(1)
Department of Neurosurgery, St Elisabeth-Tweesteden Hospital, Tilburg, The Netherlands
It has been known for ages that some form of spontaneous recovery usually follows a disabling injury. In the past, this was generally attributed to ‘the healing power of nature’ (Hippocrates’ vis naturae medicatrix) or to supernatural forces [1]. Scientific inquires have revealed the nature of many of these recovery processes, and we have learned, to some extent at least, to understand and influence the course of injury and disease. In the case of a fractured bone, for example, physical and physiological processes have been elucidated quite accurately, and several treatments have been developed that facilitate healing of the fracture and recovery of a person’s functionality. But clearly, in many other cases, in particular when there is damage to the brain, restoration of function is often incomplete or insufficient, and we are failing to grasp all the relevant factors that are involved in the process [2, 3]. This chapter reviews neural plasticity from a clinical point of view and specifically focuses on the brain’s potential to reorganize the neural circuitry for language functions at the macroscopical level (i.e. in terms of brain areas and white matter pathways).
9.1 Historical Perspective on Restoration of Function
9.1.1 Momentum
Clinicians know from experience that lesions with an acute onset, for instance, due to trauma or stroke, produce far more symptoms than those that develop more slowly, such as brain tumours. This was already described more than two centuries ago by Morgagni (1761) and Hall (1841). Hall noted that slowly growing brain tumours may even exist without any symptoms [4]. A few decades later, Hughlings Jackson used the term ‘momentum’ to refer to the combined impact of the size and the rate of growth of lesions to understand the patient’s symptomatology (i.e. mass × velocity). He disagreed with others, such as Flourens, who mainly considered the size of the lesion important and who believed in an almost complete ‘equipotentiality’ of the brain. His convictions later found support in experimental studies in animals (in the 1950–1960s), which showed that successive but partial surgical lesions generally produce far less disability than one large ablation [1, 5, 6]. A comparable multistage surgical approach has in recent times been advocated for brain tumour patients, whereby a remnant was deliberately left during a first operation (because of tumour ingrowth in brain areas that are still important for motor or language functions). When these patients were reoperated on several years later, the extent of resection could often be significantly increased with minimal morbidity [7, 8]. Apparently, further slow growth of the tumour remnant, possibly in combination with the effects of surgery, allowed further functional reshaping.
Scientific studies have confirmed what clinicians already learned a long time ago, namely, that the brain can in many cases compensate for the disabling effects of a lesion and that time is an important factor in this process. Stroke and brain tumour patients, when matched for the size and location of their lesion, clearly have different patterns of neuropsychological impairments (e.g. Anderson 1990) [9]. Tumour patients are generally least affected and may even perform completely normal on neuropsychological tests. These findings obviously confound any of the straightforward relationships between brain structure and function that were drawn in the past. Head, in 1926, was among those who were already well aware of this more complex situation. In his standard work on aphasia, he wrote:
in all attempts to correlate the site of structural changes with defects of function it must never be forgotten that the severity and acuteness of the lesion exert an overwhelming effect on the manifestations.
Thus a complete act of speech comes to be a wide-spread response of the organism to each fresh situation; this employs conscious, subconscious, automatic and purely physiological processes. The deeper the lesion the grosser and more definite the disorder. On the other hand, since the cortex is a more flexible organ with less rigid and preordained reactions, the disturbance of function due to injury of the surface is not so severe and permanent and is less easily determined [10].
9.1.2 Age
In addition to the spatial distribution and growth rate of a brain lesion, there is another variable that significantly influences functional outcome of the patient: age at the time of the brain insult. This phenomenon was also well recognized a long time ago. In 1868, just a few years after Broca’s landmark publications, Cotard published a study where he analysed the autopsy reports of 42 patients [11]. He found damage to the left frontal lobe or even the entire hemisphere in seven patients with a right-sided hemiplegia since childhood, but with normal language and intellectual functions. Cotard thus demonstrated that left hemisphere damage that is acquired in early life does not necessarily lead to lasting language deficits. Steffen, in 1885, documented a complete recovery in 18 of 25 patients with acquired childhood aphasia and reported a good outcome in most of these cases [12]. It appeared that recovery in children was better and quicker than in older patients with similar deficits [13]. These authors, along with others such as Broca (1865), Clarus (1874), Wernicke (1874) and Bernhardt (1885), proposed that the right hemisphere could support language functions in the case of severe left hemisphere damage [12, 13].
More than a century later, these initial findings were confirmed in patients where a lesioned hemisphere was surgically removed [14]. In 1962, Basser reported on a series of 34 children where a hemispherectomy was performed because of intractable epileptic seizures. All but one patient developed normal language functions, and Basser concluded that for development of speech, ‘the left and right hemisphere are equipotential’ [15]. A similar conclusion was drawn by Wilson (1970) who found in his series of 50 patients that the side of the hemispherectomy did not influence subsequent language development [16]. These observations, according to Bates and Roe:
led Lenneberg (1967) to his controversial notion that the brain is ‘equi-potential’ at birth, with lateralization determined gradually across the course of development. As a corollary, Lenneberg also argued that this period of equipotentiality and plasticity is brought to an end at puberty, providing the first systematic argument in favor of a ‘critical period’ for language [11].
To date, it remains unclear to what extent the left hemisphere is the default site for the ‘organ of language’ and what eventually determines language lateralization and language abilities [11, 17, 18]. Atypical (right hemisphere) language representation has been associated with developmental disorders, but is also present in the healthy population [19]. Laterality seems determined by multiple factors that are both environmental (acquired brain damage; exposure to language) and genetic (structural brain asymmetries; innate language abilities; gender) [19–21]. According to Bates and Roe, one of the most puzzling results in children with brain lesions is that ‘most studies fail to find any significant difference in language outcomes when direct comparisons are made between children with left- vs. right hemisphere damage’ (see also Chap. 6, Fig. 6.25) [11, 22]. These observations fit a model whereby language functions gradually develop from a bilateral to a more unilateral representation. There is also some evidence that language functions become more focally represented when infants improve their language skills. According to Bishop (2013), ‘language-impaired children with poor phonological skills have more diffuse and bilateral processing of speech sounds than typically developing children’ [19]. Other observations, whereby the type of aphasic disorders is age-specific, are also consistent with such theories:
When Brown and Jaffe (1975) reviewed the clinical aphasia material, they noted an age specificity of certain aphasic disorders. For example, a lesion in Wernicke’s area will produce motor aphasia in a child, conduction aphasia in middle age, and jargon aphasia in late life. This suggests a progressive differentiation or regional specification within the dominant hemisphere language zone. (…) In this process, expression may lateralize earlier than comprehension. The more diffuse representation of production mechanisms in younger patients accounts for the occurrence of nonfluent aphasia with more widely distributed lesions. The relative preservation of comprehension in these patients may reflect the contribution of the right hemisphere or intact portions of the left [23].
9.1.3 Experimental Studies in Animals
In the nineteenth century, experimental studies began to investigate the brain’s potential to compensate for damage. In 1824, Flourens described recovery of function after surgical damage to parts of the brains of birds and mammals. As was described in Chap. 1, these studies were rather primitive and crude [24]. Fifty years later, in the era of Fritsch and Hitzig and Ferrier, the precision and reliability of experimental studies had significantly improved (see Chap. 6). In 1876, for instance, Soltmann studied the cortex of dogs and rabbits, which he believed to play an important role in willed movements [25]. He made lesions to cortical motor regions and found remarkable functional preservation, in particular in younger animals:
In one puppy, who could walk normally following such a lesion, he showed that [electrical] stimulation of the undamaged cortex elicited bilateral movements. This was not seen in dogs lesioned as adults. He postulated that the undamaged motor cortex had taken over the function of the damaged cortex [26].
Around the turn of the nineteenth century, several studies had demonstrated that surgical lesions to the motor cortex of monkeys frequently resulted in very significant recovery of function, even when contralesional motor areas were subsequently removed in a second or third surgical session. In the same year that Leyton and Sherrington published their landmark paper on the electrically excitable motor cortex of apes and monkeys (1917), the potential importance of postlesional rehabilitation was indicated in a study by Ogden and Franz [27]. In their experiments a large part of the motor cortex in monkeys was lesioned, resulting—as expected—in a flaccid paralysis of the contralateral limbs (Fig. 9.1). In some of the animals, they constrained the contralesional upper limb immediately after surgery and also instituted daily movement therapy for the affected upper and lower limbs. What they found was that these animals regained, in their view, full functionality of their limbs, whereby much of this recovery was seen in the first 2–3 weeks. What was even more remarkable was that the animals who did not receive the limb constrainment and movement therapy remained greatly impaired. These, and several other studies, highlighted the enormous potential of the nervous system for recovery, even after it had suffered extensive damage. However, these results seemed largely forgotten for many decades, until constrained-induced movement therapy was reintroduced for hemiplegic stroke patients by Edward Taub (1931) [28]. Taub’s work, which originated in the 1960s, was predominantly based on surgically induced spinal cord lesions that left the monkeys’ limbs deafferentiated. His idea was that a monkey, or for that matter a human, did not use a paretic extremity because it had not learned to reuse it properly immediately after the injury. Under normal circumstances, an animal gets positive feedback from successful limb movements. Taub hypothesized that under pathological conditions, when movements cannot be adequately executed, this reinforcement becomes impaired. As a result, the animal gives up and starts to use other limbs to compensate for the behavioural deficit (‘learned nonuse’). This will lead to deterioration of the motor programmes of the affected limb, further diminishing the chances of functional recovery:


Fig. 9.1
Top view on a schematic drawing of the left hemisphere. Horizontal lines indicate the classic lesion site in the left precentral motor cortex of monkeys in the study of Ogden and Franz (1917) [27]
Taub believed that even after stroke, there was good chance that the motor programs for movement were present in the nervous system. Thus the way to unmask motor capacity was to do to human beings what he did to monkeys: constrain the use of the good limb and force the affected one to begin moving.1
Patient studies with ‘constraint-induced movement therapy’ were successful and demonstrated relevant improvements in the use of the affected arm [30]. Today, the therapy is widely used in the rehabilitation of stroke patients.
In the period 1980–1990, experiments that used neuronal microrecordings in monkeys confirmed that cortical maps were plastic, in the sense that they could increase or decrease in size when afferent input was modified (see for a review Kaas 1991) [31]. For instance, when a digit is amputated or a peripheral nerve transected, the cortical region for skin sensation becomes ‘invaded’ by neighbouring regions whose sensory functions have remained intact [32]. Effectively, the area is now involved in these new functions, as was demonstrated by Michael Merzenich (1942) and colleagues in a series of famous experiments [32, 33]. His group showed that within 2 months after amputation of a digit, the corresponding cortical area begins to respond to sensory stimuli of the adjacent fingers. Initially, it was estimated from these experiments that the expansion of neighbouring cortical representations did not extend beyond a distance of 1–2 mm. Subsequent experiments have suggested that the upper limit of cortical reorganization was at least a magnitude larger (i.e. up to 1 cm) [34]. Evidence for local adaptation of cortical maps has meanwhile been demonstrated for other modalities (auditory, motor, visual) in many other studies and also in humans (among others, in braille readers and in patients with phantom limb experiences) [35–38].
Even during ontogenesis, cortical specialization seems predominantly dependent on the type of afferent input that a particular area receives and not so much on the genetic predisposition. The location of an area therefore does not necessarily determine its functions. When retinal input is surgically fed into the auditory pathways of young ferrets, for example, the temporal cortex (i.e. the classic location of primary auditory cortex) will take on a systematic representation of visual space [39]. And if cortical areas are surgically relocated in young animals, functionality will change accordingly. O’Leary and Stanfield (1989) transplanted parts of the foetal cortex from one sensory domain to another (e.g. from sensorimotor to visual areas) and found that these will now take on the function that is appropriate to the input they receive at their new location [40]. Following similar rules, newborn kittens become cortically blind if one eye is deprived from normal visual experience (e.g. by suturing one eye closed). Without the appropriate visual input, this part of the visual cortex will not develop normally, and after a certain critical period, the anatomical and physiological changes become irreversible. Most cells will now have lost the ability to respond to the eye that was closed. If similar experiments are performed on adult cats, these deprivation-derived changes do not occur [41].
9.1.4 General Observations on Brain-Injured Patients
The idea that changes in behaviour are somehow anchored in brain structures originated in the ideas of James, Cajal and Hughlings Jackson, well over a century ago. Hughlings Jackson reasoned that if representations in the brain were static, then the degree to which undamaged areas could compensate would also have to be fixed. This, however, conflicted with his many observations of recovery after a focal brain lesion and led him to formulate his Principle of Compensation: functions had a dynamic representation in the brain [42]. At the end of the nineteenth century, there were several other investigators that argued against the classic and static localistic theories that dominated that era. Friedrich Goltz (1834–1902) and Charles-Édouard Brown-Sequard (1817–1894), for instance, wrote about the non-local effects that brain lesions had on the remaining healthy parts of the brain, thereby raising questions about strict localization of functions [43, 44]. Although not the first, Constantin von Monakow (1853–1930) would become the proponent of these distant-effect theories and introduced the concept of diaschisis [43]. A similar concept would decades later be formulated by Geschwind, who referred to it as a disconnection syndrome. As we have seen in Chap. 7, Geschwind’s ideas relate to those of Wernicke and his school of associationism. Today, the concept of diaschisis seems again at the heart of our understanding of brain functions and has clearly resurfaced along with new MRI techniques that are able to visualize and quantify connectivity within the brain. A good overview is provided in the paper by Carrera and Tonini (2014) [44].
von Monakow observed that the functional impairments that resulted from brain lesions were often out of proportion to the amount of brain tissue that was damaged or were better explained by a dysfunction of remote brain areas. To him, the sole location of a lesion was not a very reliable indicator of its function, except maybe for elementary sensory and motor functions. This led him to propose that any lesion in the brain causes a functional standstill (German: Stillstand) of distant areas due to a disruption of anatomical connections (Fig. 9.2). This inactivation was to a certain extent a temporary process, so he hypothesized, explaining the well-known observations, that brain damage is commonly followed by functional recovery. von Monakow did not favour the idea that new brain areas compensated for the loss of functionality. Instead, he argued that the ‘inhibition’ of uninjured areas gradually resolved and that this led the patient to regain part of his functionality [45]:


Fig. 9.2
von Monakow’s illustration (1914) of the remote effects of a focal lesion (German: Herd) in the right hemisphere. Shown are fibres of the corticospinal tract (A) that are descending to the spinal cord and cause local dysfunction. Diaschisis (i.e. ‘suspension of activity’ via collateral and associative fibres) causes dysfunction in several remote ipsilateral and contralateral hemispheric areas (B–D). von Monakow often observed recovery of function after focal lesions, a process that could already begin in the first hours after lesion onset and would usually continue in the following days and weeks. He attributed recovery to (partial) resolution of the diaschisis (Figure from von Monakow’s book Die Lokalisation im Grosshirn und der Abbau der Funktion durch Kortikale Herde (1914) [44])
The key-note of his [von Monakow’s] position is given by the universally recognized fact that the focal symptoms, which immediately follow a non-progressive lesion, are severer, more extensive and often less sharply determined than is the case after expiration of some days or weeks. Moreover, these initial manifestations may differ profoundly in character from the residual consequences of irreparable anatomical destruction. Thus, an operation upon the cortex and sub-cortical tissues may be immediately followed by a total flaccid hemiplegia accompanied by loss of sensation so gross that it would appear to be the result of a mid-brain lesion. After many weeks to months these temporary signs pass away and the clinical phenomena come to correspond more nearly in form to those consonant with injury to higher cerebral centers.2
von Monakow’s ideas resulted from his many years of clinical, anatomical and experimental studies. He eventually became head of his own research institute in Zurich (Hirnanatomisches Institut) and famously described several anatomical structures that were later given his name. The lateral cuneate nucleus and the rubrospinal fasciculus are nowadays known as von Monakow’s nucleus and von Monakow’s bundle, respectively. He was also the first to identify the arcuate fasciculus as the tract connecting the Broca and Wernicke areas, a view later adopted by Wernicke himself in 1908 [46]. von Monakow was particularly interested in the timed sequence in which cortical and subcortical components participated in brain functions, a principle that he referred to as ‘chronogenic localization’. He was convinced that the spatial representation of functions can vary over time and, for instance, emphasized that aphasia can be absent in the case of slowly growing tumours in Broca’s area.
von Monakow’s ideas can be seen as a bridge between localizable and non-localizable concepts of functional organization. His work was acknowledged by scientist such as Kurt Goldstein (1878–1965) and Aleksandr Luria (1902–1977), who themselves had moved away from classic localistic views. As Goldstein wrote in 1934 in his book The Organism:
In summarizing our discussion of the possibility of a correct anatomical evaluation, as a basis for localization, one thing seems certain: it renders rather unsatisfactory service to determine simply the location of a lesion. Whether a symptom will appear on account of a local injury, especially whether it will become a permanent symptom, certainly depends on many factors: on the nature of the disease process, on the condition of the rest of the brain, on the state of the circulation, and on the psycho-physical constitution of the patient. It also depends on the ‘difficulty’ of that performance, the disturbance of which represents the symptom, and, finally, on the reaction of the entire organism to the defect [47].
Goldstein rejected classic neurological theories on several grounds and provided alternative views not only for the representation of functions, but in particular for the treatment and rehabilitation of brain-damaged patients. He studied and treated the injured patient as a ‘whole’ and focused on altered behaviour and not only on focal neurological symptoms such as dysphasia or motor disturbances. The complex neurological and behavioural disturbances that he had seen in the many World War I casualties had broadened his scientific and clinical view. He did not merely see symptoms as the consequence of a single damaged brain area, but rather as an ‘attempted solution’ of an organism that dealt with disease [47]. Certain symptoms, so he stated, were the direct consequence of damage to a particular part of the brain. However, he considered many other symptoms ‘the expression of the struggle of the changed organism to cope with the defect, and to meet the demands of a milieu with which it is no longer equipped to deal’ [48].
Oliver Sacks (1933–2015) wrote in a foreword to one of Goldstein’s reprinted books that he was ‘one of the most important, most contradictory, and now most forgotten figures in the history of neurology and psychiatry’ [47]. To Sacks, when he read the works in the 1950s as a medical student, Goldstein’s work seemed to have:
a vigor, a vitality, a largeness of vision, that radically contrasted with the tight atmosphere of classical neurology in which we were (…) being educated. (…) He talked about ‘reactions’ to illness, about ‘adaptation’, ‘compensation’, ‘coming to terms’, reactions that we could see in our patients all the time and that were crucial to understand if any rehabilitation was to be achieved, but ones that our textbooks completely ignored [47].
Although Goldstein acknowledged that sensorimotor functions or some of the motor abilities in speech could be linked to certain specific areas, he held that impairment of cortical functions was always more or less related to global changes in cortical organization. As he wrote in 1942:
Localization of a performance means to me not an excitation in a certain place, but a dynamic process which occurs in the entire nervous system, even in the whole organism, and which has a definite configuration for each performance. (…) A specific location is characterized by the influence which a particular structure of that area exerts on the total process (…). However, that does not exclude the fact that we consider some symptoms more closely related to some areas and can make our decision, for practical purposes, on this basis [48].
Goldstein, and later Luria, thus strongly believed that no form of mental activity could be related to a localized and limited group of nerve cells or area [49].3 Cortical and subcortical areas operated in concert, and each made a specific contribution to the complex ‘dynamic structure’ that constitutes a function. Luria (1947, 1970) stressed that we should not speak of ‘localization of function’—as that is never possible—but rather of ‘localization of symptoms’:
This position which has been so strongly defended by Goldstein was originally formulated by Jackson. According to his conception, a focal lesion of the brain which disturbs a process necessary for the performance of a certain function produces a symptom. In this sense the symptom is correlated with the site of the damage, i.e. ‘localized’ to a certain area of the cortex [49].
Luria dedicated a great deal of his professional life to the study of brain-injured patients. He was one of the first researchers who realized that analysis of the functional impairments of focal lesions had important implications for the study of normal (and not only abnormal) functional systems. Before him, researchers had mainly tried to establish a correlation between function and the structure of the damaged region, thereby largely ignoring non-local effects or the recovery potential of the undamaged part of the brain. Lucia’s dynamic functional system was in line with previous work by Goldstein and in particular Hughlings Jackson, who he often cited and was keen to acknowledge. These authors spoke of ‘disintegration’ or ‘impairment’ of functions, and not so much of a ‘loss’ of function. A function, says Luria, is seldom completely lost in patients with spatially restricted brain lesions.
Luria was an exceptionally talented observer, who had learned from the large number of war casualties that traumatic speech deficits could be reduced or overcome by suitable therapy. He also deduced from his many cases that functional deficits had no simple relationship to the area that was damaged. There were simply too many different functional impairments that could develop when a single area of cortex was damaged:
If (…) damage to the posterior-superior parts of the left temporal area disrupts normal activity in the system of structures responsible for acoustic analysis and synthesis, then it cannot but produce a disturbance of auditory speech (phonemic hearing). In doing this it must affect all forms of activity in which phonemic hearing and the connections related to it play a role. With the disturbance of structured hearing it is impossible to maintain precise and differentiated speech, to find necessary words, or to perform sound analysis and synthesis necessary for writing. When this basic disturbance is present, secondary symptoms inevitably develop as indirect or compensatory effects. Literal and verbal paraphasias and disturbances in the grammatical structure of speech appear, but such functions as object recognition, orientation in space, and calculation involving elementary written numbers remain unimpaired [49].
In line with von Monakow’s theories on diaschisis, Luria proposed that functional disturbances are caused by two major mechanisms. First, there is functional loss due to damage of a particular cortical area. Second, there is a functional disturbance of several distant cortical areas, ‘very likely as the result of loss of the normal conduction of excitation in the areas directly involved’ [49]. As Luria considered the local damage to a large extent irreversible, he proposed that recovery of function must either be associated with a resolution of diaschisis or with a major reorganization of cortical processes [49]. To him, the latter was the major mode of recovery in most cases of focal brain damage. In this aspect, his opinion differed from that of von Monakow.
9.1.5 Mechanisms of Neural Plasticity
Jerzy Konorski (1903–1973), a Polish neuroscientist, gave one of the first modern definitions of neuroplasticity, emphasizing the role of structural changes in already existing pathways.4 In Konorski’s view, neurons can immediately react to incoming changes (‘excitability’), leading to a ‘permanent transition’ of a system of neurons which he called plasticity [37]. Over time, there have been many definitions, basically all revolving around the concept of change. Plasticity is thereby considered a natural and lifelong property of the brain, enabling it to change its structure in response to experience. It is driven primarily by an imbalance between functional performance and environmental demands [52]. It is the key principle of learning and memory, but is also acting when the brain is aging or affected by disease. After damage to the nervous system, due to for instance a tumour or stroke, it aims to restore the functional homeostasis of the nervous system.
Animal experiments have revealed several underlying microscopic processes that allow the brain to change its functionality. The reader is referred to the extensive literature on this subject. In short summary these mechanisms are axonal sprouting (allowing for new connections), unmasking of connections, neurogenesis, neuron–glia interaction, modification of synaptic weights, formation of new synaptic connections, and cell death and synaptic pruning (for fine-tuning of connectivity) [53–56]. At the macroscopic level, plastic changes involve restoration or redistribution of function within networks, as well as development of new compensatory cognitive strategies. These processes are still not much understood, but are increasingly studied with functional neuroimaging techniques (fMRI, PET, TMS). Although it is sometimes stated that neuronal plasticity aims to optimize the organism’s behaviour, or that the brain can even ‘change itself’, it should be remembered that there probably exists no intrinsic frame of reference against which the brain can compare its results (in a similar manner that evolutionary processes do not strive for a particular goal, but are merely the result of competition). Effects of plasticity can be positive or negative, but that is ultimately a subjective judgement.5
9.1.6 Redundancy
Alternatively, individual brain functions may be represented by more than one neural representation to begin with. In this view, that is known as multiple realizability, there is not a one-to-one but a one-to-many relationship between functional and physical brain states (see, for instance, Overgaard and Mogensen (2011) [59]). The notion that functional information is stored in multiple different anatomical configurations would explain the brain’s redundancy to (sub)cortical damage, as well as its capacity to regain functionality.
Redundancy to brain damage is a common observation in clinical practice. Neurosurgeons, for example, frequently place ventricular catheters or electrodes via an entrance that requires a small cortical lesion, or remove a tumour via a larger cortical opening without the introduction of new neurological deficits. More ‘natural’ causes also do damage to the brain without acute behavioural consequences. Aging, for instance, leads to an accumulation of small white matter lesions. Of course, all of these damaged areas were never afunctional before, but part of a larger brain network that apparently almost instantaneously compensated for the loss of a part of it. This seems only possible if there is enough redundant capacity within the network to maintain its performance. From a theoretical perspective, at least, any lesion would have to come at some cost (e.g. speed of informational processing or accuracy of behaviour). In practice, these effects are often subclinical and not measurable in neurological or behavioural terms.
Redundancy, in one form or another, has a long history in the discussion on cerebral localization of function. Already, in 1888, Golz pointed out that small cortical lesions in animals had absolutely no effect on their behaviour and that remaining regions were equally capable of supporting these functions [60]. Even larger lesions, so he concluded, produced surprisingly small effects due to this mechanism [1]. Lashley, in the 1930s, hypothesized an extreme equipotentiality for all cortical areas that were involved in sensory and perceptual processes, based on his extensive brain-lesioning experiments in rats [61]. He concluded that in these animals, behavioural impairments were simply related to the amount of tissue that was removed.
9.2 The Lateral Shift Hypothesis
Already at the end of the nineteenth century, the idea was discussed that not only left hemispheric areas could potentially play a role in the recovery of language functions. Many scholars, including Broca and Wernicke, considered the right hemisphere a ‘backup’ in case of damage to the left hemisphere. In fact, this was not so much of an issue at the time, perhaps because earlier ideas on brain functions—before hemispheric specialization became the dominant view—considered both sides of the brain functionally equivalent. As Head (1926) summarized:
Normally in right-handed persons the right hemisphere is a vast uncultivated field, which plays a subservient or secondary part in the mechanism of speech. Impressions received by the sensory surfaces are worked up by centers of the left hemisphere into factors, which underlie the highest forms of logical thinking. These are transferred to the opposite site, to be stored up as unconscious memories in this special seat of latent and automatic psychical activities. Under normal conditions centers is the right hemisphere responsible at most for affective or interjectional speech; but as the result of suitable education and training they may play a material role in the re-acquisition of power to employ language [10].
In 1887, William Gowers (1845–1915) was among the first to provide evidence that the right hemisphere might indeed contribute to recovery of language functions [62]. He noted that in patients that had recovered from aphasia due to a stroke in the left hemisphere, a second lesion in the right hemisphere sometimes induced worsening of language functions. Gowers argued that this was powerful evidence for a role of the right hemisphere in the initial recovery from aphasia after the first left hemisphere stroke:
Loss of speech due to permanent destruction of the speech region in the left hemisphere has been recovered from, and that this recovery was due to supplemental action of the corresponding right hemisphere is proved by the fact that in some cases, speech has been again lost when a fresh lesion occurred in this part of the right hemisphere [24].
At the time, Thomas Barlow (1845–1945) presented a case that was subsequently cited by many of his contemporaries as strong evidence for a functional takeover of speech functions by the right hemisphere [63]. However, the case lends itself to different interpretations. Interestingly, Barlow himself never discussed reorganization of speech functions. The case is shortly described in Fig. 9.3 and was critically reviewed before by Finger (2003) and Hellal (2007) [64, 65].


Fig. 9.3
Barlow’s case (1877) of a patient who presented with two brain infarcts, first on the left side and later on the right side [63]. The case report was seen by many of Barlow’s contemporaries as strong evidence for the ability of the right hemisphere to restore speech functions after damage. Barlow described the medical history of a 10-year-old boy who suddenly developed a right-sided hemiplegia, paralysis of the tongue and severe difficulties with speech and swallowing. In the weeks afterwards, the patient made a near complete recovery. Unfortunately, 4 months after the first insult, a hemiplegia on the other (left) side occurred, and speech again deteriorated. After initial recovery of function, the boy died 2 months later. Autopsy revealed that he had died from cardiac disease that had caused embolic infarctions in several organs including the brain (first on the left side and later on the right). Fairly small but nearly symmetrical lesions were noted on both sides of the brain (bottom figure in Fig. 9.3 is an Ecker figure that shows the lesion in the left hemisphere): ‘on each side, these regions consisted of the lower end of the ascending frontal and the hinder end of the middle and inferior frontal convolutions. These areas were pale, buff coloured, slightly depressed and slightly softer than the surrounding brain substance. Reckoning from the surface, they were less than a quarter of an inch deep, i.e. they involved the cortical and a little of the subjacent white substance [63]’. Remarkably, Barlow himself never suggested that the right hemisphere had taken over speech functions from Broca’s area. He attributed the speech disorder to damage to the face area of the motor cortex and referred to the experiments of Ferrier, who had shown that stimulation of inferior frontal areas in monkeys—in a homologue location to those of his patient—results in movement of mouth and tongue (top figure in Fig. 9.3 from Ferrier and modified by Barlow for his publication). ‘The bilateral muscles, which act together, are represented on the two sides of the brain. After the first attack of hemiplegia, although this region on the left side was probably permanently damaged, yet still the right side remained intact. But, after the second attack of hemiplegia, the corresponding region on the right side became damaged; and henceforth, as far as voluntary movements of the mouth and tongue were concerned, the boy was irretrievably deficient’. As Finger (2003) concluded in his analysis of the case [64]: ‘thus, from Barlow’s perspective, the quite capable and partially redundant right motor cortex was simply left with sole control of the bilateral mouth musculature after the boy’s left hemisphere was severely damaged and the remote effects of the insult subsided. But after the right hemisphere subsequently suffered ischemic damage, neither side of the brain still possessed the circuitry needed to control these muscles voluntarily. This was why the boy was “irretrievably deficient” when he now tried to converse [64]’ (Figures and text taken from Barlow (1877) [63])
Others pleaded for a language role of the right hemisphere already in the normal, healthy situation. Hughlings Jackson was one of the first who considered both hemispheres to be important for language functions. Although he agreed with Broca’s statement that a loss of speech was associated with damage to the left hemisphere, he believed that comprehension was a bilateral function. As Finger (2001) wrote:
He theorized the right hemisphere could still learn the meaning of words as a result of associative laws (hearing of the word “horse” while also seeing a horse) and that it possessed a consciousness for place and things. Yet, it seemed clear to him that only the left hemisphere could truly become “conscious in words”. (…) Jackson (1868) accepted the belief that the left frontal lobe grew faster than the right frontal lobe and took the lead in voluntary speech. He also believed the right posterior lobe grew in advance of that on the left, and from this he deduced that the posterior right hemisphere was the leading part for perception and imagination [66].
9.2.1 Broca
Broca’s view of the right hemisphere’s role in language is surprisingly different to his legacy of strict left hemisphere language dominance, and resembles some of the contemporary thoughts about this issue [11]. For that reason, I will discuss it at some length here. According to Broca, ‘articulated speech’ is normally (i.e. in healthy conditions) the privilege of the left hemisphere. To explain this organic predisposition, he referred to the works of Gratiolet and Bertillon, who had provided evidence that left hemisphere development starts earlier than that of the right hemisphere. As it is thus more precocious at birth, it best fits to encompass language functions at a young age. ‘The tendency to speak with the left hemisphere is born’, so wrote Broca in his 1865 paper [67]. But Broca did not link language functions exclusively to the left hemisphere. In that same paper he also explained that the ‘connection’ between ideas and words is a capacity that belongs to both hemispheres. Proof of this, according to Broca, is seen in patients with deep and extensive lesions in the left hemisphere. These patients are disabled in their speech, but continue to understand what is spoken to them. Each hemisphere can therefore, in case of a lesion or disease, ‘reciprocally substitute for each other; however, the faculty to express them by means of coordinated movements, in which the practice requires a very long period of training, appears to belong to but one hemisphere, which is almost always the left one’ [67].
In Broca’s view, both hemispheres are thus capable of language comprehension, hence the possibility of functional recovery after brain damage (note that a left temporal language centre was only to be discovered a decade later). In order for people to become right-brained, Broca assumed that the left third frontal convolution was involved in an injury very early in life. Under those circumstances, the right homologue area would substitute and have the ability to learn to speak. He compared this process to a child that is born without a right hand, but still becomes as skilful with his remaining left hand as he would have ordinarily been with the other. Broca stressed that the mastery of articulated speech is complex and difficult, something that a child only manages to succeed after long and tentative efforts ‘of the most complicated degree’ [67]. Broca was convinced that training would be very beneficial for functional recovery in patients, but it should be given with the same intensity and duration as a child that learns to speak. These patients, so he concluded, should be treated with the ‘tireless patience of a mother who teaches her son how to speak’ [67]. But obviously that was not done at that time.
Broca’s ideas were supported by one of his cases from the Salpêtrière Hospital. During the autopsy of a 47-year-old patient, he noted that the third left frontal convolution was lacking, along with the inferior parietal convolution and the superior temporal convolution [67]. In other words, the convolution d’enceinte was missing (see Fig. 1.4 in Chap. 1). In addition to this, several other areas in the left hemisphere were underdeveloped. Broca concluded that there was a congenital atrophy of the left side of the brain and speculated that this was due to congenital absence of the Sylvian artery that he was unable to find during the autopsy. The patient had seizures from a very early age and had atrophic and paretic right extremities. But there were no obvious language problems. Broca concluded that in this case there was no doubt that the right inferior frontal convolution was involved in language functions. Immediately, he asked himself a much broader question: why does such a functional compensation not occur in all cases of aphémie? If both hemispheres can indeed contribute to language functions, then a lesion in only one hemisphere should not be enough to cause lasting language impairments. But why was this not the case in everyday practice? To explain this, Broca assumed that larger lesions generally affected the intellect of most patients. Consequently, he stated that their ‘weakened mind’ prevented them from learning to speak exclusively with the right hemisphere, ‘which up to now had only played an accessory role in the function of expression by means of articulated speech’. So it is not so much that the right hemisphere is not capable of language functions, but that these patients lack the cognitive abilities to learn to speak again and to complete the intensive training that is needed for successful rehabilitation.
Broca was one of the first to consider a ‘takeover’ of language functions by the right hemisphere [68]. Since the latter half of the nineteenth century, this explanation has been frequently brought forward in particular in patients with a damaged left hemisphere but only minor language impairments. This lateral shift hypothesis is certainly very intuitive, something that undoubtedly contributed to its remaining popularity. However, the evidence for it is rather meagre, and cohesive theories are still lacking [69]. Code, in 1987, wrote that it is predominantly based on ‘a loose collection of clinical anecdotes and speculation’ [68]. Even with the advent of modern neuroimaging techniques, the issue has not been settled. Careful interpretation of results, though, indicates that the left hemisphere very often remains critically involved in language functions, as will be explained in the next sections.
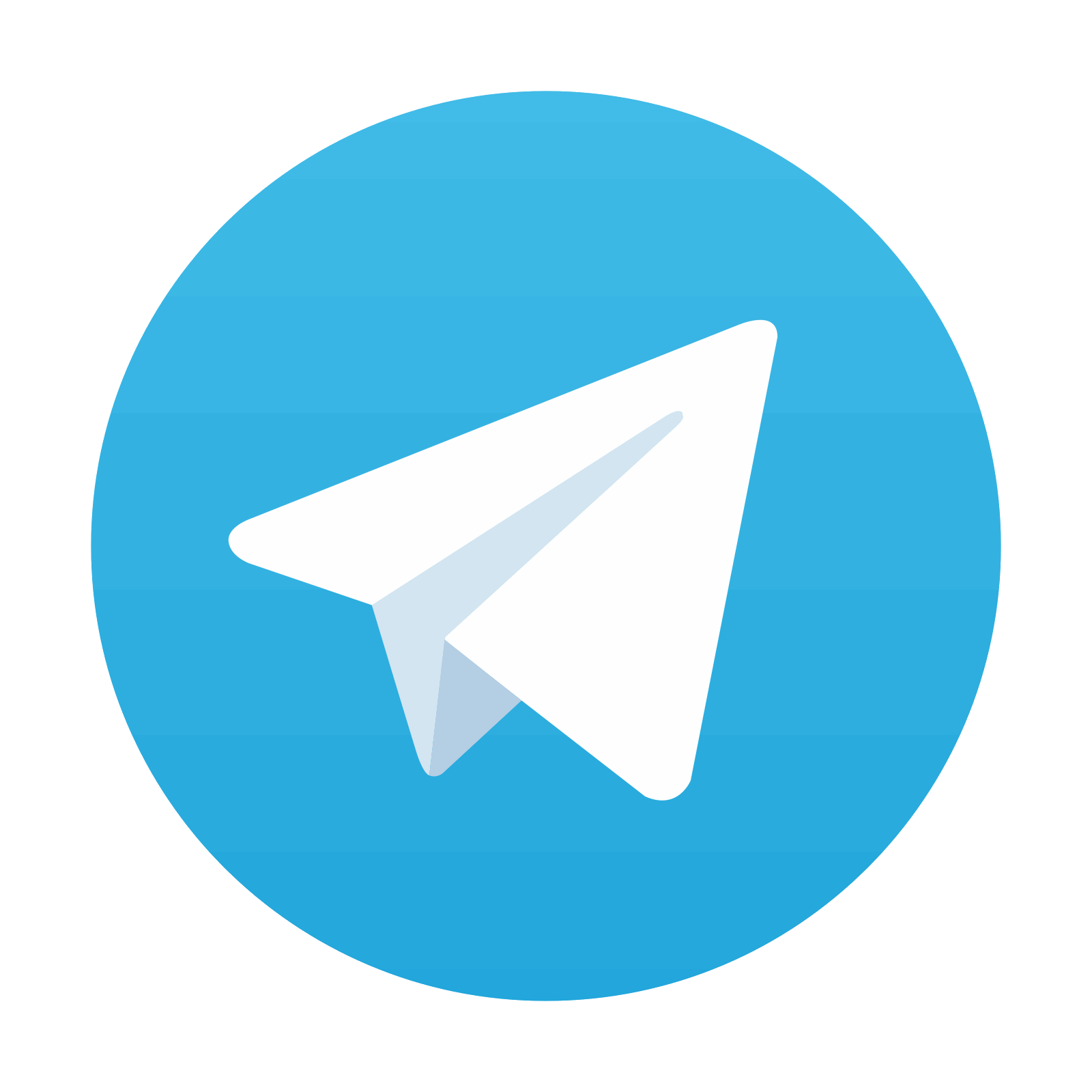
Stay updated, free articles. Join our Telegram channel

Full access? Get Clinical Tree
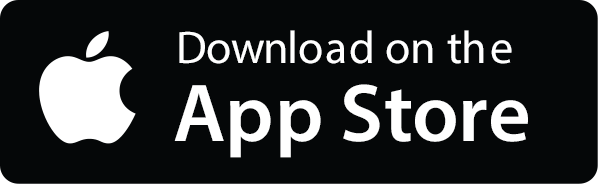
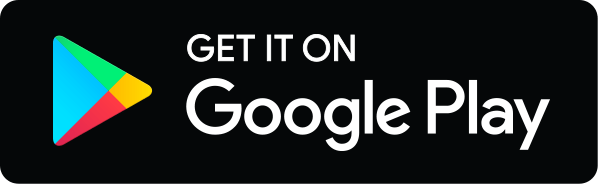