the dose delivered with photons. In contrast, photons deliver a dose both proximal and distal to the target. Although there is some intensity modulation inherent in any proton plan, protons may also be delivered with multiple inhomogeneous small beams (intensity-modulated proton therapy [IMPT]) or scanning techniques. Biologically, protons are considered equivalent to photons when prescribing, after calculating a small adjustment for a relative biological effectiveness (RBE) of 1.1.15,16 Protons are prescribed in units of Gy(RBE) ( Table 11.1).

Protons are currently available in only a handful of centers; several centers have recently opened and many more are in the planning or construction phases. Given the higher capital and operating costs of proton therapy, it is important to select patients likely to derive a substantial clinical benefit from proton radiation. Recognizing the sensitivity of developing tissues, the risk of a radiation-induced malignancy, and the considerable cost to society of caring for children with chronic medical problems and children with developmental delay, curable pediatric CNS tumors represent a leading indication for proton radiation.
Specific Malignancies
Medulloblastoma/Supratentorial Primitive Neuroectodermal Tumor
Medulloblastoma is an embryonic neoplasm arising from the cerebellum. It accounts for approximately 20% of pediatric brain tumors and is the second most common pediatric brain tumor following pilocytic astrocytoma.5 Median age of onset is 5 to 6 years.17 At present, disease extent is categorized as standard risk or high risk. Standard risk is nonmetastatic disease in children over the age of 3 with ≤ 1.5 cm2 of residual disease following surgery. However, much has been discovered over the past decade about prognostic biological characteristics of the cells and signaling pathways for medulloblastoma, and this will lead to changes in risk stratification for these patients in the coming years.18 For patients with standard-risk disease receiving craniospinal radiation (CSI) and chemotherapy, cure rates exceed 80%.19 Survival rates are less favorable for patients with high-risk medulloblastoma, but 5-year disease-free survival rates of 60 to 70% can be achieved.19–21
Standard radiation therapy for medulloblastoma consists of treatment to the entire craniospinal axis at a dose of 23.4 to 36 Gy followed by a additional radiation to the posterior fossa or tumor bed plus a margin (involved field) to bring the total dose to this region to 54 Gy. Prior to the use of chemotherapy, 36 Gy CSI was considered standard, but based on outcomes of a phase II trial by Packer et al,19 23.4 Gy CSI followed by a boost to the posterior fossa to 54 Gy delivered with chemotherapy became the favored treatment for standard-risk disease, and the efficacy of the regimen has been confirmed in subsequent studies. Several publications indicate that treatment to the involved field may provide comparable outcomes with decreased morbidity compared with posterior fossa radiation, but this is still being investigated in the current Children′s Oncology Group (COG) study, ACNS0331, which has reached accrual for children over the age of seven and will complete accrual for children ages 3 to 7 in the near future. This trial also investigates reduced dose CSI (18 Gy) for children ages 3 to 7. Children′s Hospital of Philadelphia published outcomes for children under the age of 8 treated with 18 Gy CSI followed by additional radiation therapy to the tumor bed.22 At a median follow-up of over 10 years, seven of 10 children were disease free with decreased neurocognitive decline compared with a dose of 36 Gy.
For children with high-risk medulloblastoma or supratentorial primitive neuroectodermal tumor (SPNET), standard radiation includes a CSI dose of 36 Gy followed by a boost to a total dose of 54 Gy to the posterior fossa or tumor bed with chemotherapy. Modern techniques including IMRT and protons can provide improved sparing of neuroendocrine structures and the cochlea. Protons provide complete sparing of organs anterior to the vertebral bodies23 ( Fig. 11.3 ).
The management of disease in children under the age of 3 presents a particular challenge. CSI is considered to lead to unacceptable neurocognitive outcomes in these young children. Management generally consists of chemotherapy first, to delay radiation therapy, and delivery of radiation is often limited to the involved field for children younger than 3 years of age and has even been omitted in several studies.24,25 It has been difficult for cooperative groups to come to a consensus on a standard radiation approach for these young children. Outcomes are inferior, perhaps in large part due to the omission, deferral, or alteration of radiation therapy.
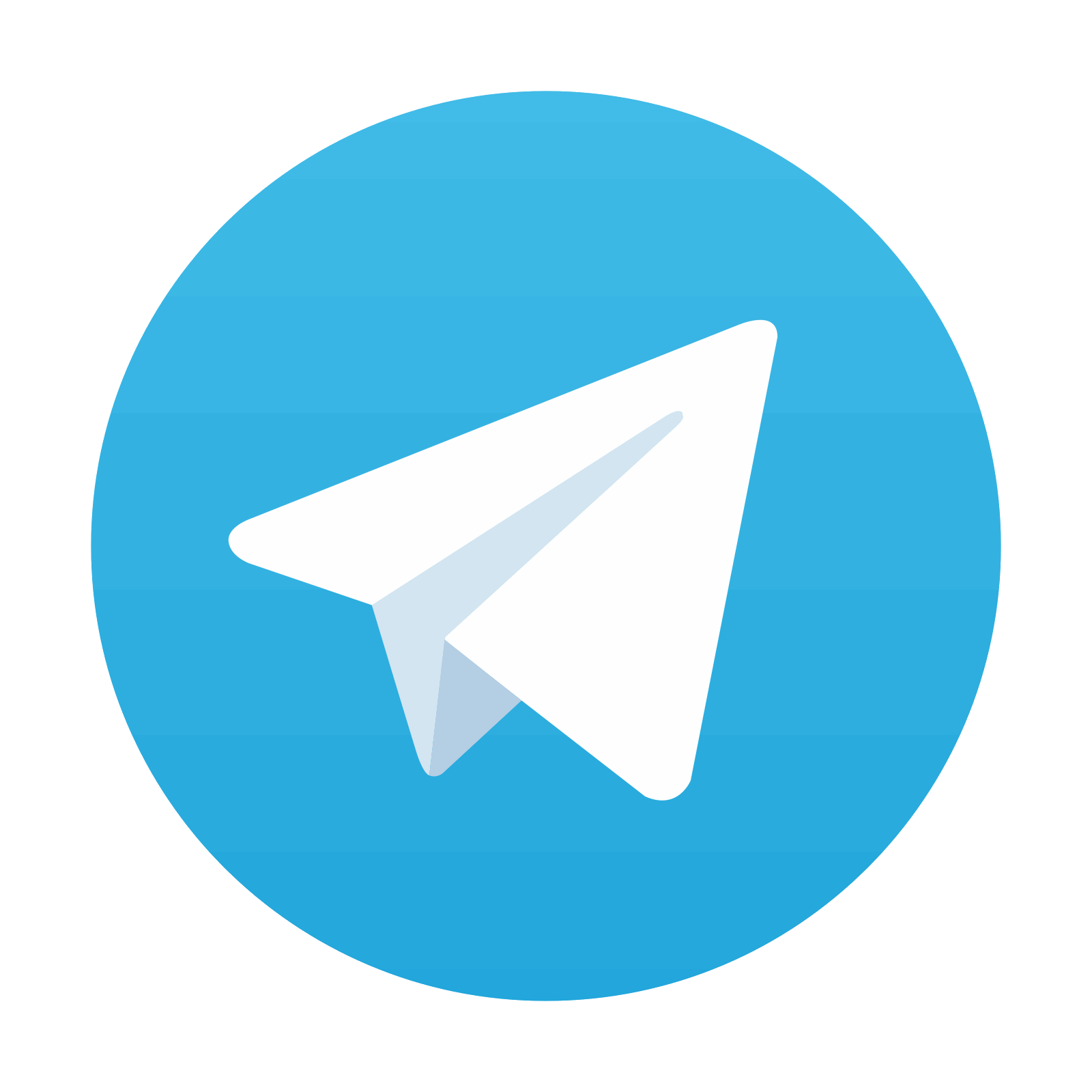
Stay updated, free articles. Join our Telegram channel

Full access? Get Clinical Tree
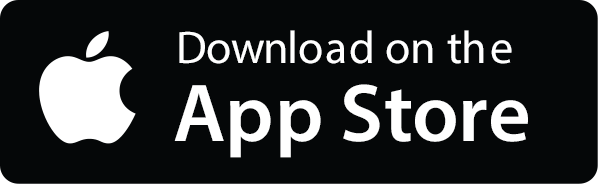
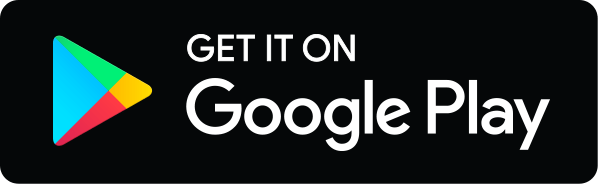
