Glutamate neuroreceptor localization and distribution in Central Nervous System. (a) Glutamatergic pathways, (b) hypothetical tracking of glutamatergic neuronal fibers where red-colored fibers represents NMDA receptors, green—AMPA receptors, and blue—kainite receptors
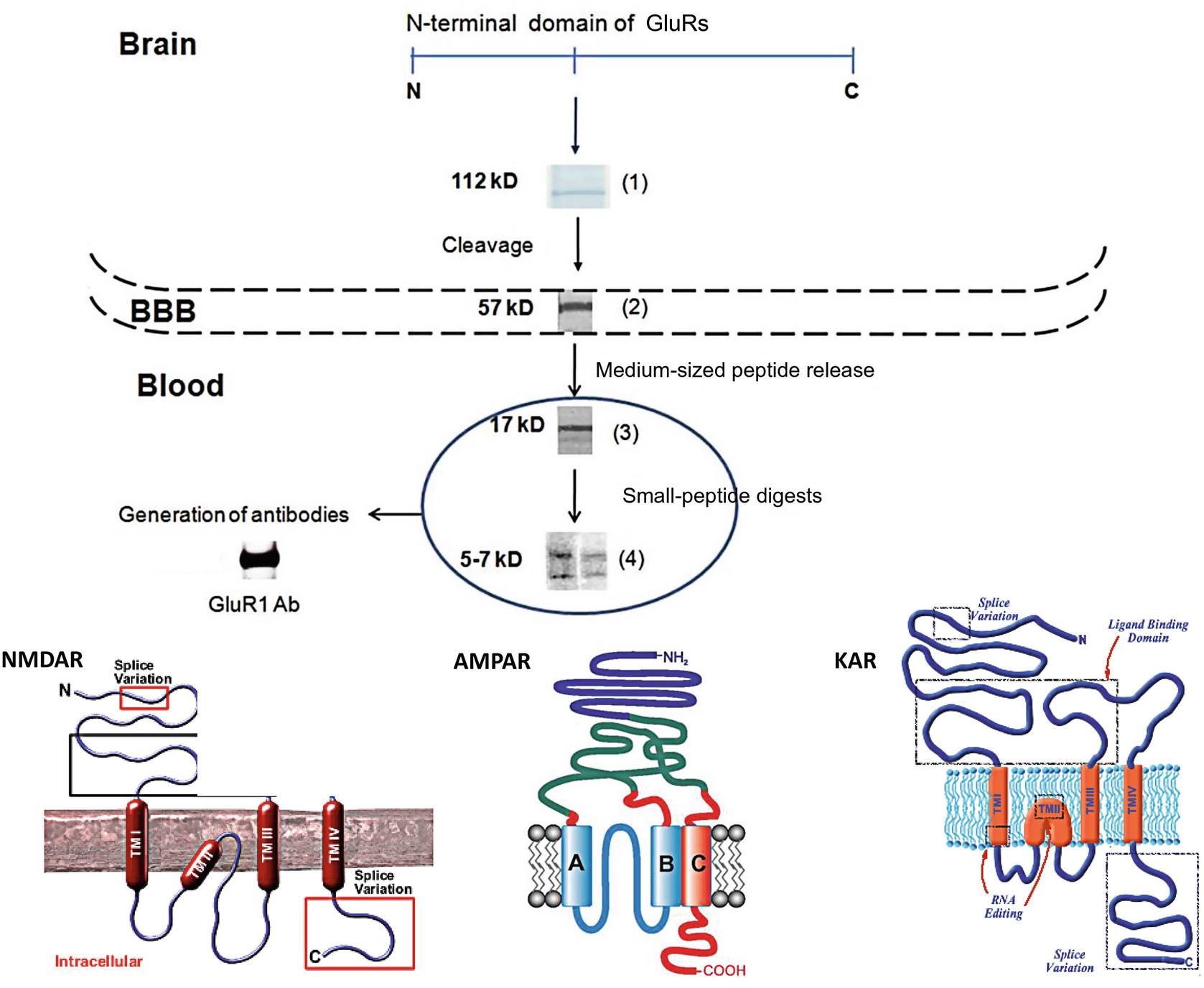
Scheme of GluRs neurodegradomics due to acute stroke
Cerebral blood flow (CBF) autoregulation is controlled by excitatory amino acid receptors including glutamate (GluR) and its subtypes of NMDAR, AMPAR, and kainic acid (KAR) receptors [12, 19]. The dominant role of GluR subtypes in neurotoxicity due to stroke has been demonstrated in acute ischemic stroke [20–22]. Considering the structural distribution of these GluR subtypes in specific levels of the NVU, future translational study design should find a correlation between a panel of related biomarkers, clinical information, and neuroimaging findings including diffusion- and perfusion-weighted imaging.
Molecular diversity of GluR genes encoding various receptor subunits is responsible for the pharmacological and functional heterogeneity associated with predominant subtype locations in the cerebral vascular territory [23]. In addition, the extracellular N-terminal domains of the ionotropic GluRs control diverse receptor functions (i.e., subunit assembly, receptor trafficking, channel gating, agonist potency, and allosteric modulation) [24–26]. Several divergent features of the GluRs also derive from natural tendency of certain subunits to assemble in heteromeric complexes: NR1/NR2A/NR2B [27], GluR1–4 [28], GluK1/GluK5, and GluK2/GluK5 [29, 30].
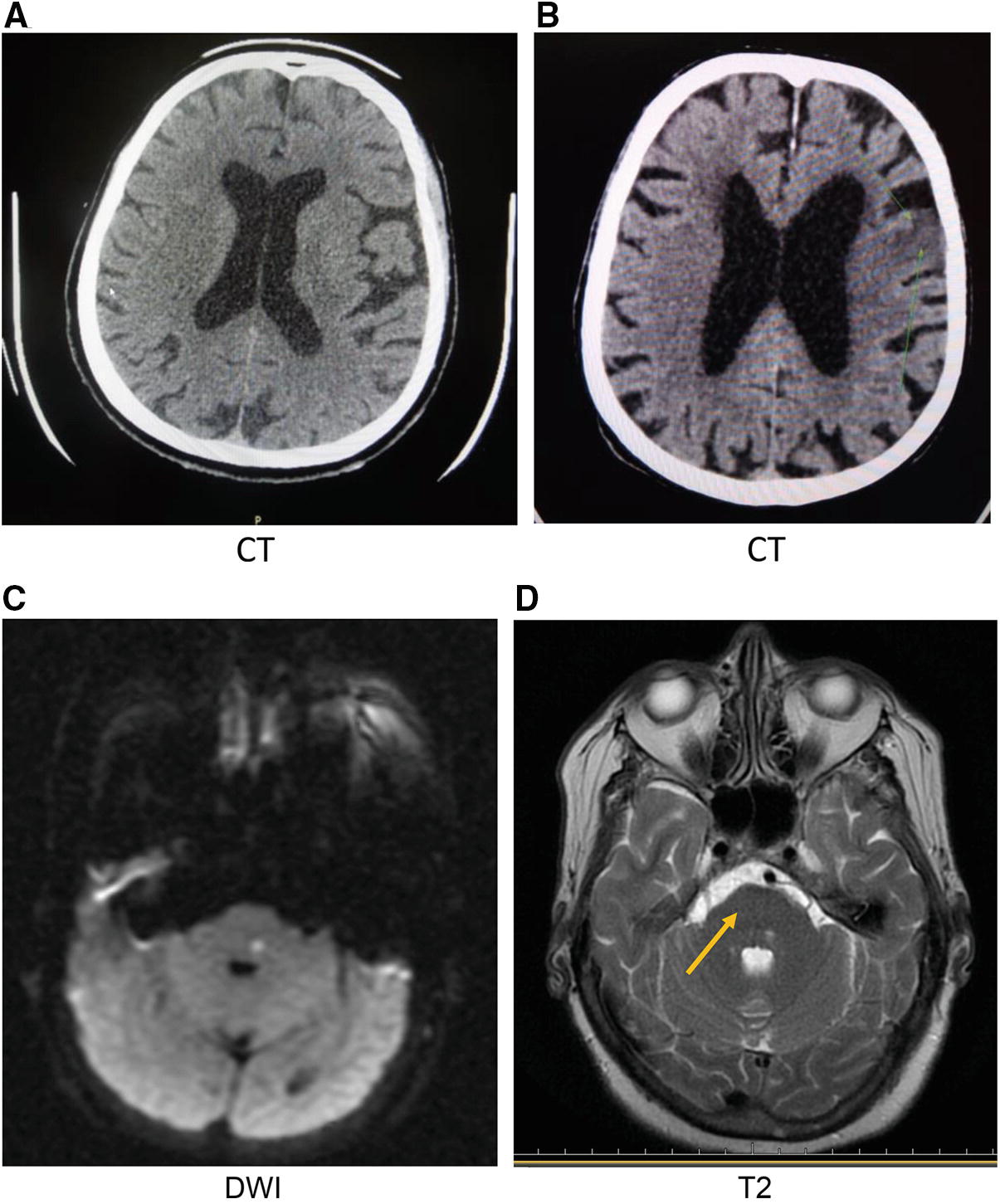
Radiological images of case reports with NR2 peptide detections. (a) CT of acute cardioembolic stroke with diabetes mellitus as preexisting condition, (b) CT of acute atherothrombotic stroke, (c) DWI of acute stroke of unknown origin in vertebral area, and (d) T2-weighted image for the same patient
4 NR2 Peptide as a Biomarker of Cortical Microvessel Occlusion
NR2 subtypes of NMDA receptors are located on the surface of neuroendothelial cells [13, 35]. These subtypes are mostly dependent on glucose metabolism as a source of energy and involved in the regulation of NVU functions predominantly in grey matter [21]. Metabolic alterations of glucose and glutamate amounts in NVU are often associated with risk factors of cortical lesions caused by cardiovascular diseases [36] and diabetes [37].
Preliminary studies of the NR2 peptide as a neurovascular biomarker demonstrated its utility in detecting acute ischemic stroke vs. cerebral hemorrhage and stroke mimics [21, 22]. A significant correlation of NR2 peptide values and new ischemic cortical lesion with volumes below 100 mL was found and associated mostly with cardioembolic stroke [21, 38]. The NR2 peptide also increases during carotid endarterectomy and stenting due to microemboli with following postoperative neurological deficits [39]. Some cases of unknown stroke origin or cryptogenic stroke have extremely elevated NR2 peptide concentrations [40]. Subjects with vascular risk factors such as diabetes and atherosclerosis showed elevated NR2 peptide levels that may indicate cerebral microvessel dysfunction in the areas of NMDAR pathways. Possible brain lesion formation in cortical and subcortical areas might be seen (see Appendix 1).
NR2 peptide assay indicated the capability of recognizing acute ischemic stroke with a sensitivity of 92–98% and specificity of 72–97% at 0.5 and 1.0 ng/mL cutoffs respectively [21]. It was also demonstrated that a statistically significant increase of NR2 peptide occurred in acute IS compared to non-stroke (P < 0.0001). Additionally the NR2 peptide assay notably discriminated stroke mimics from TIA and ischemic stroke. The best cutoff value for TIA diagnosis was 1.0 ng/mL (sensitivity, 83%) at which a positive predictive value of 73% was achieved [22].
Further, the high diagnostic power for the NR2-peptide assay in assessing neurological adverse events (NAE) with a sensitivity of 99% was calculated [22]. It was shown that NR2-peptide concentrations for NAE after carotid endarterectomy and carotid artery stenting (n = 7) were significantly increased preoperatively, intraoperatively, and postoperatively (P < 0.05) compared to those without NAE.
It would appear that measurement of the NR2 peptide for patients with suspected stroke symptoms presented to emergency room (ER) within 24 h of the onset may be beneficial for management decision. Further translational study is needed as to the best use of this data. This neurovascular biomarker may have a potential clinical use for assessment of acute cortical strokes of embolic and thrombotic subtypes in patients suffering cardiovascular disorders or diabetes mellitus as preexisting conditions.
5 AMPAR Peptide as an Indicator of Subcortical Lesions Caused by Small Vessel Occlusion
Biomarkers of subacute stroke, completed stroke, and prior stroke (possibly derived from previously failed acute stroke biomarkers) may find an application in identifying acute stroke cases with large core infarcts.
These cases with large core to penumbra ratios might be at increased risk of hemorrhagic transformation or poor outcome if thrombolysis or revascularization procedures are employed. Alternatively, the more chronic biomarkers may find use in the optimization of secondary stroke prevention treatment strategies or in situations where multiple subclinical small vessel infarctions over time are not amenable to the more modern acute stroke therapies. Optimal acute cerebral vascular biomarkers will correlate with imaging and enhance accuracy of diagnosis, improve prognosis, and help to monitor therapy [4].
It is known that AMPAR subtypes (GluR1, GluR2/3, and GluR4) are primarily distributed in the forebrain and subcortical pathways (hippocampus, amygdala, thalamus, hypothalamus, and brainstem) [41]. GluR1/2 has been shown to be expressed by microglia [42] in close proximity to small blood vessels [43]. It was also demonstrated that the GluR1 subtype of AMPAR was mainly located in dendrites and axons of brain white matter, while GluR2/3 and GluR4 belong to spinal cord white matter [44]. The oxygen-dependent ionotropic AMPA receptor subtypes are expressed at the astrocyte–small vessel interface [14] or white matter microglia [15, 17] vulnerable to excitotoxic events following acute stroke.
Due to co-localization of NMDAR and AMPAR in certain subcortical areas it would be plausible to detect not only increased NR2 but also AMPAR peptides. Considering prior investigations [45, 46], the detection of AMPAR peptide would be the most effective for assessment of lacunar lesions and hemorrhagic transformations particularly in deep subcortical structures of hindbrain.
Hypertension and hypoxia may affect arterial microvessel velocity and contractility as well as causing significant dysfunction—vasospasm in small arteries of subcortical structures [47, 48]. Increased microvascular tone is a compensatory mechanism against hypertension enhancing autoregulation and redistribution of blood flow and pressure from major arteries at the base of the brain to small resistant vessels [23]. These small vessels might be directly damaged by high pressure that can induce lacunar lesions or microbleedings in subcortical areas [49]. Additionally, hypoxia or multiple subtle brain injuries may be associated with subcortical microbleeding (Appendix 2), and the subsequent acute inflammation causing focal white matter hyperintensities [32].
Abnormally elevated AMPAR peptide values were observed in patients with uncontrolled chronic hypertension (P < 0.01) and prior multiple concussions. White matter fiber tracts were often absent in frontal and parietal areas of brain of concussed subjects [32]. These individuals showed hypoperfusion abnormalities in subcortical and basal ganglia regions on MRI accompanied by microscopic hemorrhage and lacunar infarctions (unpublished data).
It is possible that AMPAR peptide detection in suspected acute stroke would aid in assessment of white matter lesions (lacunar stroke) caused by small vessel occlusion as well as hemorrhagic transformations. Simultaneous measurements of AMPAR and NR2 peptides might help in assessment of possible location of subcortical lesions prior to CT/MRI and could reflect the severity of stroke.
6 Panel of GluR Peptide Biomarkers for Assessment of Acute Stroke Severity
The diagnosis of acute stroke is currently largely based on clinical assessment and non-contrast head CT [50]. However, CT is insensitive to early ischemic changes [51, 52]. Although advanced neuroimaging, such as diffusion-weighted MRI (DW-MRI) or perfusion head CT, are more sensitive to detect early ischemia, they are not readily available in most hospitals [52–54]. In addition, MRI is expensive and may be contraindicated in a significant number of patients due to metal implants, medical instability, obesity, and/or severe claustrophobia [53]. Similarly, patients with renal impairment or contrast allergies cannot undergo perfusion CT. In these cases, diagnosis and treatment rest on clinical symptoms that introduce the risk of inappropriate use of thrombolytic therapy, adverse outcome, and increased treatment cost.
Preexisting conditions like atherosclerosis and cardioembolic conditions might produce large infarcts in gray and white matter [8], while lacunar lesions of different etiology are found in subcortical areas and deep white matter [55]. Diabetes is another risk factor that could produce cortical lesions due to metabolic alterations in neurovascular unit. Ischemic lesions in cryptogenic strokes are often found in deep subcortical and brainstem vascular territories [56]. To understand the disease origin, it would be plausible to add specific blood assay(s) to clinical and radiological examinations.
A rapid panel of GluR peptide assays [57] might help to assess the severity of cortical/subcortical infarctions particularly at risk of hemorrhagic transformations [58]. The progressing acute ischemic stroke is difficult to predict at ER presentation. It was estimated about 30% patients would recover, up to 6% will have unfavorable outcome, while approximately 46–50% might have unpredictable outcome [59]. One of the scenarios could result in symptoms worsening because of microlesions might expand to deeper subcortical structures and/or merge forming larger volume lesions.
It was reported that up to 20% of patients presenting with stroke-like symptoms have no cerebral ischemia but present with the so-called “stroke mimics,” including complicated migraine, postictal paresis, psychological disturbance and other etiologies [60]. These patients should not receive thrombolytics. If a biomarker assay(s) could accurately differentiate stroke mimics from acute IS and TIA, potentially dangerous treatment with thrombolytics could be avoided. Furthermore, costs due to unnecessary hospital admissions, extensive neuroimaging and diagnostic studies could theoretically be reduced.
The selection of potential neurovascular biomarkers based on structural distribution of glutamate receptors family (NMDAR and AMPAR) in certain neurovascular territories is explored (Appendixes 1 and 2). Results of GluR peptide assays in acute IS support the premise that preexisting conditions affect the acute cerebral infarction development. Indeed, diabetic and cardioembolic conditions showed a tendency to worsen an acute cortical infarction that accompanied drastically increased NR2 peptide values. Hypertension and prior concussions/mild TBI could sequentially trigger subcortical (lacunar ) strokes or hemorrhagic transformations that correlated with elevated AMPAR peptide levels in peripheral fluids. Multiple preexisting conditions might affect the severity of acute ischemic stroke that could be more accurately assessed by a panel of GluR peptide biomarkers [61].
The combined use of neuroimaging and a rapid panel of GluR peptide assays might improve diagnostic certainty of cortical/subcortical infarctions at ER. Additionally, the rapid assay panel could speed up patients’ stratification that could significantly improve stroke outcomes and increase efficiency of ambulance services and stroke center management.
7 Methods
7.1 Translational Study Protocol
A translational study protocol to assess the utility of GluR peptide assays for acute stroke is considered. The protocol could be used as a template for various design scenarios of clinical studies when the project involves any physical contact or medical interventions with participants. It would be the best approach if the investigation is planned to be a multicenter, prospective, and blinded clinical study.
The initial requirements including purpose, objectives, hypothesis, specific aims, and background information for selected neurovascular biomarkers should be addressed in the research protocol.
7.1.1 Objectives
To investigate diagnostic values of GluR (NR2, AMPAR, KAR) peptides for assessment of acute ischemic events in ER within 12 h after the onset. This study intended to analyze diagnostic accuracy of each biomarker depending on the level of arterial/venous network involved (capillary, small vessels, arterial, or venous circulation) in the formation of cerebral lesions defined by MRI. The secondary objective is to examine the possibility to differentiate the severity of acute ischemic stroke using the biomarkers related to preexisting conditions.
7.1.2 Aims
- 1.
Examine GluR peptides levels in a healthy population and subjects with preexisting conditions.
- 2.
Examine changes in GluR peptide levels that occur in patients presented to ER with symptoms suggestive of acute stroke (stroke vs. non-stroke).
- 3.
Examine GluR peptide values and diagnostic utility in patients with acute ischemic stroke relative to standard stroke protocol (USA).
- 4.
Examine GluR peptide levels and their relationship to MRI and neuroimaging modalities.
Based on proposed Aims the following hypotheses should be tested.
7.1.3 Hypotheses
- 1.
GluR peptides values will not be elevated in healthy volunteers.
- 2.
GluR peptides levels will show greater increase in acute ischemic stroke than acute cerebral hemorrhage and stroke mimics.
- 3.
GluR peptides amounts will correlate and demonstrate at least similar diagnostic utility compared to standard stroke workup.
- 4.
GluR peptides levels will triage acute IS patients for MRI.
The relevant prior experience and gaps in current knowledge should be addressed showing how the biomedical study will add to current information. The background evaluation should delineate relevant preliminary data and references for each biomarker.
7.2 Inclusion and Exclusion Criteria
As a general approach the correct study cohort should selected based on (1) disorder subtype, (2) preexisting, and (3) “mimic” conditions or concomitant diseases, (4) race, (5) age, and (6) gender. Then control group should include not only age-, race-, and gender-matched healthy volunteers but contain additional group of persons with preexisting conditions (atherosclerosis, diabetes, and hypertension) and stroke mimics (migraine, palsies, and postictal paresis).
It is known the stroke causes lesion(s) in certain brain vascular territory(ies) where the definite subtype of GluR would be preferably located. Then the study groups should be considered from lesion location points (cortical or subcortical and vertebrae), stroke subtype (cardioembolic, thrombotic, cryptogenic). It is required the data from MRI diffusion and diffusion tensor modalities will be acquired to assess hyperintensities in cortical and subcortical areas due to acute stroke (please see Chapter 13).
Additionally, it is necessary indicate (1) the time since onset when sample drawn, (2) prior medications used (cross-reaction or factor diminishing biomarker levels), and (3) type of sample (plasma or serum) to assess the peptide or protein.
The information about “critical values” of the test is needed for the blood test to speed up the diagnosis of acute ischemic stroke prior to CT imaging—for example, at ER (see Appendix 3). Critical values are test results that fall significantly outside the normal range and may represent life-threatening values even if obtained from routine tests (e.g., “panic values” or “red-line values”) and must be conveyed immediately to the physician or other health care professional so that therapeutic measures can be instituted rapidly.
The following eligibility criteria are designed to select subjects for whom participation in the study is considered appropriate. All relevant medical and nonmedical conditions should be taken into consideration when deciding whether a subject is suitable for the study. Subject eligibility should be reviewed and documented by an appropriate member of the investigator’s study team before being included in the study. The enrollment starts after informed consent is obtained and all inclusion/exclusion criteria met.
7.2.1 Study Population
The study will consist of patients with acute stroke-like symptoms (including cerebral hemorrhage and stroke mimics) and/or suspected cerebral infarction (not necessary first) according to Cincinnati prehospital stroke scale (Cincinnati code) admitted through emergency medical services (EMS) [62].
Control group will include age- and gender-matched healthy volunteers and subjects with preexisting conditions.
7.2.2 Inclusion Criteria
Patient or legal representative has read, understood, signed, and dated the Informed Consent prior to initiation of the study procedures.
Male or female patients 21 years of age or older. There is no upper maximum age.
Patient or legal representative must present or report with ongoing and/or a history of neurologic symptoms suggestive of stroke within 12 h of symptom onset with and without history of preexisting conditions (atherosclerosis, diabetes, and cardiovascular disease).
A blood sample must be collected within 12 h of symptom onset.
7.2.3 Exclusion Criteria
If excluding vulnerable populations (pregnant women, illiterate or non-English speaking individuals) a scientific rationale for the exclusion should be provided. Inconvenience or cost is not an acceptable rationale.
Presentation outside the 12-h time window of symptom onset or if the onset time cannot be identified as likely within this time frame.
Patients with traumatic brain injury.
Patients who have a contraindication to having a brain MRI (claustrophobia, pacemaker, defibrillator, neurostimulator, a metal implant of any kind or any metallic foreign bodies such as bullets, shrapnel, metal slivers).
Pregnant women will be excluded from testing which involves the use of MRI and CT scans specific to this protocol.
Patients with a history of severe psychiatric illness.
Patients with a history of alcohol or substance abuse.
Patients who may be unable or unlikely to complete the study.
Currently enrolled in another investigational study or participated in an investigational study in the 30 days prior to the present study start.
Patient has received rt-PA treatment after current admission to the hospital and before blood drawn for the peptide assays.
7.2.4 Number of Research Participants
The study will recruit 800 participants and proceed in two phases. The intent of the first phase is to estimate optimal cutoffs for each time window from which the blood sample for the GluRs peptides levels quantification is drawn (i.e., 0–6, 6–12 h) for discriminating ischemic vs. stroke mimics and cerebral hemorrhage. The intent of the second phase is to validate each peptide as a diagnostic test using the cutoff values identified in the first phase. According to sample size calculation there should be n = 100 patients per group and time window (Appendix 4) to be enrolled in at least 3–4 Centers of Excellence for Stroke ERs to complete the study within 2 years.
Practical experience shows that written informed consent usually could not be obtained from about 30% to 50% enrolled patients due to ER conditions. Therefore, the above cited number of study participant should be increased by 50% from initial enrollment. Consider the use of electronic database where the recruitment at each participating side should be reflected in numbers per day. Additionally, Cincinnati code sensitivity ranges from 44% to 95% and specificity showed a wide bracket (23–96%) due to the scale reproducibility variations between EMS teams in the same region of country [62]. This code is designed to assess large vessel occlusions and does not detect up to 38% of posterior cerebral circulation strokes. The use of Cincinnati scale provides about 57.6% correctly recognized cases of acute stroke [63] with about 25% of patients arriving at a hospital within 3.5 h, and less than 65% arriving within 8 h of symptom onset [64].
7.3 Recruitment Methods
Patient enrollment starts when all training of study personnel and certification requirements are met. Staff are trained on how to effectively administer informed consent and provide adequate information for each potential participant as well as the procedures for the study. A standardized consent form should contain a clear and concise study description in language at an acceptable reading level and will be customized with center-specific information. All key personnel should be required to provide proof of Human Subjects Education prior to beginning of screening. A properly constituted, valid Institutional Review Board (IRB) must review and approve the protocol, the investigator’s informed consent document, any subsequent changes to the protocol, and related patient information and recruitment materials before the start of the study. It is the responsibility of the principal investigators at each site to ensure that written informed consent is obtained from the patient or the legal representative before any activity or procedure is undertaken that is not part of routine care and that each participant receives a copy of the signed consent.
Enrollment starts after informed consent is obtained and ends after the site diagnosis has been entered into the electronic standardized Case Report Form (CRF) following the diagnostic workup. This process typically occurs within 24–72 h of study enrollment but in single cases may take a longer time. Group enrollment will be closed as soon as the maximum number of patients has been accrued for each group and time window. There is no randomization. Different diagnostic categories (IS, intracerebral hemorrhage, stroke mimic, or control) and time points from symptom onset will be accrued by group quota.
7.4 Study Design, Procedures and Timeline
Study design and related research procedures to be performed should be described. Study safety or minimizing risk monitoring should be included. The major procedures are intended to perform: neurological, radiological assessment of patients and blood sample withdrawal on admission to ER.
7.4.1 Neurological Assessments
Schedule of events for symptomatic patients
Procedure | ED presentation within 12 h of the onset |
---|---|
Informed consent | × |
Inclusion/exclusion criteria | × |
Demographics and medical history | × |
Current medication usage | × |
Clinical laboratory testsa | × |
NIH Stroke Scale | × |
TOAST classification | × |
Stroke symptom questionnaireb | × |
Performed imagingc | × |
Blood draws: | |
GluR peptide assays (plasma) | × |
Patient’s final diagnosisd | × |
Study related adverse events (AE) | × |
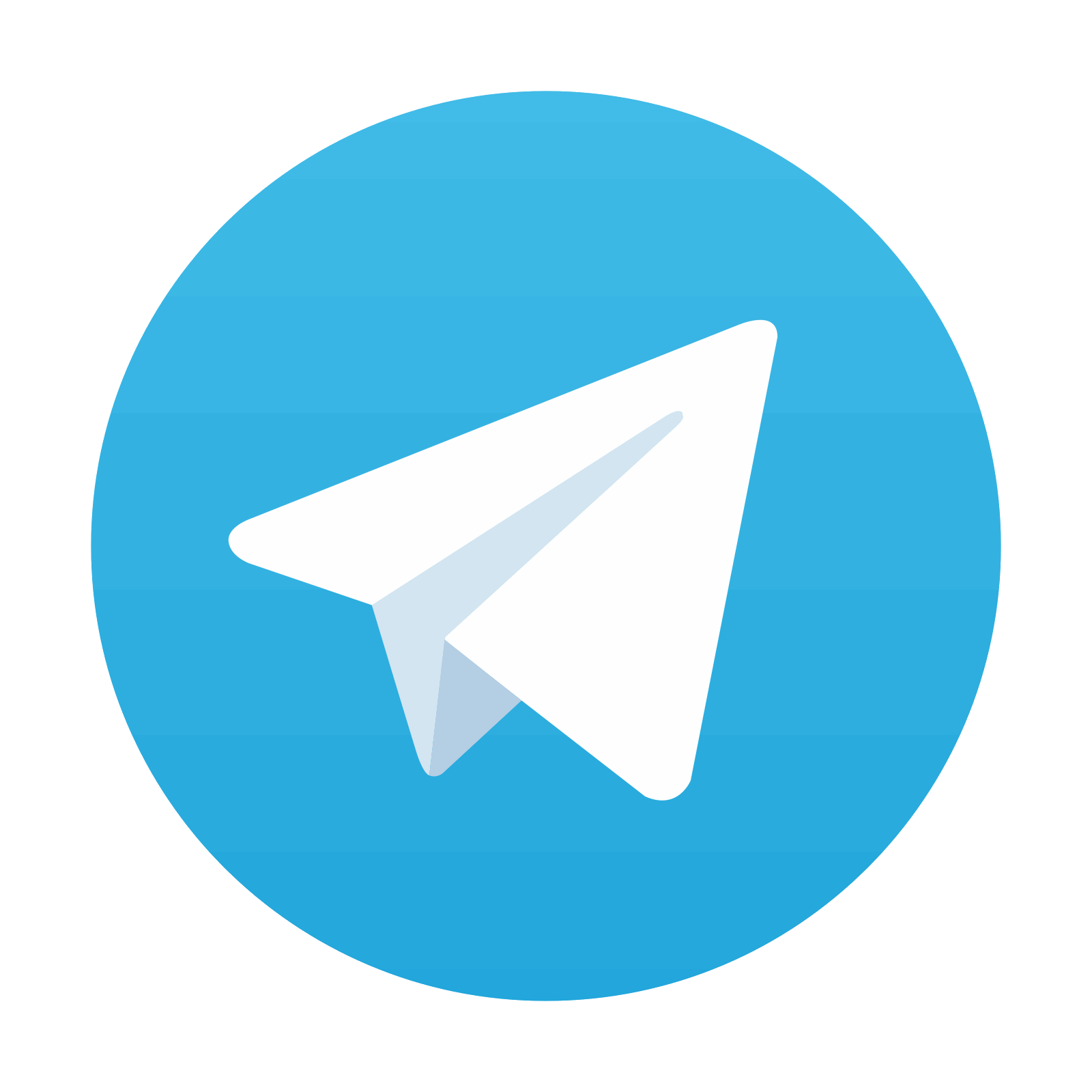
Stay updated, free articles. Join our Telegram channel

Full access? Get Clinical Tree
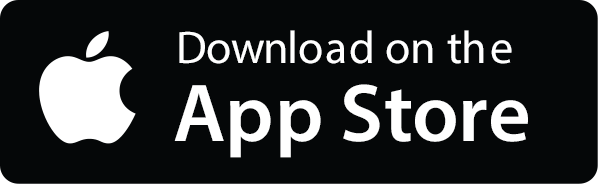
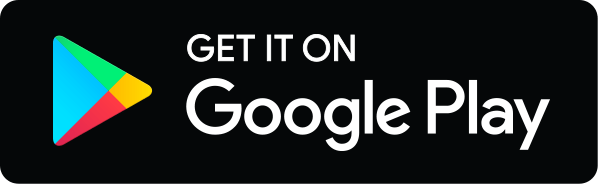