The defining feature of addiction is compulsive, out-of-control drug use, despite negative consequences.
Addictive drugs induce pleasurable states or relief from distress, thus motivating repeated drug use.
Drugs of abuse are both rewarding and reinforcing. Rewards are stimuli that the brain interprets as intrinsically positive, and reinforcing stimuli are those that increase the probability that behaviors paired with them will be repeated.
The brain reward circuitry targeted by addictive drugs, which normally responds to natural reinforcers such as food and sex, includes the dopaminergic projections from the ventral tegmental area (VTA) of the midbrain to the nucleus accumbens (NAc) and other forebrain structures.
Repeated use of addictive drugs produces multiple unwanted changes in the brain that may lead to tolerance, sensitization, dependence, and addiction.
Dependence is an adaptive state that develops in response to repeated drug administration; when unmasked by cessation of drug use, this adapted state may lead to withdrawal symptoms.
Tolerance refers to the diminished effect of a drug after repeated administration at the same dose, or to the need for an increase in dose to produce the same effect; sensitization describes the opposite response to repeated drug administration.
Cocaine and amphetamines produce their psychoactive effects by potentiating monoaminergic transmission through actions on the dopamine transporter, together with actions on the serotonin and norepinephrine transporters.
The reinforcing effects of opiate drugs result from their binding to endogenous opioid receptors, most importantly μ opioid receptors in both the VTA and NAc.
The immediate effects of ethanol are believed to result primarily from facilitation of GABAA receptors and inhibition of NMDA glutamate receptors. At higher doses, ethanol inhibits the functioning of many ion channels as well.
The effects of nicotine are caused by its activation of nicotinic acetylcholine (nACh) receptors; its reinforcing effects depend in part on nACh receptors located on VTA dopamine neurons.
Delta-9-tetrahydrocannabinol, the active psychotropic ingredient in marijuana, exerts its primary pharmacologic effects by binding to a G protein–coupled receptor in the brain known as the CB1 receptor.
The psychotomimetic drugs of abuse, phencyclidine (angel dust, PCP) and ketamine, bind specific sites in the channel of the NMDA glutamate receptor, where they act as noncompetitive NMDA antagonists.
Drugs of abuse cause addiction by inducing a range of molecular and cellular adaptations in several brain reward regions, which are becoming increasingly well known.
Drug addiction is a progressive and often fatal behavioral syndrome characterized by compulsive drug seeking and consumption despite serious negative consequences. The drug-centered existence of addicts can cost them their jobs, personal relationships, financial standing, happiness, and, in some cases, their lives. Drug-addicted individuals often appear to have lost the ability to make choices that promote their own happiness and survival. Many drug addicts who seek treatment report that they realize the destructive nature of their addiction but are unable to alter their addictive behavior.
In laboratory settings in which social and environmental variables are controlled, normal animals with access to addictive drugs typically engage in self-administration of these substances. Such behavior indicates that addiction is the result of a conserved neurobiologic substrate in animal and human brains that is vulnerable to regulation by addictive drugs. The actions of such drugs on this neural substrate tend to promote continued drug-taking behavior in a way that becomes increasingly involuntary. In both animals and humans, roughly 50% of the risk for addiction is genetic, but the specific genes that comprise that risk remain almost completely unknown.
Understanding the biologic determinants of addiction and the biologic factors responsible for individual vulnerability to addiction will aid in the development of truly effective treatment and prevention strategies. Thus, it is important to determine (1) the neurochemical and anatomic basis, and naturally intended function, of the reward circuitry in the healthy brain, and (2) the changes in this circuitry produced by addictive drugs that cause the addicted brain to be fundamentally different from a drug-free brain.
Addictive drugs are both rewarding and reinforcing. A reward is a stimulus that the brain interprets as intrinsically positive or as something to be approached. A reinforcing stimulus is one that increases the probability that behaviors paired with it will be repeated. Not all reinforcers are rewarding; for example, a negative or punishing stimulus might reinforce avoidance behaviors.
Drug-induced pleasurable states are important motivators of initial drug use. Drug actions that produce these states also produce associated, but ultimately undesirable, changes in brain reward circuitry that promote future drug use. Another form of reinforcement involves the alleviation of unpleasant symptoms—either from preexisting states or caused by drug withdrawal—by means of drug use. Conditioned reinforcement, which occurs when previously neutral stimuli become associated with the pleasurable effects of drugs, is another type of positive reinforcement. All of these mechanisms contribute to repetitive drug taking that, in vulnerable individuals, may result in an addicted state.
The reinforcing effects of drugs can be demonstrated in animals, where rodents and nonhuman primates readily self-administer certain drugs by pressing a lever or placing their noses in an aperture. In this self–administration paradigm, the amount of work (number of lever presses or nose pokes) an animal does to gain access to a given amount of drug indicates the strength of reinforcement induced by the drug. The strength with which different drugs reinforce behavior in animals correlates well with their tendency to reinforce drug-seeking behavior in humans. Cocaine, for example, is highly reinforcing when injected intravenously. Laboratory animals readily self-administer this drug, and some of them will give up survival necessities, such as food and water, or work excessively, even to the point of death, in order to gain access to cocaine. Such evidence of the power of cocaine’s reinforcing properties helps to explain its addictiveness in humans. In general, drugs that are less addictive in humans (such as marijuana) are not as likely to be self-administered by animals, and drugs that are not addictive in humans are not reinforcing in or self-administered by animals. Nicotine appears to represent an exception to this rule; although it does not strongly reinforce drug-seeking behavior in animals, it produces strong addiction in some humans.
Another paradigm used to investigate drug reward in animals is known as conditioned place preference, where animals learn to associate a particular environment with passive drug exposure. For example, a rodent will learn to spend more time on the side of a box where it was previously given cocaine. This paradigm reflects the strong cue-conditioned effects of addictive drugs and provides an indirect measure of drug reward. In the conditioned reinforcement paradigm, animals learn to associate a neutral cue, such as light, with a natural reinforcer, such as water. With sufficient training, the neutral cue becomes a conditioned reinforcer, namely, something the animals will work (lever press, nose poke) to obtain. Addictive drugs dramatically potentiate the degree to which animals will work for conditioned reinforcers.
The neural substrates that underlie the perception of reward and the phenomenon of positive reinforcement are a set of interconnected forebrain structures called brain reward pathways 16–1; these include the nucleus accumbens (NAc; the major component of the ventral striatum), the basal forebrain (components of which have been termed the extended amygdala, as discussed later in this chapter), hippocampus, hypothalamus, and frontal regions of cerebral cortex. These structures receive rich dopaminergic innervation from the ventral tegmental area (VTA) of the midbrain. Addictive drugs are rewarding and reinforcing because they act in brain reward pathways to enhance either dopamine release or the effects of dopamine in the NAc or related structures, or because they produce effects similar to dopamine.
16–1
Neural reward circuits for various drugs (cocaine, amphetamines, opiates, nicotine, and ethanol) in a sagittal section of rat brain. A limbic–extrapyramidal motor interface is apparent. Dashed lines indicate limbic glutamatergic afferent inputs to the nucleus accumbens (NAc). Purple lines represent efferent signals from the NAc believed to be involved in drug reward. Bold blue lines indicate projections of the mesocorticolimbic dopamine system, which are believed to be critical substrates for drug reward. This dopamine system originates in the ventral tegmental area (VTA) and projects to the NAc, olfactory tubercle, ventral striatal domains of the caudate–putamen (C–P), and amygdala (AMG), among other regions. Red dashed lines represent major glutamatergic inputs to the NAc. Brown lines indicate opioid peptide–containing neurons, which comprise systems that may be involved in opiate, ethanol, and possibly nicotine reward; these systems include local enkephalinergic circuits (short segments) and the hypothalamic β-endorphin circuit (long segment). Gold areas indicate the approximate distribution of GABAA receptor complexes that may mediate sedative/hypnotic (ethanol) reward. Green solid structures indicate nicotinic acetylcholine receptors, which are located on dopaminergic, opioid peptidergic, and glutamatergic neurons. AC, anterior commissure; ARC, arcuate nucleus; Cer, cerebellum; DMT, dorsomedial thalamus; FC, frontal cortex; Hippo, hippocampus; IF, inferior colliculus; LC, locus coeruleus; LH, lateral hypothalamus; OT, olfactory tract; PAG, periaqueductal gray; RPn, raphe pontis nucleus; SC, superior colliculus; SNr, substantia nigra pars reticulata; VP, ventral pallidum. (Adapted with permission from Koob GF. Drugs of abuse: anatomy, pharmacology and function of reward pathways, Trends Pharmacol Sci. 1992 May;13(5):177-184.)

Over 50 years ago, animals were shown to press a lever to electrically stimulate discrete brain regions, which provided the first direct evidence for specific neural substrates in the brain that are capable of mediating reinforcement. Although such intracranial self–stimulation of several brain structures is reinforcing, stimulation of the medial forebrain bundle and closely associated areas results in the strongest reinforcement of paired behavior. Addictive drugs and reinforcing electrical brain stimulation activate the same brain reward circuitry, as suggested by the synergistic effect that addictive drugs have on brain stimulation reward (BSR) thresholds; in the presence of drugs, less stimulation is needed to produce a particular response. Virtually all addictive drugs enhance BSR. Reinforcement produced by stimulation of the medial forebrain bundle is caused in large part by activation of axons of VTA dopamine neurons coursing toward the NAc and other forebrain reward structures 16–1. There also appear to be dopamine-independent mechanisms of the reinforcing effects of medial forebrain bundle self-stimulation. The nature of these mechanisms is unknown, although certain hypothalamic peptides have been speculated to be involved.
The brain reward circuitry that is targeted by addictive drugs normally mediates the pleasure and strengthening of behaviors associated with natural reinforcers, such as food, water, social interactions, and sexual contact. Dopamine neurons in the VTA are activated by food and water, and dopamine release in the NAc is stimulated by the presence of natural reinforcers, such as food, water, or a social or sexual partner. Thus, drugs tap into neural networks that apparently evolved to reinforce behaviors that are necessary for survival and reproduction. These systems can be viewed as complementary to survival networks in the brain that mediate learning about dangerous and harmful stimuli (Chapter 15).
Sensory cues produced by natural reinforcers activate reward pathways under normal circumstances, whereas addictive drugs directly activate the same neural circuitry by chemical means 16–1, bypassing the need for evolutionarily useful behaviors. Hence, the powerful control over behavior exerted by addictive drugs may stem from the brain’s inability to distinguish between the activation of reward circuitry by drugs and natural activation of the same circuitry by useful behaviors. Any activity, whether related to drug taking or survival, that activates this circuitry is regarded as one that should be repeated. Moreover, chemical activation of reward circuitry by addictive drugs can be much more powerful and sustained than activation triggered by natural reinforcers. Exposure to addictive chemicals not only produces extreme euphoric states that may initially motivate drug use but also causes equally extreme adaptations in reinforcement mechanisms and motivated behavior that eventually lead to compulsive use. Accordingly, the evolutionary design of human and animal brains that has helped to promote our survival also has made us vulnerable to addiction.
Drug | Action |
---|---|
Opiates | Agonist at μ, δ, and κ opioid receptors1 |
Cocaine | Inhibits monoamine reuptake transporters |
Amphetamine | Stimulates monoamine release2 |
Ethanol | Facilitates GABAA receptor function and inhibits NMDA glutamate receptor function3 |
Nicotine | Agonist at nicotinic acetylcholine receptors |
Cannabinoids | Agonist at cannabinoid (CB1 and CB2) receptors4 |
Hallucinogens | Partial agonist at 5HT2A serotonin receptors |
Phencyclidine (PCP) | Antagonist at NMDA glutamate receptors |
Inhalants | Unknown |
Repeated exposure to an addictive drug induces profound cellular and molecular changes within neurons of the brain reward circuitry, which in turn are believed to cause the alterations in reinforcement mechanisms that contribute to addiction. Drug-induced adaptations reflect both homeostatic compensations for excessive stimulation by drugs and alterations in multiple memory-related systems in the brain that serve to sustain addiction over long periods of time. These adaptations gradually alter normal control of motivated behavior, and eventually produce the compulsive and increasingly involuntary drug-seeking behavior that characterizes addiction. Thus, compared with the normal brain, the addicted brain programs behave in a fundamentally different way that can be long-lasting and perhaps even permanent.
Familiar pharmacologic terms such as tolerance, dependence, and sensitization are useful in describing some of the time-dependent processes that underlie addiction. Tolerance refers to the diminishing effect of a drug after repeated administration at the same dose, or to the need for an increase in dose to produce the same effect. Tolerance may develop to some of the effects of a drug but not to others; for example, tolerance frequently develops to the analgesic, euphoric, and respiratory depressant effects of opiates, but not to the pupillary constriction produced by these drugs. Pharmacokinetic tolerance is caused by increased drug metabolism or clearance, whereas pharmacodynamic tolerance is a result of adaptations in the neural elements that respond to drugs initially. Pharmacodynamic tolerance is the more important mechanism in terms of its contribution to behavior, including addiction. Sensitization, also referred to as reverse tolerance, occurs when repeated administration of the same drug dose elicits escalating effects. Dependence is defined as an adaptive state that develops in response to repeated drug administration, and is unmasked during withdrawal, which occurs when drug taking stops. Dependence resulting from long-term drug use may have both a somatic component, manifested by physical symptoms, and an emotional–motivational component, manifested by dysphoria and anhedonic symptoms, that occur when a drug is discontinued. While physical dependence and withdrawal occur dramatically with some drugs of abuse (opiates, ethanol), these phenomena are not useful in the diagnosis of an addiction because they do not occur as robustly with other drugs of abuse (cocaine, amphetamine) and can occur with many drugs that are not abused (propranolol, clonidine).
The official diagnosis of drug addiction by the Diagnostic and Statistical Manual of Mental Disorders (2013), which uses the term substance use disorder, is flawed. Criteria used to make the diagnosis of substance use disorders include tolerance and somatic dependence/withdrawal, even though these processes are not integral to addiction as noted. It is ironic and unfortunate that the manual still avoids use of the term addiction as an official diagnosis, even though addiction provides the best description of the clinical syndrome.
Cocaine, amphetamines, and methamphetamine are the major psychostimulants of abuse. The related drug methylphenidate is also abused, although it is far less potent. These drugs elicit similar initial subjective effects 16–2; differences generally reflect the route of administration and other pharmacokinetic factors. Such agents also have important therapeutic uses; cocaine, for example, is used as a local anesthetic (Chapter 2), and amphetamines and methylphenidate are used in low doses to treat attention deficit hyperactivity disorder and in higher doses to treat narcolepsy (Chapter 13). Despite their clinical uses, these drugs are strongly reinforcing, and their long-term use at high doses is linked with potential addiction, especially when they are rapidly administered or when high-potency forms are given.
Cocaine and amphetamines produce their psychoactive effects by potentiating monoaminergic transmission 16–2 through actions on dopamine, serotonin, and norepinephrine transporters, although the precise mechanisms underlying this potentiation vary 16–3. These proteins normally transport synaptically released neurotransmitter back into the presynaptic nerve terminal and thereby terminate transmitter action (Chapter 6). Actions at the dopamine transporter (DAT) are the most important for the reinforcing effects of these drugs; for example, mice with a null mutation in the DAT gene are much less sensitive than normal mice to the behavioral effects of cocaine or amphetamines.
16–2
Highly simplified scheme of converging acute actions of drugs of abuse on the VTA–NAc. Stimulants directly increase dopaminergic transmission in the NAc. Opiates do the same indirectly: they inhibit GABAergic interneurons in the VTA, which disinhibits VTA dopamine neurons. Opiates also directly act on opioid receptors on NAc neurons, and opioid receptors, like D2 dopamine receptors, signal via Gi; hence, the two mechanisms converge within NAc neurons. The actions of the other drugs remain more conjectural. Nicotine activates VTA dopamine neurons directly via stimulation of nicotinic cholinergic receptors on those neurons, and indirectly via stimulation of its receptors on glutamatergic nerve terminals that innervate the dopamine cells. Ethanol, by promoting GABAA receptor function, may inhibit GABAergic terminals in VTA and hence disinhibit VTA dopamine neurons. It may similarly inhibit glutamatergic terminals that innervate NAc neurons. Cannabinoid mechanisms involve activation of CB1 receptors (which, like D2 and opioid receptors, are Gi linked) on glutamatergic and GABAergic nerve terminals in the NAc and possibly on NAc neurons themselves. PCP may act by inhibiting postsynaptic NMDA glutamate receptors in the NAc. Finally, evidence suggests that nicotine and ethanol may activate endogenous opioid pathways, and that these and other drugs of abuse (eg, opiates) may activate endogenous cannabinoid pathways (not shown).

16–3
Mechanism of action of cocaine and amphetamine on monoamine nerve terminals. A. Cocaine potentiates the actions of monoamines at the synapse by inhibiting monoamine transporter proteins, which normally carry previously released transmitter back into the nerve terminal. B. Amphetamine serves as a substrate for monoamine transporter proteins and is transported into the nerve terminal. In the nerve terminal, amphetamine disrupts the vesicular storage of monoamine transmitters, which leads to an increase in their extravesicular levels; consequently, these transmitters are pumped out of the nerve terminal by a reverse action of the transporters. Recent research has demonstrated the involvement of dopamine transporter trafficking to and from the nerve terminal plasma membrane in the actions of psychostimulants.

The reinforcing effects of cocaine and amphetamines require an intact mesolimbic dopamine system. Systemic administration of dopamine antagonists, or of the dopamine synthesis inhibitor α-methylparatyrosine (AMPT), decreases self-administration; in contrast, antagonists of various adrenergic or serotonergic receptors have little effect on such behavior. Selective antagonists for multiple dopamine receptor subtypes (D1, D2, and D3) are effective in decreasing the reinforcing actions of cocaine. Dopamine levels are increased in the NAc during self-administration of cocaine or amphetamines, as mentioned previously, and blockade of dopaminergic transmission in the NAc—for example, in response to intra-NAc injections of dopamine receptor antagonists or of the toxin 6-hydroxydopamine (Chapter 6)—dramatically reduces drug reinforcement. Dopamine in the NAc exerts complex effects on γ-aminobutyric acid (GABA)ergic medium spiny projection neurons, with excitation or inhibition seen depending on several factors including the predominant form of dopamine receptor expressed in a particular cell (see the section “Extended Reward Circuitry”). However, how altered excitability of these neurons contributes to drug reinforcement remains unknown.
Methamphetamine is an amphetamine derivative whose pharmacologic effects are very similar to those of amphetamine, but is longer acting due to pharmacokinetic considerations. Methamphetamine is easily synthesized from over-the-counter products (eg, the α-adrenergic agonist, pseudoephedrine), and this has led to its increasing use as an abused drug. Unlike cocaine and amphetamine, methamphetamine is directly toxic at higher doses to midbrain dopamine neurons.
The opiates and their synthetic analogs are the most effective analgesic agents known (Chapter 11), yet they are widely abused because of their effects on brain reward circuitry 16–3. Morphine and heroin, along with a host of prescription opiates (eg, oxycontin), are among the most commonly abused opiates. Abuse of these drugs may be driven by a variety of factors, including their reinforcing effects, their ability to relieve both preexisting dysphoria and unpleasant symptoms related to drug withdrawal, and the intense craving they produce after long-term use. Physical dependence on opiates can occur independently of addiction; for example, patients with cancer pain may become physically dependent on these drugs but do not compulsively abuse them. Termination of opiate use is accompanied by emotional–motivational symptoms as well as somatic withdrawal symptoms 16–3.
Immediate effects of opiate drugs result from their binding to endogenous opioid receptors. As discussed in Chapter 7, the three types of opioid receptors—μ, δ, and κ—are distinguished by their pharmacologic profiles and anatomic distributions. These receptors belong to the G protein–coupled receptor superfamily and exhibit significant homology, particularly in transmembrane and intracellular regions. Opioid receptors couple with Gi/o proteins to inhibit adenylyl cyclase, to activate inwardly rectifying K+ channels, and to inhibit voltage-gated Ca2+ channels. They typically mediate inhibitory responses that involve a reduction in excitability and cell firing, and inhibition of neurotransmitter release. Examples of neural and behavioral actions mediated by μ, δ, and κ opioid receptors are listed in 16–4.
Receptor Subtype | Effect of Agonists | Effect of Antagonists | |
---|---|---|---|
Analgesia |
|
|
|
Respiratory function |
|
|
|
Gastrointestinal tract function |
|
|
|
Sedation |
|
|
|
Reward function |
|
|
|
Opiates activate brain reward circuitry via two main mechanisms: (1) disinhibition of the VTA, which results in dopamine release in the NAc, and (2) dopamine-independent activity in the NAc. For example, reinforcing effects of intravenous heroin can be partly attenuated by administration of an opioid receptor antagonist directly into the VTA or by lesions of VTA dopaminergic neurons. Opiate activation of such neurons results from opiate inhibition of GABAergic interneurons in the VTA that normally inhibit principal dopamine neurons 16–2.
Opiates also produce reinforcement through direct dopamine-independent actions on μ, and perhaps δ, receptors expressed in NAc neurons. These receptors are normally targets of the enkephalinergic (and possibly endorphinergic) neurons that innervate this brain region. Animals will work to self-administer opiates directly into the NAc, even in the presence of dopamine receptor blockade or 6-hydroxydopamine lesions of dopaminergic terminals in this region of the brain. Within the NAc, opiates directly inhibit some of the same populations of medium spiny projection neurons that are inhibited by dopamine. Thus, opioid and dopaminergic systems appear to converge on a common efferent reward pathway in the NAc 16–2.
In contrast to μ and δ opioid receptor subtypes, κ opioid receptor activation is not reinforcing; indeed, it is aversive. Activation of κ receptors decreases dopamine release in the NAc; thus, the mesolimbic dopamine system may mediate aversive effects of opiates as well as their reinforcing properties. See Chapter 11 for a discussion of the use of κ opioid agonists (eg, benzomorphan analgesics) in the treatment of pain. Tonic activation of the different opioid receptors in the reward circuitry by endogenous opioid peptides may modulate responses to natural reinforcers and influence an individual’s motivational state.
Ethanol is a CNS depressant that shares some behavioral effects with sedative–hypnotic drugs such as barbiturates and benzodiazepines (16–5; Chapters 5, 13, and 15). In humans it is clearly reinforcing and addictive, as evidenced by its widespread compulsive use. Ethanol reinforcement can be demonstrated in animals as well. The many serious health problems associated with long-term ethanol use, such as gastritis, cirrhosis, and malnutrition, most likely are related to the extremely large amounts of ethanol that are necessary for its psychoactive effects (as much as 100 mM in tolerant users), and also to the ability of this small molecule to interact with numerous physiologic systems.
Despite the high concentrations required for its psychoactive effects, ethanol exerts specific actions on the brain. The initial effects of ethanol result primarily from facilitation of GABAA receptors and inhibition of NMDA glutamate receptors. At higher doses, ethanol also inhibits the functioning of many other types of ion channels. Recent evidence supports the view that ethanol selectively affects these channels via direct actions at low-affinity binding sites.
Ethanol allosterically regulates the GABAA receptor to enhance GABA-activated Cl− flux. The anxiolytic and sedative effects of ethanol, as well as those of barbiturates and benzodiazepines, result from enhancement of GABAergic function. Facilitation of GABAA receptor function is also believed to contribute to the reinforcing effects of these drugs. Not all GABAA receptors are ethanol sensitive. As mentioned in Chapters 5 and 15, GABAA receptor complexes comprise combinations of five distinct subunit families. The regional distribution and relative abundance of these subunit combinations vary, and thus likely explain differences in the sensitivity of GABAA receptors to ethanol in different brain regions.
Ethanol also acts as an NMDA antagonist by allosterically inhibiting the passage of glutamate-activated Na+ and Ca2+ currents through the NMDA receptor. The sensitivity of NMDA receptors to ethanol, like that of GABAA receptors, may depend on receptor subunit composition. Other NMDA antagonists, such as phencyclidine (PCP) and ketamine, produce profound cognitive deficits and psychotic symptoms; thus, the dissociative and psychotomimetic effects of ethanol (at higher doses) may be mediated by means of such antagonism. Because other NMDA antagonists are reinforcing, some of ethanol’s addicting properties also are likely mediated by this mechanism.
The cellular mechanisms through which ethanol influences reinforcement systems is not yet known, but evidence suggests the involvement of several neurotransmitter systems (see 16–2). The reinforcing effects of ethanol are partly explained by its ability to activate mesolimbic dopamine circuitry, although it is not known whether this effect is mediated at the level of the VTA or NAc. It also is not known whether this activation of dopamine systems is caused primarily by facilitation of GABAA receptors or inhibition of NMDA receptors, or both. Ethanol reinforcement also is mediated in part by ethanol-induced release of endogenous opioid peptides within the mesolimbic dopamine system, although whether the VTA or NAc is the predominant site of such action is not yet known. Accordingly, the opioid receptor antagonist naltrexone reduces ethanol self-administration in animals and is used with modest effect to treat alcoholism in humans. Ethanol affects many other neurotransmitter systems in the brain, which may also contribute to its behavioral actions.
The best established genetic contribution to addiction is the protective effect that mutations in alcohol-metabolizing enzymes have on risk for alcoholism. Mutations that increase alcohol dehydrogenase (ADH) activity and decrease aldehyde dehydrogenase (ALDH) activity are additive and promote accumulation of acetaldehyde following ingestion of alcohol. This produces intoxication at low doses and a flushing reaction that is unpleasant, resembling the reaction to disulfiram, a drug used to prevent relapse (see below). These variants are common among people of East Asian descent, and individuals expressing these variants rarely abuse alcohol.
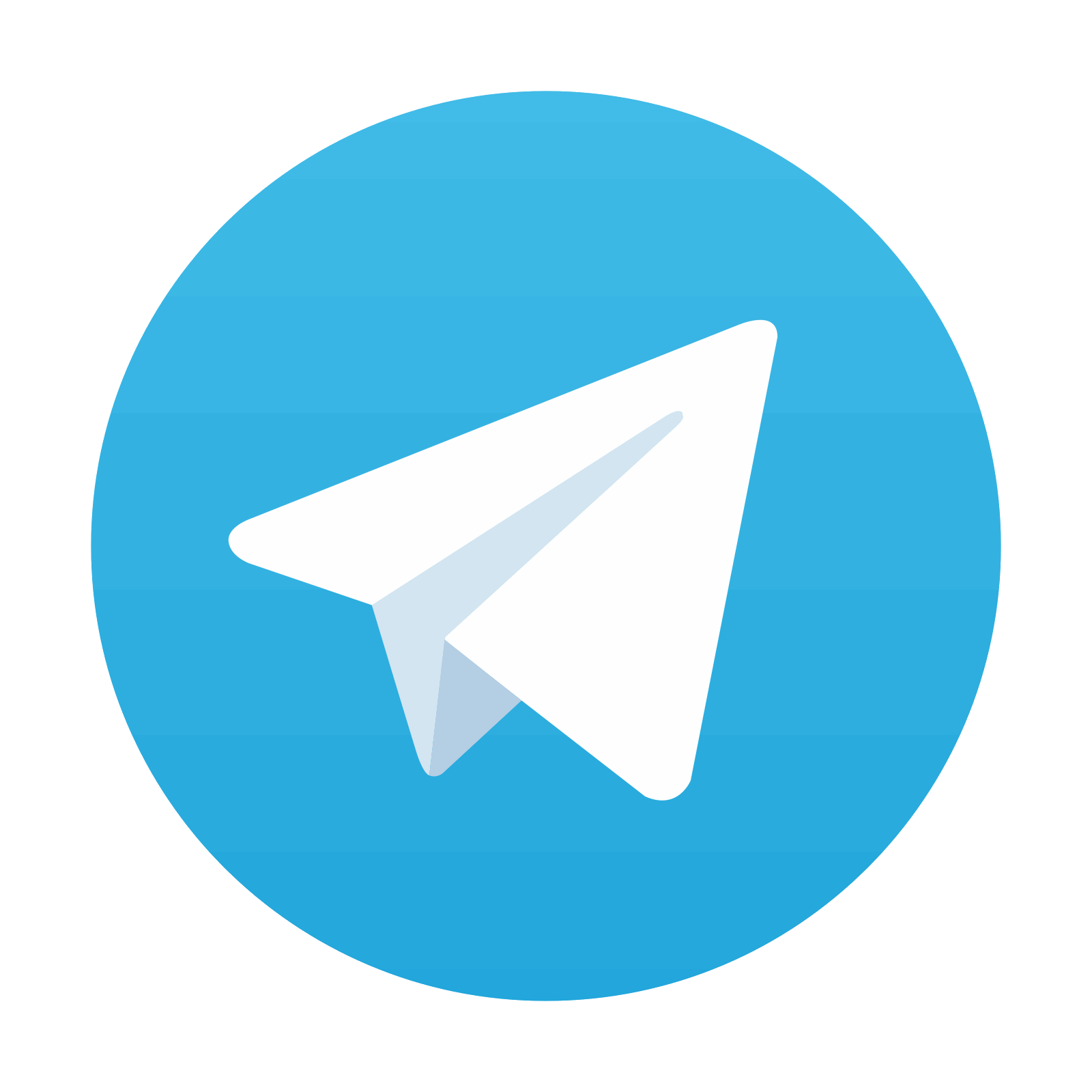
Stay updated, free articles. Join our Telegram channel

Full access? Get Clinical Tree
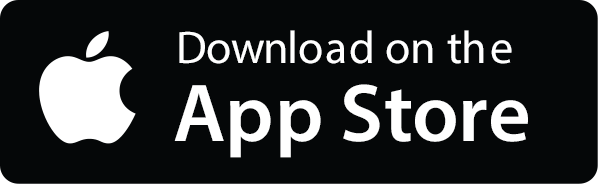
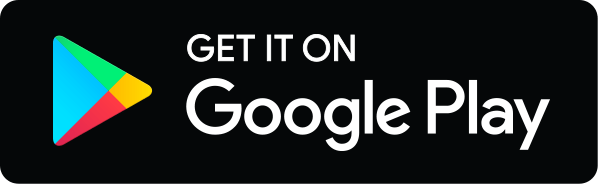