Fig. 1
Orexin receptor actions on brainstem arousal system neurons. Actions of orexin receptors on tyrosine hydroxylase positive (TH+) neurons from the locus coeruleus (LC), tryptophan hydroxylase positive (TPH+) dorsal raphe (DR) neurons and neuronal nitric oxide synthase positive (nNOS+) cholinergic laterodorsal tegmental (LDT) neurons. Arrow thickness indicates strength of functional coupling based on responses in slices from mice lacking one or the other OX receptor. Responses were determined using whole-cell recording and Ca2+ indicator fluorescence changes. See text for details
4 More Than Simple Excitation—Orexin Current Noise Provides High-Frequency Input During the Slow Depolarization
From the original electrophysiological description of orexins as excitatory peptides in primary cultures, it was clear that orexins could increase the rate of spontaneous transmitter release from terminals as manifest by increases in the miniature EPSC and IPSC (mEPSC/mIPSC) frequency (van den Pol et al. 1998). One or both of these presynaptic actions, have been observed in some, but not all central neurons studied in brain slices, suggesting orexin can play different roles in different circuits. These findings also suggest that orexin peptides do more than simply excite soma-dendritic targets (for a more general discussion, see Leonard and Kukkonen 2014). Emerging evidence indicates that in addition to modulating the strength of synaptic connections, orexins also influence the integrative properties of their target neurons.
In one example, we recently reported that in addition to providing a depolarizing input to serotonergic DR neurons and cholinergic LDT and PPT neurons, the noisy orexin cation current provides substantial high-frequency input at physiologically relevant frequencies (Ishibashi et al. 2015b). Measurement of the spectral properties of the orexin current revealed that the spectral amplitude significantly increased in theta, alpha, beta frequency bands and approximately doubled in the gamma frequency band for cholinergic LDT and PPT neurons, while in serotonergic DR neurons, this more than tripled across all of these frequencies.
While noise often reduces system reliability and sensitivity, noise can also confer beneficial effects. This is apparent in non-linear systems with a threshold where noise can selectively augment the effects of small signals through a process termed stochastic resonance. Hence, we propose that orexin current noise provides high frequency input to enhance the effectiveness of small EPSPs through stochastic resonance.
In this same study, we found that input of a virtual noisy orexin conductance to cholinergic LDT and PPT neurons, using a dynamic clamp activated an intrinsic Ca2+-dependent resonance that peaked at theta and alpha frequencies. Neuronal resonances depend on the interplay between the passive and active membrane properties and function to boost the effectiveness of inputs occurring within the resonance bandwidth. Thus, we propose another function of the orexin noise is to engage the intrinsic resonance which could enhance inputs during the waking and REM states and help promote firing of these cholinergic LDT and PPT neurons during these states (Boucetta et al. 2014).
5 More Than Simple Excitation—Orexin Modulates the Late AHP and Alters Neuronal Firing Properties
In addition to producing a slow depolarization, orexins can slow spike repolarization and reduce the post-spike after hyperpolarization (AHP) (Yang and Ferguson 2003; Yang et al. 2003; Horvath et al. 1999; Murai and Akaike 2005) although the underlying channels have not been identified nor have the consequences of this modulation been studied. Recent findings in paraventricular thalamic neurons, indicate that orexins also can inhibit the long-lasting slow AHP (I sAHP) that builds up following multiple spikes (Zhang et al. 2010). Although the underlying channel of the Ca2+-dependent K+ current are not identified, the I sAHP is found in many neurons where it powerfully influences firing pattern. It is also a well known target for modulation and is suppressed by activation of both Gs and Gq coupled neurotransmitter receptors (for review Andrade et al. 2012). This current, produces strong spike-frequency adaptation and inhibition of this current by orexins enhances the excitability of these neurons to sustained depolarizing input (Zhang et al. 2009, 2010).
Recently, we found a novel effect of orexin-A to enhance a late after hyperpolarization following single and multiple spikes in serotonergic dorsal raphé neurons. These neurons have a large, medium AHP, mediated by small-conductance (SK) Ca2+-activated K+ channels, that promotes slow firing and rapid spike-frequency adaptation (Aghajanian and Vandermaelen 1982). We found that in addition to producing a noisy depolarization, orexin enhances this K+ current and induces a longer-lasting, unidentified Ca2+-dependent late AHP current that appears distinct from I sAHP. Together, these enhanced outward currents increase spike-frequency adaptation and limit firing by slowing steady-state firing and reducing excitability (Ishibashi et al. 2015a).
Collectively, these diverse and potentially opposing modulatory actions imply that orexin receptors function to can powerfully tune how their target neurons respond to other inputs. Future studies aimed at understanding the diversity of mechanisms and impact of these emerging actions could provide important insights into how orexins normally regulate information flow in the brain and how this leads to perturbed circuit function in the absence of orexins in narcolepsy.
6 What Are the Behavioral Consequences of Orexin Receptor Signaling at Cholinergic and Monoaminergic Neurons of the Ascending Arousal System?
As described above, OX1 receptors drive excitation of noradrenergic LC and cholinergic LDT neurons and both receptors drive orexin excitation of serotonergic DR neurons. To gain insight into the functional consequences of orexin receptor signaling at these neurons we compare the changes in orexin excitation to the behavioral impairment reported for these knockout mice.
Mice with constitutive knockouts of both orexin receptors (DKO mice) have a narcolepsy phenotype similar to prepro-orexin knockouts (Chemelli et al. 1999; Mochizuki et al. 2004) and show fragmented waking, sleep attacks and cataplexy (Kalogiannis et al. 2011; Hondo et al. 2010; Hasegawa et al. 2014). Since a large fraction of LDT, DR and LC neurons are stimulated by orexin, the absence of orexin signaling at these loci in DKO mice is consistent with a role in promoting wake and sleep consolidation, suppressing REM sleep, preventing sleep attacks and suppressing cataplexy. Indeed, selective excitation of orexin neurons promotes sleep-wake transitions (Adamantidis et al. 2007) and selective inhibition of orexin neurons promotes slow-wave sleep and reduced firing of DR neurons (Tsunematsu et al. 2011). Moreover, focal orexin injection into the LDT (Xi et al. 2001) or LC (Bourgin et al. 2000) prolongs waking bouts and suppresses REM sleep. Importantly, inhibition of TH + LC neurons using optogenetics blocks the ability of selective stimulation of orexin neurons to promote sleep-wake transitions (Carter et al. 2012), while stimulation of TH + LC neurons prolongs waking (Carter et al. 2010) and enhances the wake-promoting effect of orexin neuron stimulation (Carter et al. 2012). Thus, orexin–excitation of TH + LC neurons appears necessary for the acute ability of orexin neurons to generate transitions to arousal from sleep. This suggests OX1 signaling in LC might also be critical for promoting consolidated waking bouts. A recent receptor rescue study, supports this idea since wake consolidation was increased in proportion to the number of TH + LC neurons that re-expressed OX1 receptors in constitutive DKO mice (Hasegawa et al. 2014). This appeared quite specific since re-expression of OX1 receptors in PPT or OX2 receptors in either TMN or DR did not increase wake bout duration in DKO mice. Nevertheless, additional factors must play a role since OX1−/− mice do not have fragmented sleep-wake states or sleep attacks (Mieda et al. 2011; Hondo et al. 2010), even though orexin excitation is abolished in LDT and LC neurons and is reduced in DR neurons (Kohlmeier et al. 2013). Thus, OX1 excitation of LDT, DR and LC neurons is not necessary for consolidated wake bouts, at least when OX2 receptor signaling is intact.
In contrast, OX2 knockouts have sleep attacks at the same frequency as do prepro-orexin knockouts (Willie et al. 2003) and have shorter wake bouts even though orexin-mediated excitation of LDT, DR and LC neurons is intact in these mice. Thus, orexin excitation of LDT, DR and LC neurons is not sufficient for the expression of normally consolidated bouts of spontaneous waking in the absence of OX2 signaling.
These above findings stress the importance of OX2 receptor signaling in consolidating wakefulness, yet re-expression of OX2 receptors in DR did not increase wake bout duration in DKO mice (Hasegawa et al. 2014) but re-expression of OX2 in the tuberomammillary region did prolong wake bout duration in OX2−/− mice (Mochizuki et al. 2011), but not in DKO mice (Hasegawa et al. 2014).
Collectively, these data are consistent with both OX1 signaling in LC and OX2 signaling in the TM region but not the DR in contributing to consolidation of wake activity. As noted by Hasegawa et al., it is important to interpret results from re-expression experiments with great cation since the effectiveness of the rescue will depend on many factors including re-expression efficacy and the degree of impairment to be overcome.
Cataplexy is another important impairment of narcolepsy, and cataplexy-like arrests are frequent in the dark phase of orexin ligand deficient mice (Chemelli et al. 1999; Mochizuki et al. 2004) and DKO mice (Kalogiannis et al. 2011). Since OX2−/− mice have rare cataplexy compared to prepro-orexin null mice (Willie et al. 2003), the attenuated cataplexy in OX2−/− mice likely results from residual OX1 signaling. Orexin injections into the LDT and LC suppress REM sleep, and knockdown of OX1 in LC increases REM during the dark phase (Chen et al. 2010). Hence, it is plausible that normal OX1-mediated excitation in LDT, LC and DR, reduces cataplexy in OX2−/− mice. However, neither re-expression of OX1 in LC or PPT neurons or OX2 in TMN rescued cataplexy-like arrests in DKO mice (Hasegawa et al. 2014). In contrast, re-expression of OX2 in DR neurons completely rescued cataplexy in DKO mice (Hasegawa et al. 2014). Since signaling by OX2 and OX1 receptors converge onto similar, if not identical, effectors in serotonergic DR neurons (Kohlmeier et al. 2013), these findings strongly support the idea that residual OX1 signaling in DR attenuates cataplexy in OX2−/− mice. Moreover, since OX1−/− mice do not have cataplexy, these findings suggest residual OX2 signaling in DR neurons prevents cataplexy. Thus, orexin mediated DR activity appears to play a key role in coordinating muscle atonia with sleep states and preventing intrusion of muscle atonia into waking.
The previous discussion of orexin signaling in the ascending arousal system has focused on its role in regulating the expression of spontaneous bouts of sleep and waking. Of course, orexin signaling at these loci may also contribute to arousal levels in other circumstances such as during stress, anxiety, panic and/or food and drug seeking (Winskey-Sommerer et al. 2004; Johnson et al. 2012; Heydendael et al. 2013; Steiner et al. 2012; Piccoli et al. 2012; Nair et al. 2008; Boutrel et al. 2005; Harris et al. 2005). Moreover, such signaling likely also support other functions. For example, OX1−/− mice show impaired acquisition and expression of fear conditioning and re-expression of OX1 in TH + LC neurons rescues cued fear conditioning (Soya et al. 2013).
7 Conclusions
While orexins are recognized as excitatory neuropeptides, emerging evidence indicates orexins have actions that go beyond simple excitation, indicating they should be regarded as modulatory peptides that can also produce post-synaptic inhibition. In addition to producing a slow depolarization, orexin produces a set of modulatory actions at ascending arousal system targets. Evidence from whole-cell recordings from constitutive receptor knockout mice and receptor rescue experiments indicate that OX1 signaling in the LC and both OX1 and OX2 receptors in the DR play important roles in consolidating bouts of waking and restricting muscle atonia to REM epochs, respectively. It remains to be determined how the modulatory actions of orexin contribute to the ability of these neuronal groups to organize arousal.
Acknowledgments
This work was supported by grants NS027881 and HL064150 from the NIH of the USPHS
References
Acuna-Goycolea C, van den Pol AN (2009) Neuroendocrine proopiomelanocortin neurons are excited by hypocretin/orexin. J Neurosci 29:1503–1513PubMedCentralCrossRefPubMed
Andrade R, Foehring RC, Tzingounis AV (2012) The calcium-activated slow AHP: cutting through the Gordian knot. Front Cell Neurosci 6:47PubMedCentralCrossRefPubMed
Bayer L, Eggermann E, Saint-Mleux B, Machard D, Jones BE, Muhlethaler M, Serafin M (2002) Selective action of orexin (hypocretin) on nonspecific thalamocortical projection neurons. J Neurosci 22:7835–7839PubMed
Belle MD, Hughes AT, Bechtold DA, Cunningham P, Pierucci M, Burdakov D, Piggins HD (2014) Acute suppressive and long-term phase modulation actions of orexin on the mammalian circadian clock. J Neurosci 34:3607–3621PubMedCentralCrossRefPubMed
Boucetta S, Cissé Y, Mainville L, Morales M, Jones BE (2014) Discharge profiles across the sleep-waking cycle of identified cholinergic, GABAergic, and glutamatergic neurons in the pontomesencephalic tegmentum of the rat. J Neurosci 34:4708–4727PubMedCentralCrossRefPubMed
Bourgin P, Huitron-Resendiz S, Spier AD, Fabre V, Morte B, Criado JR, Sutcliffe JG, Henriksen SJ, de Lecea L (2000) Hypocretin-1 modulates rapid eye movement sleep through activation of locus coeruleus neurons. J Neurosci 20:7760–7765PubMed
Boutrel B, Kenny PJ, Specio SE, Martin-Fardon R, Markou A, Koob GF, de Lecea L (2005) Role for hypocretin in mediating stress-induced reinstatement of cocaine-seeking behavior. Proc Nat Acad Sci USA 102:19168–19173PubMedCentralCrossRefPubMed
Brown RE, Sergeeva O, Eriksson KS, Haas HL (2001) Orexin A excites serotonergic neurons in the dorsal raphe nucleus of the rat. Neuropharmacology 40:457–459CrossRefPubMed
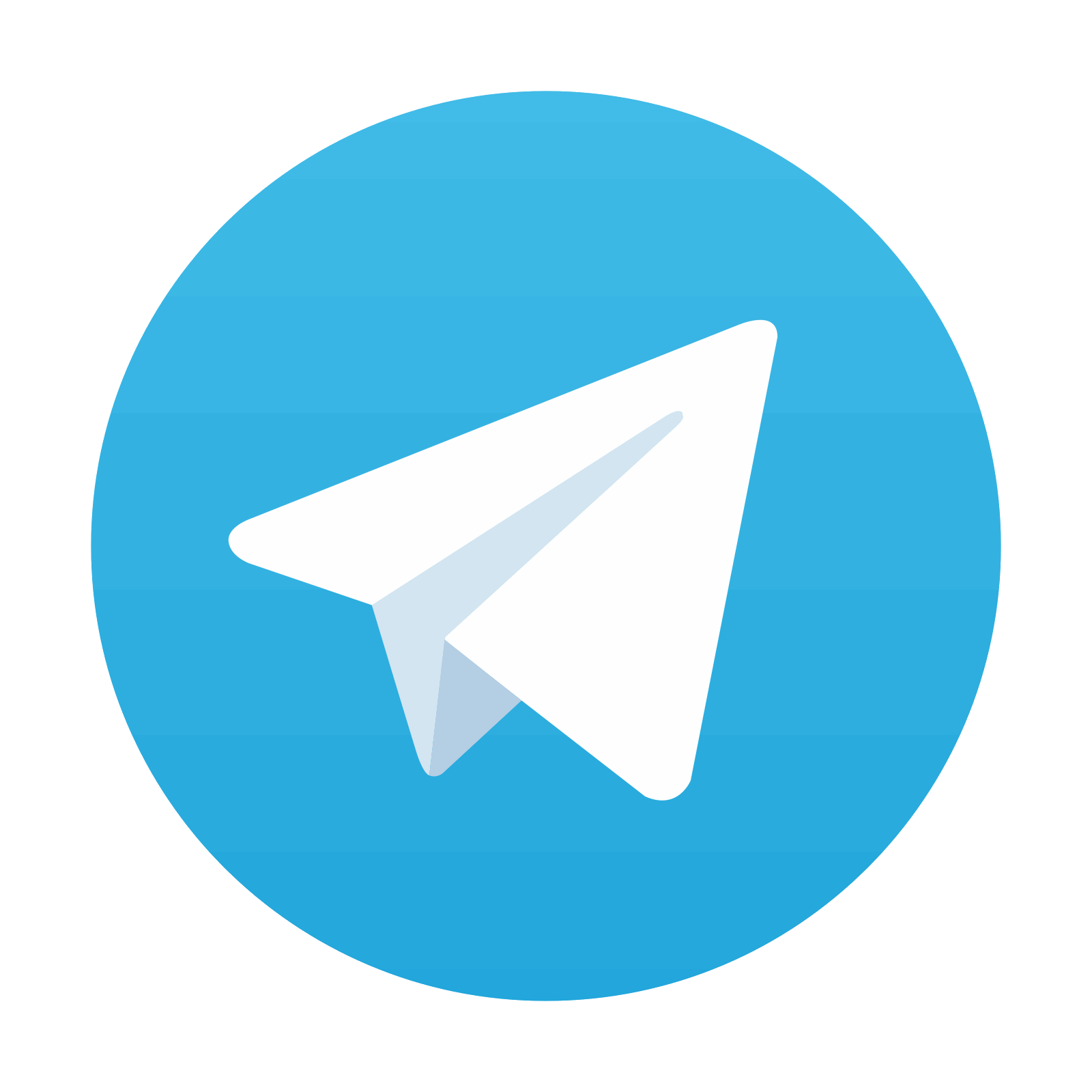
Stay updated, free articles. Join our Telegram channel

Full access? Get Clinical Tree
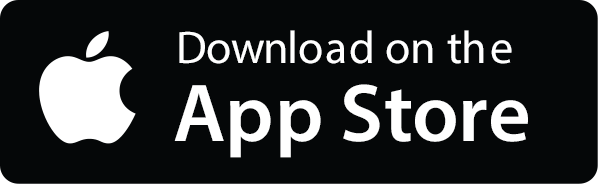
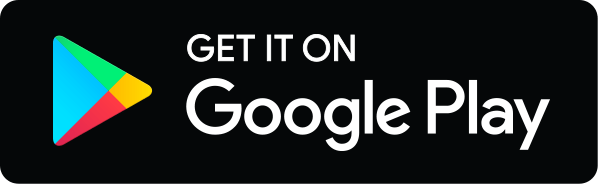