Timing of radiotherapy
Low-grade gliomas
High-grade gliomas
At progression, usually after chemotherapy
Postoperatively
Dose
54 Gy in 1.8 Gy fractions
59.4 Gy in 1.8 Gy fractions
GTV
Tumor bed + solid and cystic tumor on T1 MTR
Tumor bed + solid and cystic tumor on T1 MTR
CTV
GTV + 1.0 cm
GTV + 2.0 cm
PTV
CTV + 3–5 mm
CTV + 3–5 mm
PFS 5 years
68–87 %
30 %
OS 5 years
80–100 %
60–66 %
30.3.1 Timing
In pilocytic and low-grade tumors, a complete resection does not require adjuvant radiation (RT) [7, 8].
In low-grade CAs after a complete resection, approximately 13 % of patients relapse, half of those occurring between 6 and 12 months of surgery [4]. In those not requiring surgery at recurrence, three of six in one series regressed, while the others had further treatment [4].
In the Mishra series, 5-year progression-free survival (PFS) rates were 79 % ± 13 % for patients treated with complete resection (CR), 60 % ± 8 % for those with subtotal resection (STR), and 46 % ± 8 % for those with biopsies alone. Five-year OS rates were 100 % for those treated with CR, 88 % ± 5 % for those with STR, and 89 % ± 5 % for those with biopsies alone. Ten-year PFS rates were 45 % ± 8 % for patients with STR and 30 % ± 8 % for those with biopsies alone. Ten-year OS rates were 82 % ± 6 % for patients with STR and 76 % ± 8 % for those with biopsies. The hazard ratio (HR) for PFS with a complete resection was 0.14 when compared to biopsy alone. This did not translate to a significant hazard ratio for OS [7].
In the setting of an incomplete resection, adjuvant chemotherapy may be considered either at initial diagnosis or at progression.
Low-grade tumors have been reported to spontaneously regress with no further treatment or following incomplete surgical resection [4, 8]. In Saunders et al., 5 of 11 patients who were observed after an incomplete resection progressed at a mean of 12 months; 5 of 11 showed tumor regression at a mean of 32 months [4]. The remaining patient progressed as a high-grade tumor. In the Netherlands, a retrospective review of 25 children (mean age 10.8 years) with pilocytic CAs who had undergone a subtotal resection showed that 15 progressed, 8 were stable, 1 spontaneously regressed, and the last regressed after irradiation [8].
A review by Palma revealed that incompletely resected pilocytic tumors remain stable in about one third of cases and spontaneously regress in about 14 % [9].
In the event of progression, radiation can be utilized if further surgery is not feasible or if there is an incomplete resection at the salvage procedure.
Against this approach, the Kidd series demonstrated increase relapses after radiation in the group who were treated at recurrence, rather than at the initial diagnosis (see below) [10]. However, only 5 of the 20 patients in this series were infratentorial.
Grade 2 tumors similarly do not necessarily require radiation as part of their initial management [7]. A retrospective review of grade 2 astrocytomas in children at Memorial Sloan Kettering showed similar PFS and OS when comparing those receiving RT at initial diagnosis versus no initial RT. The respective 5- and 10-year PFS rates were 59 % ± 13 % and 43 % ± 14 % for those not receiving radiation at diagnosis, as compared with 60 % ± 7 % and 44 %± 8 % for those who did. The respective 5- and 10-year OS rates were 93 % ± 6 % and 82 % ± 12 % for those receiving no radiation at diagnosis, as compared with 84 % ± 5 % and 77 %± 7 % for those who did. Importantly, this analysis removed children under 3 and those who had undergone a gross total resection to remove selection bias for radiation [7]. Only 10 % of the patients had infratentorial tumors in this study.
In the adult setting, a large randomized EORTC study showed no difference in overall survival in those who were observed (7.4 years, 5 years) compared to those offered early radiation (7.2 years). There was a significantly reduced progression-free survival in the observation group (55 % 5 years PFS vs 35 %), with the observation group having a longer time from relapse to death (in part due to the use of radiation as salvage therapy) [11].
In high-grade tumors, consideration may be given to intensive chemotherapy to delay the need for radiotherapy in children under 3–4 years of age [6]. However, if it is considered that the supratentorial volumes can be minimized and thus the neurocognitive effects reduced, then initial irradiation may be preferable [5, 12]. In very young patients (<3 years old) with high-grade tumors, an appropriate strategy is to achieve a maximal safe resection followed by adjuvant chemotherapy with radiation held in reserve until progression or relapse [2].
30.3.2 Radiation Dose
The results of the prospective EORTC study 22844 of dose response in low-grade gliomas in adults demonstrated no significant difference in survival or PFS between administration of 45 and 59.4 Gy in 379 patients [13].This was also seen when allowance was made for incomplete versus complete resection. Interestingly however twice the number of patients (26 vs 13) in the higher-dose arm had interruptions to their radiotherapy of a week or more, and nine of those had treatment discontinued.
In the prospective St Jude series [5, 14] of conformal radiotherapy for low-grade gliomas, a dose of 54 Gy was used in 1.8 Gy per fraction (#), over 6 weeks. There were 17 cerebellar tumors in this cohort of 78. Similar fractionation schedules have been reported by other groups [3, 10].
As a result, many centers use 54 Gy as their routine dose for low-grade gliomas.
In high-grade gliomas, there have been conflicting results regarding dose response. In the adult population there were equivalent results between 35 Gy in 10 #s and 60 Gy in 30 #s in Phillips’ study, although it was closed due to poor accrual [15]. In a recent pilot study, Beauchesne et al. [16] examined thrice daily (TDS) radiation of 0.75 Gy up to 67.5 Gy total and found that, in comparison to the EORTC-NCIC 26981-22981 study, the OS and PFS (5.1 and 9.5 months, respectively) were superior to conventional radiotherapy alone, but not to RT and temozolomide.
An older study of hyperfractionation to adult gliomas (61.4, 71.2, and 80 Gy) showed no improvements in OS between the dose groups [17]. Another Japanese series [18] using 60–80 and 90 Gy in 2 Gy per fraction 5 days per week showed no improvement in the overall survival of patients with differing doses, but there were fewer local failures in the 90 Gy group (94/13 vs 16 of 19).
Most centers and studies use radiation of 54–60 Gy, with a recent St Jude series of high-grade gliomas (in children) with temozolomide mandating 59.4 Gy in 1.8 Gy fractions.
30.3.3 Radiation Volumes
The volume for irradiation in cerebellar astrocytomas is local conformal. A clinical and autopsy series [19] (in adults) demonstrated that in 15 patients with infratentorial glioblastoma, there was no evidence of CSF spread, indicating no need for craniospinal irradiation (CSI). However a small study from Toronto [20] demonstrated that three of four pediatric patients with cerebellar glioblastoma ultimately relapsed in the spine; similarly a Japanese series had five consecutive patients with malignant cerebellar astrocytomas relapsing with leptomeningeal disease [21]. Other authors have suggested CSI in this group [22]. Some centers have given craniospinal irradiation for glioblastomas, but this is not a routine in the absence of CSF seeding [3].
It is essential that dedicated spinal MRIs and CSF cytology be performed prior to planning radiotherapy to determine whether craniospinal irradiation is required.
In adult series, the majority of failures of non-JPA astrocytomas occur in the previously treated field with only a few at the field edge [23].
In the St Jude series [5, 24], the gross tumor volume (GTV) was taken to be the solid and cystic tumor seen on multisequence MRI before radiotherapy. A 10 mm expansion was made to the clinical target volume (CTV), and the planning target volume (PTV) was a 3–5 mm expansion on the CTV depending on the degree of immobilization. In this group (overall) there was only one marginal failure, with four failing metastatically and eight being local failures. The marginal failure was in a patient with optic glioma.
Jalali has reported using a 5 mm GTV-CTV expansion with a 3 mm PTV expansion in low-grade and benign tumors with similar results [25].
Thus, the use of a 10 mm GTV-CTV expansion is considered safe for low-grade tumors.
In high-grade tumors such as glioblastoma, larger margins are needed. In a landmark paper, Halperin et al. performed autopsy studies on 15 adults who had died of GBM [26]. Neuropathological analysis was performed identifying malignant cells, and the distribution was traced onto diagrams of the whole-mount histological sections. Radiotherapy plans were carried out using the most recent CT scan, with reference to the contrast-enhancing area and edema. In nine patients, a 1 cm margin on the contrast-enhancing area would have missed the identified tumor on histology. Expanding the volume to the tumor, the edema and 1 cm would have covered only six patients, whereas a 3 cm margin covered all the tumor in all patients. There was evidence of tumor tracking along neural pathways and crossing of the corpus callosum.
In a clinical study, Wallners group looked at patterns of failure in AAs and GBMs treated with irradiation. Using the CT contrast enhanced as the initial tumor volume, 56 % of patients recurred within 1 cm and 78 % within 2 cm. All patients received whole brain radiotherapy of the order of 40 Gy with the majority having a boost to 51–70 Gy. There was no mention as to the margins used for the boost therapy.
Kelly et al. from the Mayo Clinic took 195 samples from 40 patients and found that contrast enhancement most often corresponded to tumor tissue without intervening parenchyma; hypodensity corresponded to parenchyma infiltrated by isolated tumor cells or in some instances to tumor tissue in low-grade gliomas or to simple edema, and isolated tumor cell infiltration extended at least as far as T2 prolongation on magnetic resonance images in both high- and low-grade tumors.
Extrapolating these results to children, a margin of CTV expansion of 2 cm from the GTV, the contrast-enhanced mass (T1 post-gadolinium or tumor bed) plus the associated T2 or FLAIR abnormalities, is appropriate [27].
The use of functional imaging in brain tumors is also increasing. In a series of 36 patients, pretreatment methionine PET scans were performed in patients with glioblastoma. Of 19 patients with uptake in the tumor, the five who had activity outside the high-dose radiotherapy area all had noncentral failures; as compared to 2 of the remaining 14 who had activity inside the high-dose radiotherapy area [28]. MET PET has also been shown to be a strong predictor of disease involvement when used to guide stereotactic biopsies (61 of 61 in 32 patients) [29]. It has proved useful in modifying the surgical volumes in a large Belgian series in both high- and low-grade tumors [30].
It is quite likely that functional imaging will become integrated into radiotherapy planning as an adjunct to MRI and CT.
30.4 Technique
30.4.1 Modality
The increasing complexity of planning systems in addition to other technological advances means that treatment can be offered with less damage to the normal brain tissue than the previous [12, 14, 31].
Conformal 3D noncoplanar plans have become the standard of care in many institutions. The addition of noncoplanar beams allows relative sparing of the temporal lobes and the subventricular/hippocampal regions implicated in late neurocognitive complications [12, 31, 32]. Figures 30.1, 30.2, 30.3, and 30.4 demonstrate the benefits of a six-field noncoplanar plan versus a three-field coplanar plan.



Figs. 30.1 and 30.2
A three-field coplanar field arrangement showing dose distribution (Fig. 30.1) and the dose volume histogram (DVH) for the plan in Fig. 30.1 (Fig. 30.2)

Figs. 30.3 and 30.4
The same tumor volumes now planned with a six-field noncoplanar arrangement. Note the reduction in temporal lobe dose (Fig. 30.3) and the DVH for the plan in Fig. 30.3. Note the improvement in temporal lobe doses (Fig. 30.4)
Intensity modulated radiotherapy (IMRT) has been used in posterior fossa tumors. The main advantage is in reducing the inner ear/cochlear dose to less than 30–35 Gy which should result in less hearing loss, particularly high-frequency deafness [33–35]. IMRT therapy does increase the volumes of the normal brain receiving low doses which may theoretically increase the incidence of second malignancies and may also contribute to [36] some late neurocognitive effects [12].
Using stereotactic radiosurgery of 12–18 Gy to the 50–80 % isodose line, results have been reported equivalent to standard conformal therapy in grade 1 tumors [10]. In a series from Tapei which examined gamma knife treatment for low-grade gliomas, by covering the T1- and T2-weighted abnormalities with a median of 14 Gy, the 10-year PFS was 65 %. All local failures in this series were within the high-dose volume, including patients receiving SRS after progressing through initial therapy [23]. Similar results using a median of 15 Gy have been reported by Hadjipanayis et al. [37] and Kano et al. [38].
The number of centers offering proton therapy has increased in the last decade and in many countries has become a viable option for patients. Proton therapy has the benefit of reducing auditory apparatus dose and sparing the temporal lobes from both low- and high-dose exposure [36, 39]. There is no difference reported in PFS or OS in the low-grade glioma group [40].
30.4.2 Results
The survival of CAs is highly dependent on the histology [41, 42]. Glioblastomas that are EGFR immunonegative appear to be more radiosensitive and thus have better control rates [43]. In a large series of CNS tumors, Morris et al. [44] found that a 15-year overall survival for low-grade gliomas was approximately 90 %, whereas for high-grade gliomas it was around 60 %. In the Swiss series [1] of pilocytic astrocytomas, there was a 100 % 5-year OFS and 96.8 % 10-year OFS.
Functional imaging is proving to be important in the predictions of outcome. FET PET showing uptake with a diffuse pattern on MRI in low-grade gliomas has a poorer outcome than diffuse tumors with no uptake; the best outcomes occurred in circumscribed tumors with no uptake [45].
Saunders group treated 12 children with RT for low-grade tumors with regression or stabilization first occurring at a mean age of 6 months with complete changes at 14.9 months. Only one child progressed [4].
Kidd et al. similarly found 100 % OS at 5 years and 80 % at 10 years in pediatric patients receiving radiotherapy for grade 1 tumors. The 5-year progression-free survival was 68 %, with those having upfront therapy faring better than those after disease progression (77 % vs 50 %, p = 0.013) [10]. The infratentorial group fared better than the supratentorial group (80 % PFS 5 years vs 59 %). Patient age had no impact on outcome.
In the Merchant series [5, 14], the overall survival was 98.5 % at 5 years and 95.9 % at 10 years, the event-free survival was 87.4 % (5 years) and 74.3 % (10 years). The 5- and 10-year local failure rate was 8.7 and 16.4 %, respectively.
For high-grade gliomas, overall the prognosis is better than in the adult setting with a 5-year OS of 66 % but a 5-year event-free survival of 28 % [2].The median survival of adults with high-grade cerebellar astrocytomas is of the order of 14 months [14]. In some series, there is no difference in outcomes for AA versus GBM in children under 3.
30.4.3 Surveillance
In low-grade tumors, the need for routine surveillance is less marked than in high-grade tumors, ependymomas, or medulloblastoma [4]. The Saunders group recommends routine surveillance imaging for low-grade CAs at 6 months, 1, 2, 3.5, and 5 years after either complete surgical resection or radiotherapy [4]. In the incompletely resected group, they recommend surveillance at six monthly intervals for 3 years, then yearly for 2 years, and two yearly thereafter [4].
Dirven’s group recommends fewer scans again; surveillance scans at 1 and 4 years for complete resection and yearly for residual disease [8].
In the future, the response to treatment using functional imaging may become an important tool to guide the frequency of assessment.
30.4.4 Toxicity: Acute
Acute side effects of posterior fossa radiotherapy are generally mild and easily managed. The most common side effects are alopecia, otitis, and effects consistent with peritumoral swelling (headache, nausea, and vomiting) [11].
In the weeks and months after radiotherapy, changes in the capillary permeability and transient demyelination may cause headaches, somnolence, fatigability, and worsening of the initial presenting symptoms. This occurs in up to 60–70 % of children receiving cranial radiation and is proportional to the whole brain DVH [46, 47].This effect is self-limiting and usually recovers after 2–3 weeks. It should not progress into further neurological compromise [46, 48, 49].
30.5 Intermediate Imaging Changes
In the weeks and months following radiotherapy for low-grade tumors, routine imaging may reveal an increase in asymptomatic edema and/or cyst development [14, 38]. The latter effect may even occur during the radiotherapy treatment itself. This should settle spontaneously but may necessitate surgical drainage of the cyst should symptoms develop [14]. It can be mistaken for a local recurrence and therefore may cause significant distress to patients, their families, and the treating team. If there is doubt, then functional imaging or a biopsy may be required. Mostly repeat imaging is all that is needed approximately 6 weeks later.
30.6 Toxicity: Late
The impact of treatment on later health is significant in children who have had brain tumors. The incidence of death due to medical complications occurred 25 times more than expected in the matched population in Morris’ review of the St Jude series [44]. This is in addition to the significant health and functional effects that the treatment of brain tumors entails.
30.6.1 Neurocognition
There is limited data on the long-term toxicity of cerebellar astrocytomas. Much of the appropriate data comes from series which examine gliomas at any site or radiation given for other posterior fossa tumors.
It is important to appreciate that radiotherapy alone is not the sole cause of neurocognitive impairment in children with brain tumors. More surgical complications are associated with a sharper decline in later IQ [50]. Increased inattentiveness has been observed in children undergoing two or more surgical procedures as compared to one [51]. Hydrocephalus requiring a shunt has been linked to impaired attention, slowed processing, and lower scores on the Child Behaviour Checklist [5, 51–54]. Results are also better before radiation in children who have a biopsy only in comparison to a subtotal resection [5].
Some chemotherapeutic agents have been implicated in reduced neurocognitive functioning, particularly intrathecal methotrexate [55] although this is not routinely used in the management of astrocytomas. The most common agents used in CAs (temozolomide and carboplatin) have not been shown to affect cognition. However, in Merchant’s series [5] a trend was seen to higher scores on visual auditory learning (10.7 points p = 0.0983) in those not treated with chemotherapy.
The Aarsen series [56] examined 35 patients with infratentorial tumors of whom 15 required remedial teaching and seven needed special education. The majority had received radiotherapy postoperatively. Importantly, the mean preoperative bicaudate index of hydrocephalus was 0.22 in this group, compared to 0.13 in the supratentorial group. They also found that, compared to the normal population, these patients had significantly lower scores on verbal intelligence, sustained attention, speed (cancelation test speed), long-term visual-spatial memory, executive functioning, and naming. Further sub-analysis showed that children with a cerebellar PA had significantly lower scores on all these tests with exception of verbal intelligence in comparison to the norms [56].
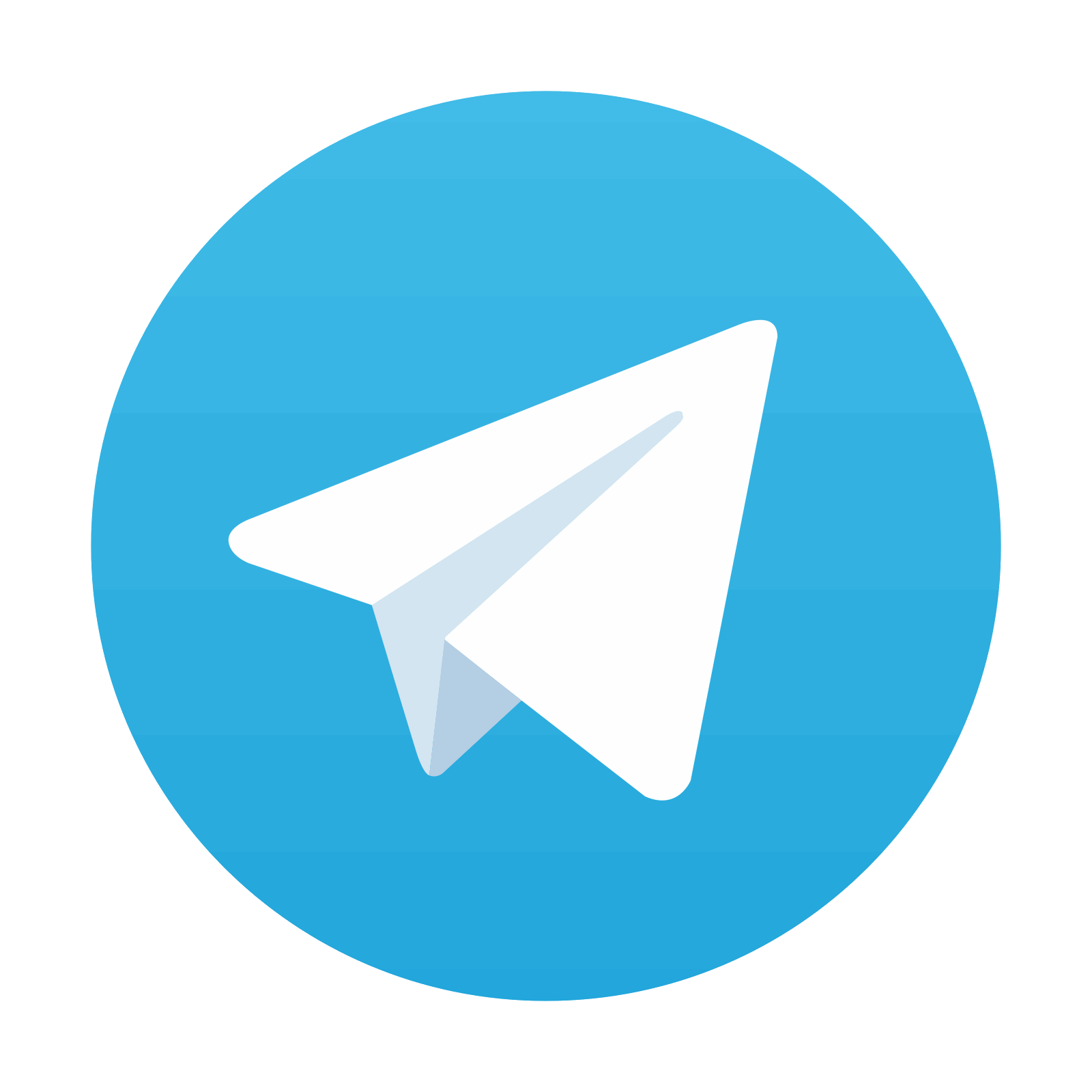
Stay updated, free articles. Join our Telegram channel

Full access? Get Clinical Tree
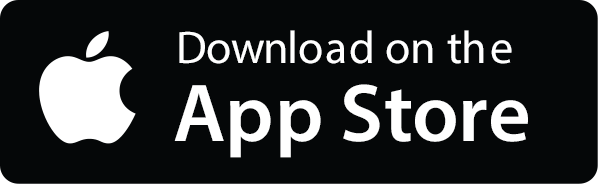
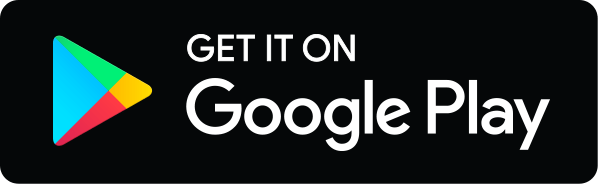