Radiotherapy of Pediatric Brain Tumors
During the past 20 years, the role of radiation therapy in the treatment of childhood brain tumors has increased owing to the promise of new treatment methods and an improved understanding of the indications, critical parameters, and side effects attributed to this modality. Future investigations will tailor the selective use, sequencing, and escalation and de-escalation of radiation dose and volume. Advancement beyond conformal and intensity-modulated radiation therapy is anticipated with the use of proton therapy. The latter has been used only in its earliest form (passively scattered or three-dimensional conformal proton therapy) and will soon give way to the preferred method of spot scanning, which will allow intensity-modulated proton therapy.
51.1 Medulloblastoma
Medulloblastoma is the most common malignant brain tumor in children and presents at a median age of 5 to 7 years. The negative consequences of this tumor and the side effects of irradiation are offset only by the knowledge that radiation therapy has curative potential, in even the most advanced cases.
51.1.1 Prognostic Factors
Prognostic factors for medulloblastoma include the extent of disease and resection, age at presentation, and the intensity and quality of radiation therapy and chemotherapy. The effect of age at presentation is highly correlated with the selection of treatment and the delay or omission of radiation therapy in the front-line management of the disease in very young children. More recently, histopathologic and molecular subtyping studies have identified small subgroups for which survival is excellent or very poor.1–4 How the histopathologic and molecular grouping affects the use of radiation therapy remains to be determined.
51.1.2 Radiotherapy
Several decades ago, the standard of care for children with medulloblastoma included surgery and craniospinal irradiation; chemotherapy was experimental. Craniospinal irradiation included treatment of the neuraxis, including all subarachnoid volumes contiguous with the central nervous system (CNS) and cerebrospinal fluid (CSF) pathways, and supplemental (“boost”) irradiation of the primary site. The neuraxis component of therapy received 36 Gy, and the boost volume, formerly the anatomical posterior fossa, received approximately 54 Gy.
Treatment of Standard-Risk Medulloblastoma
The use of craniospinal irradiation changed markedly when investigators explored reducing the craniospinal dose for patients characterized as having standard-risk disease (i.e., those without metastatic disease or substantial residual or extensive tumor). In 1999, the Children’s Cancer Group CCG-9892 study showed that the addition of postradiation chemotherapy (lomustine, vincristine, and cisplatin) to a reduced-dose (23.4 Gy) craniospinal irradiation regimen resulted in a 5-year progression free survival (PFS) of 79%; thus, the addition of chemotherapy to the treatment regimen permitted a 12.6-Gy reduction in the craniospinal dose without affecting the rate of tumor progression.5 Since then, little has changed therapeutically in terms of disease control. Indeed, the confirmatory A9961 study (conducted between 1996 and 2000 and reported in 2006) showed that a postradiation regimen of vincristine, cisplatin, and lomustine resulted in a 5-year event-free survival (EFS) rate of 82%; the combination of vincristine, cisplatin, and cyclophosphamide resulted in a rate of 80%.6 The latter regimen had a slightly higher rate of acute toxicity, and some concern remains about the high incidence of secondary tumors in this treatment group.
Complications
The classic portals for the boost volume included a pair of parallel opposed beams targeting the entire posterior fossa with margin. When this type of treatment was delivered to very young children, the loss of IQ was approximately 4.2 points per year, even at the reduced craniospinal dose of 23.4 Gy.7 Children who likely started out with a low–average IQ would be expected to have a subnormal IQ within 3 years after completing radiation therapy. With no plateau, intellectual disability would be expected by 5 to 6 years after treatment. It was concluded that unless the method of primary site irradiation (posterior fossa boost) was modified, very little could be done to change the volume receiving the highest (> 45 Gy) doses.
The first prospective trial to reduce the dose to the posterior fossa was conducted between 1996 and 2003 in children with standard-risk medulloblastoma, defined as T1 to T3B and M0 disease according to the Chang staging system.8 Postoperative residual disease measuring less than 1.5 cm2 received craniospinal irradiation (23.4 Gy), conformal posterior fossa irradiation (36 Gy), and conformal primary site irradiation (55.8 Gy) with a 2-cm clinical target volume margin. Radiation therapy was followed by high-dose chemotherapy with peripheral blood stem cell support. Children treated with this regimen, which reduced the volume of the posterior fossa receiving the prescribed boost dose by almost 15%, had a 5-year EFS rate of 83.0% (standard deviation [SD], 5.3%), and no change was noted in the pattern of treatment failure. This modest reduction in the volume receiving the highest dose lowered IQ loss to only 2.4 points per year.9
Emerging data strengthen the association between the distribution of dose to regional volumes of normal brain and decline in cognitive function in several domains. The negative effect on IQ and academic test scores associated with increasing mean dose was shown for the entire brain volume, supratentorial brain volume, left and right temporal lobes, and left hippocampus (IQ, math and reading scores).10 Proton therapy is an excellent measure to reduce extra-CNS and CNS doses in these patients: photon dose volume data associated with a decline in IQ are graphically inferior to proton dose volume data for similar patients.11
Another important and devastating side effect of radiation therapy is CNS necrosis. Among 148 patients who received 23.4 Gy of craniospinal irradiation and 88 patients who received more than 36 Gy of craniospinal irradiation, the cumulative incidence of CNS necrosis at 5 years was 3.7% (SD, 1.3%).12 Those patients in whom CNS necrosis was observed had the largest infratentorial volumes, receiving doses in excess of 50, 52, and 54 Gy, providing new limits of tolerance for children with medulloblastoma and other CNS embryonal tumors treated with aggressive surgery, craniospinal irradiation, and intensive chemotherapy.
51.1.3 Treatment Alternatives
Biology is likely to have an effect on risk stratification in future trials. Because 30% of patients present with metastatic disease, extent of disease will remain an important factor. By histopathology, 10% of patients have desmoplastic medulloblastoma and a favorable prognosis, 74% have classic medulloblastoma and an intermediate prognosis, and 16% have anaplastic medulloblastoma and a poor prognosis. According to the biological subgroup classification, 13% of patients have Wnt pathway activation and an excellent prognosis, 17% have sonic hedgehog activation and a less favorable outcome, and the remaining 70% have biological tumor subtypes and a range of outcomes ranging from average to poor. Options for managing patients who have standard-risk disease with favorable clinical–pathologic and molecular risk features include omitting radiation therapy altogether, omitting craniospinal irradiation, and reducing the dose to the neuraxis and primary site. For patients with high-risk disease, considerations include intensifying the radiation dose and volume, administering chemotherapy and radiation therapy concurrently, and escalating the dose at the primary site if local treatment failure is the associated pattern of failure.
51.1.4 Medulloblastoma in Patients Younger than 3 Years
Some of the greatest recent changes in how medulloblastoma is treated have been in the youngest patients. More recent studies have used radiation therapy in the front-line management of these patients following an approximately 4-month-long induction phase of postoperative chemotherapy. The Pediatric Brain Tumor Consortium PBTC-001 study reported in 2005 used the standard four agents of cisplatin, vincristine, cyclophosphamide, and etoposide, in conjunction with intrathecal mafosfamide, followed by radiation therapy.13 Radiation therapy was risk-stratified on the basis of age and extent of disease and ranged from 45 Gy (cumulative primary site dose) for the younger and lowest-risk group (after gross total resection) to 54 Gy for the older patients and those with residual disease at the time of irradiation. The 5-year PFS rate exceeded 50% in the cohort of children in whom craniospinal irradiation was omitted.
In the Children’s Oncology Group (COG) A9934 study, the use of similar systemic chemotherapy without intrathecal therapy and focal irradiation to 50.4–54 Gy resulted in a 4-year PFS rate of 50% (SD, 6%).14 PFS rates were improved by using a strategy of initial surgery; induction chemotherapy for 4 months; second surgery when necessary; and age-, risk-, and response-adaptive conformal radiation therapy with posterior fossa treatment and primary site irradiation, followed by 8 months of chemotherapy with conventional agents. The A9934 study included irradiation with a 10-mm clinical target volume margin. Patients whose disease progressed during induction chemotherapy experienced primary site treatment failure, whereas those with disease progression after radiation therapy experienced neuraxis failure in the spine or regions of the brain that were spared by the omission craniospinal irradiation (▶ Fig. 51.1). The newer regimens that prescribe early but not immediate postoperative radiation therapy have a very high rate of local tumor control.
Fig. 51.1 Sagittal computed tomographic scan of a very young child (age younger than 3 years) with medulloblastoma treated in the Children’s Oncology Group A9934 study by conformal radiation therapy (ca. 2000). Dose gradient from 5,040 to 500 cGy is apparent. Low-dose regions were the site of metastatic failure after radiation therapy.
51.1.5 Reducing Radiation Dose and Volume
As outlined earlier, clinical–pathologic and molecular risk classification will help to stratify patients for treatment. Radiation dose and volume may be able to be reduced in some children with medulloblastoma. Strategies for achieving such reductions include reducing the craniospinal dose to 18 Gy, as was done in the COG ACNS0331 trial; reducing the cumulative dose to the primary site to 45–50 Gy, as was done in the PBTC-001 and A9934 trials; and reducing the clinical target volumes for the boost component of therapy. Other strategies to consider are partial irradiation of the tumor bed and radiation therapy methods that spare normal tissue, such as intensity-modulated photon therapy or one of the current or developing methods of proton therapy.
51.2 Ependymoma
Ependymoma is the third most common brain tumor in children. The incidence is highest in very young children, which is relevant when the indications for radiation therapy are considered. In children, ependymoma most often arises in the infratentorial compartment and is intimately associated with neurovascular structures and the brainstem. This is important because of the normal tissue dose constraints that one must consider in planning radiation therapy. Supratentorial ependymoma may arise in the lining of the ventricular system or within brain parenchyma. In these cases, the postoperative tumor bed may range from a simple spherical structure to a large intracranial cavity, with associated subdural hygroma or complex or unidentifiable tumor volume.
51.2.1 Three-Dimensional Radiation Therapy
In 1993, the International Commission on Radiation Units and Measurements outlined a nomenclature for target volumes and three-dimensional radiation therapy planning. These recommendations were first adopted for the treatment of ependymoma in the St. Jude Children’s Research Hospital RT1 trial. The gross tumor volume was defined as the residual tumor and/or the tumor bed; the clinical target volume was an expansion of the gross tumor volume, with an additional margin of 10 mm that was anatomically constrained at barriers where tumor invasion was unlikely (e.g., base of skull, calvaria, tentorium); and the planning target volume (PTV) was a margin meant to account for geometric uncertainty (3 to 5 mm). The trial included 153 patients treated between 1997 and 2007. The 7-year EFS and overall survival (OS) rates were 72% (SD, 6%) and 81% (SD, 5%), respectively. The 7-year local control rate was 83% (SD, 5%), the 7-year cumulative incidence of local failure was 16.3% (SD, 3.4%), and the 7-year cumulative incidence of distant metastasis was 11.5% (SD, 2.9%).15
51.2.2 Reducing Radiation Volume
The initial experience with three-dimensional planning for ependymoma included multiple beams at various orientations pointed at the planning target volume. Each beam had the beam’s eye view shape of the planning target volume. The intersection of the beams results in a dose distribution that is adjusted and weighted to shape the prescribed dose (54 to 59.4 Gy) to the planning target volume. When treatment plans were initially developed with this early three-dimensional treatment-planning process, it was apparent that large volumes of normal brain could be spared in the process of treatment planning and delivery.
These methods were further refined to include intensity-modulated radiation therapy, a more sophisticated form of three-dimensional conformal radiation therapy in which computation-intensive iterative planning is used to balance dose constraints for normal tissues against target volume coverage goals. The prospect of sparing normal tissues in a young and vulnerable patient population accelerated referrals for systematic conformal radiation therapy in the late 1990s and likely led to the more aggressive preradiation optimization of patients to minimize the amount of residual disease before irradiation. A targeting example for ACNS0831, the current COG trial, appears in ▶ Fig. 51.2.
Fig. 51.2 Sagittal computed tomographic scans of a child with infratentorial ependymoma after gross total resection targeted for radiation therapy according to the ACNS0831 Children’s Oncology Group guidelines. The postoperative tumor bed defines the gross tumor volume (upper, left), surrounded by a 5-mm anatomically constrained clinical target volume margin (upper, center) and a geometrically expanded 3-mm planning target volume margin (upper, right) targeted to receive 5,400 cGy. The second phase of treatment, an additional 540 cGy, targets the gross tumor volume, excluding the portion that is adjacent to the upper cervical spinal cord (lower, left and center). The modified gross tumor volume is geometrically expanded by 3 mm to create a second planning target volume (lower, right).
51.2.3 Complications
Cognitive outcomes were reported along with disease control for the patients with ependymoma in the St. Jude Children’s Research Hospital RT1 trial.16 No decline in IQ was noted for the entire group, including the cohort of younger children (age younger than 3 years). The latter group had lower baseline values and no decline with time. With additional follow-up, now extending to more than 10 years, evidence that conformal radiation therapy spares cognition remains apparent. As an example, no clinically significant declines occur in the academic achievement of children with ependymoma treated with conformal radiation therapy.17 Similarly, verbal and visual auditory learning18 and adaptive behavior19 appear to be spared in children with ependymoma treated with focal conformal radiation therapy.
One of the added benefits of conformal radiation therapy is the ability to associate more precise estimates of radiation dose with functional outcomes. The dose to the hypothalamus has been directly correlated with the incidence and time to onset of clinically significant endocrine deficiencies in children with ependymoma and other brain tumors. Growth hormone secretion is the most common endocrine deficiency and the most sensitive to radiation dose.
Neurologic effects of irradiation on motor and sensory function and coordination are rare and are most often associated with infrequent cases of radiation necrosis. However, the effect of irradiation on recovery from tumor-related and surgery-acquired deficits should be considered, especially in children with infratentorial ependymoma after surgery for locally extensive disease. Prospective data suggest that the radiation dose does not impede recovery of neurologic function in children with ependymoma.
One additional aspect of neurologic function to consider is hearing loss after radiation therapy. Hearing loss as a function of time after radiation therapy is relatively uncommon, especially when the total dose to the cochlea can be limited to 45 Gy.20 Thus, preservation of hearing is largely achievable in most patients when the target volume margins and parameters of some of the aforementioned clinical trials are used. With smaller clinical target volume margins, more precise methods of localization and verification, and intensity-modulated radiation therapy or proton therapy, irradiation of the cochlea with doses that will result in hearing loss should be infrequent unless the internal auditory canal is part of the intracranial volume at risk.
51.3 Low-Grade Glioma
Low-grade gliomas make up approximately 26% of childhood CNS malignancies seen in the United States.21 Low-grade gliomas are a heterogeneous group of tumors that are located most commonly in the cerebellum, followed by the cerebral hemispheres, the deep midline structures, the visual pathways, and the brainstem. Observation after gross total resection is the standard of care and can produce PFS rates of 80% for grade II tumors and more than 90% for grade I tumors.22 Tumors may demonstrate an indolent natural history after incomplete resection, but the PFS rate at 5 years is only 55%.23 Therefore, neurologic symptoms, progression on imaging, or risk for progression at a critical site necessitates treatment with either radiation or chemotherapy. The choice of treatment remains controversial, particularly for younger children.
51.3.1 Treatment Alternatives
The sequencing of therapy is affected by physician bias, patient age, tumor location and grade, risks associated with progression, severity of symptoms, and lack of randomized evidence. Some studies show that immediate adjuvant radiotherapy improves PFS rates,24–26 and one study suggests that such treatment improves seizure control.24 Adjuvant radiotherapy after incomplete surgical resection increases the 10-year PFS rate from 40 to 82%.26 More controversial is the benefit of immediate radiotherapy on survival in this setting, with only one study showing that immediate radiotherapy offers a survival benefit.27 A trend toward survival benefit for radiotherapy has also been shown for World Health Organization (WHO) grade II tumors.28 However, the majority of studies demonstrate no survival advantage with the addition of radiotherapy.24,26,29,30 Therefore, after an incomplete resection, a course of observation is usually recommended for asymptomatic patients who are not at severe risk for neurologic compromise if the tumor progresses. The addition of radiotherapy to chemotherapy has demonstrated a PFS benefit over chemotherapy alone31; however, the ideal timing and sequence of radiotherapy and chemotherapy remain unknown.
51.3.2 Three-Dimensional Conformal Radiotherapy
Radiotherapy techniques have improved over time. A Phase II trial of three-dimensional conformal radiotherapy resulted in excellent 10-year EFS and OS rates of 74% and 96%, respectively.32 The tumor volumes were based on magnetic resonance images, and a 1-cm clinical target volume margin was used. Among 78 patients, 13 developed treatment failure: 4 with metastatic progression, 1 with marginal failure, and 8 with in-field failure. All patients were prospectively evaluated to determine the effect of conformal radiotherapy on cognitive abilities, hearing, and endocrinopathies.33 It is important to note that patients with more aggressive surgery up front or with neurofibromatosis type 1 and younger children had lower baseline neurocognitive function before radiotherapy. Also, 24% of tested patients had growth hormone abnormalities before the initiation of radiotherapy. Cognitive effects 5 years after conformal radiotherapy correlated with patient age, neurofibromatosis type 1 status, tumor location and volume, extent of resection, and radiation dose. The effect of age exceeded that of radiation dose, and patients younger than 5 years experienced the greatest decline in cognition.
51.4 Optic Pathway Tumors
Radiation therapy is highly effective for optic pathway tumors, with 10-year PFS rates higher than 80%.34–37 The initial choice of therapy does not affect survival, but when radiotherapy is administered, PFS rates at both 5 and 10 years are improved.22,34,38 Many patients with optic pathway tumors present at a young age, with 25% younger than 18 months and 50% younger than 5 years.39,40 For children younger than 2 years, younger age and diencephalic syndrome are poor prognostic factors.41 After combination chemotherapy, progression has been noted at a median of 3 years from treatment.42 At the time of progression, second-line chemotherapy, surgical resection, and radiation therapy need to be considered, with a decision made by a multidisciplinary team. Data suggest that patients who undergo surgery before radiation therapy enter treatment with better visual acuity and have better long-term outcomes43 (▶ Fig. 51.3).
Fig. 51.3 Visual acuity before and after radiation therapy based on eye condition and surgical intervention. Log MAR 0 = 20/20; log MAR 1 = 20/200. Log MAR, logarithm of minimal angle of resolution. (Source: Awdeh RM, Kiehna EN, Drewry RD, et al. Visual outcomes in pediatric optic pathway glioma after conformal radiation therapy. Int J Radiat Oncol Biol Phys 2012;84(1):46–51.43)
51.5 World Health Organization Grade I Tumors
WHO grade I astrocytomas include juvenile pilocytic astrocytoma and subependymal giant cell astrocytoma and are generally characterized by well-circumscribed lesions.44 Juvenile pilocytic astrocytomas are most commonly in the cerebellum and the diencephalic region; only 3% of patients have disseminated disease. Subependymal giant cell astrocytomas develop along the lining of the ventricles and are associated with tuberous sclerosis.
The primary treatment for WHO grade I tumors is maximal safe resection. The 15-year survival rate for completely resected tumors is 90%.45 Radiation is recommended for symptomatic or progressive disease that cannot be resected or for residual disease with the potential to grow and damage critical structures.
Infratentorial tumors are more likely to be resectable,35 whereas tumors in the deep tissues of the brain are less likely to be completely resected; therefore, they are likely to benefit from radiotherapy.45 Children with deep-seated tumors treated with surgery and radiotherapy have a 15-year survival rate of 64%.45 The recently changed WHO criteria now classify ganglioglioma as a WHO grade I tumor. These tumors do not respond as well to irradiation or chemotherapy as their pilocytic counterparts do.
51.6 World Health Organization Grade II Tumors
WHO grade II gliomas include diffuse astrocytoma, pleomorphic xanthoastrocytoma, oligodendroglioma, oligoastrocytoma, and pilomyxoid astrocytoma.44 These tumors are more likely to progress than are WHO grade I tumors. A report of 90 children with WHO grade II glioma describes 5-year OS and PFS rates of 90% and 56%, respectively, with 10-year rates of 81% and 42%, respectively.39 Of note, for patients older than 3 years without a gross total resection, the early administration of radiation does not appear to influence PFS or OS rates: the 10-year PFS rate is 43% whether the patients receive radiotherapy early or at the time of progression. A 15-year cumulative incidence of malignant transformation of 6.7% has been reported from a group of patients with low-grade (grade II) gliomas and did not correlate with the use of radiotherapy.46
51.7 High-Grade Glioma
High-grade gliomas, including anaplastic astrocytoma (WHO grade III), anaplastic oligodendroglioma (WHO grade III), and glioblastoma multiforme (WHO grade IV), comprise 10 to 20% of pediatric brain tumors. These tumors are most commonly found in the cerebral hemispheres, thalamus, or basal ganglia. The molecular profile of childhood high-grade glioma is different from that of adult high-grade glioma and more often resembles that of a secondary glioblastoma multiforme, with an abundance of TP53 mutations. Standard treatment for these aggressive tumors includes maximum safe resection followed by radiotherapy and chemotherapy, given either concurrently or sequentially. Gross total resection (i.e., > 90% tumor resection) confers a clear PFS benefit.47–49 The most recent clinical trial reported 3-year EFS and OS rates of 11% ± 3% and 22% ± 5%, respectively, for a treatment regimen that included the administration of temozolomide concurrently with and after irradiation.50 These results were inferior to those from a prior series47 that included radiation therapy followed by multiagent chemotherapy. The respective 3-year and 5-year OS rates are 35% and 36% when children receive multimodality therapy.47,51 However, patients in a Phase II trial did not benefit from high-dose chemotherapy given before radiotherapy.51
51.8 Brainstem Glioma
The term brainstem glioma refers to both focal (20%) and diffuse intrinsic (80%) tumors.52 Patients with diffuse intrinsic tumors typically have a dismal prognosis, with a median OS of no more than 12 months.52–57 A short duration of presenting symptoms correlates with a worse survival.58,59 Attempts to improve outcomes have included hyperfractionated radiotherapy with total doses ranging from 66 to 78 Gy, resulting in median survival times of 8 to 13 months.60–67 A French trial reported improved survival of 17 months when front-line chemotherapy was used before radiotherapy; however, the chemotherapy caused significant infections and increased hospitalization.68 Concurrent chemotherapy has not demonstrated a benefit,63,69,70 nor have other strategies, including selective blood–brain barrier disruptors combined with chemotherapy,71 concurrent inhaled carbogen,54 intravenous recombinant interferon-β,72 and adjuvant chemotherapy.73
51.9 Germ Cell Tumors
Pure germinomas, nongerminomatous germ cell tumor (NGGCT) subtypes (i.e., embryonal carcinoma, endodermal sinus tumor, choriocarcinoma), and teratomas comprise up to 70%, 20%, and 20% of all germ cell tumors, respectively. Together, these tumors account for approximately 4% of pediatric CNS neoplasms.32,74 They typically arise in the midline of the third ventricle, most commonly posteriorly in the pineal region, or by the anterior recess of the third ventricle.75
51.9.1 Treatment Alternatives
Treatment for germ cell tumors continues to evolve over time and is based on the tumor type. Before safe biopsy of these tumors became available, radiotherapy was used as a diagnostic tool. If a tumor demonstrated a quick response to a radiation dose of 20 Gy, then it was classified as a germinoma.76,77 Now, stereotactic biopsy is often used to obtain a diagnosis and has a reported mortality rate of 1.3% and a neurologic morbidity rate of 3%.78 Radical resection offers no benefit over biopsy alone for intracranial germinoma.79 However, there is a benefit to surgical resection for NGGCT, in the form of both more accurate diagnosis74 and better overall outcomes,80 when it is combined with chemotherapy and radiation.
Radiation therapy is curative for pure germinoma, with reports documenting 10-year cause-specific survival and OS rates of up to 100% and 83%, respectively, for pathologically verified germinoma.77,81,82 A single-institution experience demonstrated 5-year rates of spinal axis failure and intracranial failure of 49% and 45%, respectively, for patients treated with partial-brain radiotherapy, compared with rates of 0% and 6% for those receiving whole-brain radiotherapy or craniospinal irradiation.82 The addition of chemotherapy has allowed volume and radiation dose to be reduced with equally good results.83–85 Prospective trials from the Société Internationale d’Oncologie Pédiatrique (French Society of Pediatric Oncology) and the Japanese Study Group show that relapses occur within the ventricles when involved-field radiotherapy (IFRT) is used after chemotherapy and have now changed their radiotherapy target to whole-ventricle irradiation (WVI) following chemotherapy.86–88
Survival rates of patients with NGGCTs treated with chemotherapy followed by craniospinal irradiation with a boost to the primary site range from 67 to 74%.80,89 A Phase II COG trial for NGGCTs (ACNS0122) investigated neoadjuvant chemotherapy followed by 36 Gy of craniospinal irradiation and a boost to the primary tumor site or resection cavity for a total dose of 54 Gy. The patients who experienced a complete or partial response at the end of induction or who had mature teratoma or fibrosis at the time of second-look surgery had excellent 3-year EFS rates ranging from 85.7 to 94.1%.90 Therefore, ACNS1123 is investigating the role of reduced-volume radiotherapy (30.6 Gy of WVI and 54 Gy of IFRT) for patients with a good prognosis. The Japanese Study Group has reported outcomes of the treatment of patients with an intermediate prognosis with five cycles of carboplatin and etoposide, followed by WVI to 30.6 Gy and IFRT to 50 Gy, with 10-year PFS and OS rates of 81.5% and 89.3%, respectively.88
51.10 Craniopharyngioma
Although craniopharyngioma in children is a histologically benign tumor, management with surgery and radiotherapy may be associated with varying morbidity. Complications include neurologic deficits, panhypopituitarism, diabetes insipidus, cognitive deficiencies, behavioral problems, visual disturbances, vasculopathy, malignant transformation, and secondary malignancies.91–97 Great care should be taken when therapeutic decisions are made for these children.
Craniopharyngioma can be managed with a gross total resection alone; however, even when gross total resection is confirmed by neuroimaging, the rate of recurrence is from 20 to 27%.98,99 Recurrence after surgery is related to tumor size, residual calcifications detected by computed tomography, and history of prior surgery or irradiation.98,99 The alternative to radical surgery is limited surgery and focal irradiation. The St. Jude Children’s Research Hospital experience suggests that fewer complications occur after a combined-modality approach and a minimal extent of surgery have been used.100 Ten-year PFS rates from 84 to 100% have been reported when patients undergo limited surgical resection followed by radiotherapy.101–103 In pediatric patients with craniopharyngioma, several factors are significantly associated with worse neurocognitive outcomes: age younger than 7.4 years, more extensive surgery, multiple surgical procedures, diabetes insipidus, hydrocephalus, CSF shunt, shunt revisions, Ommaya reservoir laterality, and cyst aspirations.104 IQ is also affected by radiation dosimetry factors, including percent volume of total brain, supratentorial brain, and left temporal lobe receiving doses greater than 45 Gy.
Radiation oncologists should be aware that tumor cyst expansion during treatment is common and that serial magnetic resonance imaging should be performed, with consideration given to developing a new plan when there is a risk for target volume compromise.105 Similarly, consideration may be given to cyst aspiration or decompression during radiotherapy without delaying radiation treatments. ▶ Fig. 51.4 displays modeled IQ data after surgery and radiation therapy for craniopharyngioma, with the effects of age and diabetes insipidus (a marker of the extent of surgery) shown.
Fig. 51.4 IQ modeled as a function of time after irradiation, age, and diabetes insipidus. DI, diabetes insipidus.
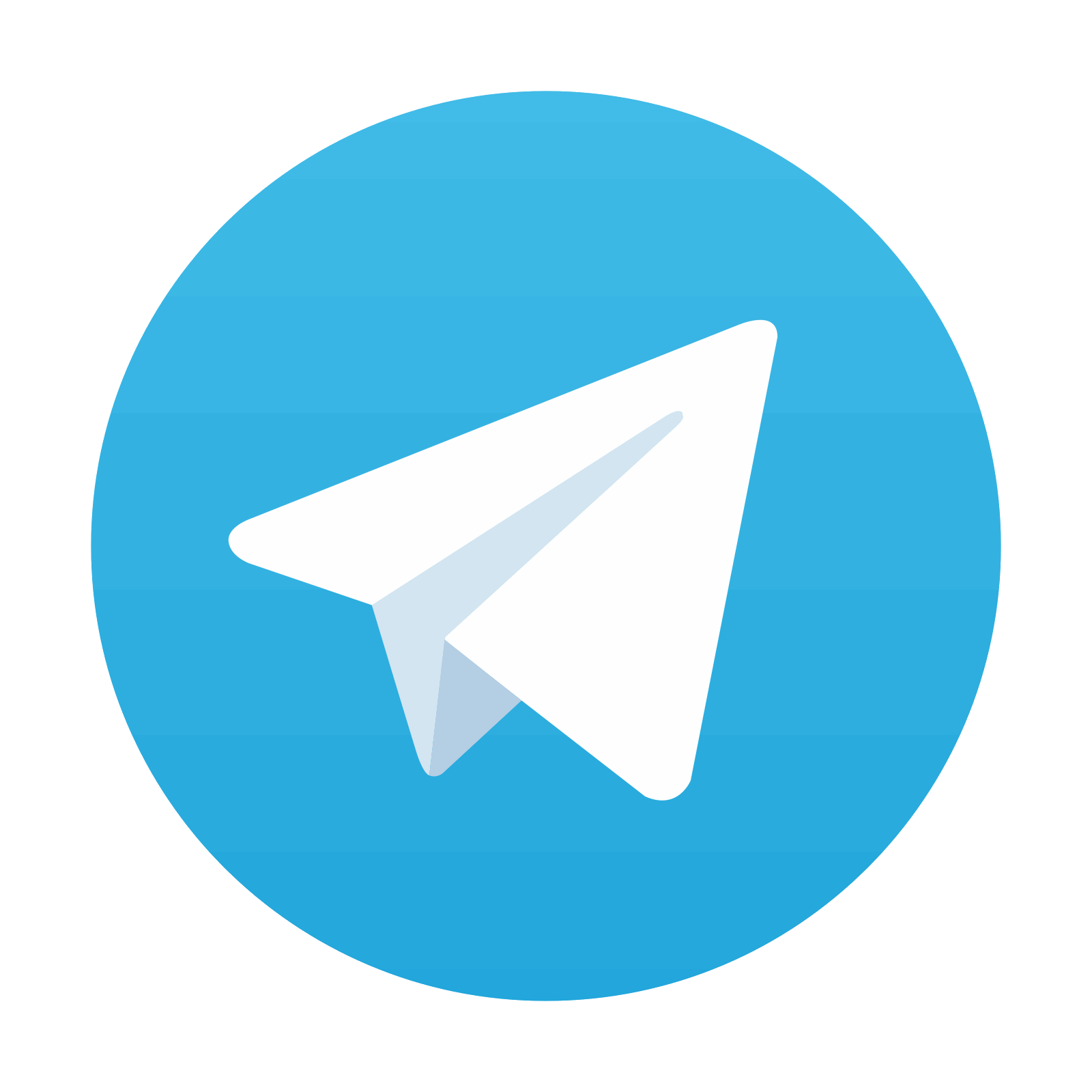