Fig. 1
Overview of potential mechanism through which chronically restricted and disrupted sleep may contribute to the development and maintenance of depression. Green arrow indicates a mild positive effect of restricted or disrupted sleep on neuroendocrine stress systems, which may result in elevated glucocorticoid levels. Red arrows indicate inhibitory effects on a variety of pathways, ultimately leading to a disturbance in connectivity and communication between brain regions involved in mood regulation (see text for details)
4.1 Stress and Glucocorticoids
Depression is often considered to be a disease of stress, not only because in many cases it is preceded by traumatic or stressful events, but also because the state of depression is frequently associated with an increased activity of neuroendocrine stress systems, particularly the hypothalamic–pituitary adrenal (HPA) axis . Various studies have described depression as a condition with HPA axis overactivity on the basis of elevated levels of hypothalamic corticotropin-releasing hormone and adrenal cortisol (e.g., Nemeroff et al. 1984; Gillespie and Nemeroff 2005).
Stress is also considered to be one of the major causes of sleep disturbance, and the latter may play an important role in the development of stress-related depression. In fact, even without external events triggering a state of stress, restricted or disrupted sleep may act as a stressor itself, both at the physiological and cognitive level.
At the physiological level, restricted or disrupted sleep may lead to a mild activation of the major neuroendocrine stress systems, including the HPA axis (for review, see Meerlo et al. 2008). Several controlled studies in humans have reported mild elevations of plasma cortisol levels associated with sleep deprivation (Chapotot et al. 2001; Leproult et al. 1997; Von Treuer et al. 1996). In most cases, the activation of the neuroendocrine systems during sleep deprivation is modest but may in subtle ways depend on the physical and mental activities subjects are engaged in (for review, see Meerlo et al. 2008). For instance, cortisol levels may rise during sleep deprivation when this is associated with sustained mental work (Radomski et al. 1992). The latter is highly relevant in real-life conditions, where people during sustained wakefulness often need to deal with all sorts of challenges.
At the cognitive level, insufficient sleep may dramatically alter the perception of the environment and the challenges that one is facing. Such challenges may then be perceived as more stressful than they would be under well-rested conditions. Indeed, a study in medical residents showed that sleep loss intensifies the negative emotional perception of disruptive and unforeseen events (Zohar et al. 2005). The latter has been confirmed in a controlled and experimental study showing that one night of sleep deprivation enhances negative emotional responses in a picture viewing task (Yoo et al. 2007).
Disrupted sleep may represent a state of stress particularly in cases where people have little control over their sleep, including sleep disorders such as insomnia. To many individuals that suffer from chronic sleep problems, the lack of sleep itself becomes a mental burden and a worrisome condition that may perhaps amplify the activation of neuroendocrine stress systems. This may be one factor contributing to the elevated levels of glucocorticoids reported in chronic insomniacs (Vgontaz et al. 2001).
The activation of the neuroendocrine stress systems as a consequence of restricted or disrupted sleep is often rather mild and may initially be considered a normal biological response that is aimed at supporting the metabolic demands of wakefulness. However, when disrupted sleep becomes a chronic condition, these same stress hormones may contribute to central nervous system changes that gradually sensitize individuals to depression (Meerlo et al. 2008). For example, chronically elevated levels of glucocorticoid stress hormones may gradually reduce serotonergic receptor expression and impair serotonergic signaling in the brain (Karten et al. 1999; Meijer and De Kloet 1994). Elevated levels of glucocorticoids have also been associated with a reduction in hippocampal neurogenesis and hippocampal volume (Lucassen et al. 2010). Such changes in serotonergic signaling and hippocampal integrity may ultimately contribute to a disturbance in cognitive function and mood regulation (see sections below and Fig. 1).
4.2 Attenuated Serotonergic Neurotransmission
The serotonergic system in the brain is involved in the regulation of mood, and it has long been thought that an impairment of serotonergic neurotransmission may underlay the development and maintenance of depression (for reviews, see Cryan and Leonard 2000; Sobczak et al. 2002; Stockmeier 2003). Numerous studies have indicated an attenuated serotonin-1A receptor-mediated signaling in depression, as shown by blunted physiological responses to serotonin-1A agonists (Lesch 1991; Mann et al. 1995; Shapira et al. 2000) and a reduction in 1A binding capacity in PET studies (Drevets et al. 1999; Hirvonen et al. 2007; Sargent et al. 2000). Although postmortem studies have yielded varying results, some of them are consistent with a decrease in serotonin -1A receptor function in depression (for review, see Stockmeier 2003). Finally, antidepressant medication is often based on drugs that enhance serotonergic neurotransmission (Blier and de Montigny 1994; Middlemiss et al. 2002). Given this evidence for a role of the serotonergic system in clinical depression, a gradual alteration in serotonergic signaling seems a candidate mechanism by which disrupted and restricted sleep might increase the risk for this disease. Importantly, in one PET study on patients suffering from major depression, the lower serotonin-1A receptor binding capacity showed a particularly strong association with the occurrence of insomnia, thereby underscoring the relationship between sleep disturbance, depression, and changes in serotonergic neurotransmission (Hirvonen et al. 2007).
Experimental studies in laboratory rats have shown that chronic sleep restriction reduces serotonin-1A sensitivity (Roman et al. 2005a, 2006; Novati et al. 2008). In response to an injection of a 1A receptor agonist, sleep-restricted rats had a blunted temperature response (Roman et al. 2005a) and a blunted pituitary ACTH response (Novati et al. 2008), similar to what has been reported for depressed patients (Lesch 1991; Shapira et al. 2000). The desensitization of the serotonin-1A receptor system gradually developed over time. One or two nights of restricted sleep had no clear effect yet, but after a week of sleep restriction the serotonin-1A responses in rats were significantly reduced (Roman et al. 2005a; Novati et al. 2008). Importantly, the desensitization of the 1A system persisted for many days even with unlimited recovery sleep. In fact, after a week of restricted sleep, complete normalization of serotonin-1A receptor sensitivity almost required a similar period of recovery (Roman et al. 2005a). Some effects of restricted sleep may thus be more persistent than is generally assumed.
As indicated in the previous section, a desensitization of the 1A receptor system as a consequence of insufficient sleep might be partly related to elevated glucocorticoid levels (Karten et al. 1999; Meijer and De Kloet 1994). However, in the rat studies, surgical removal of the adrenals, the main source of glucocorticoids, did not prevent the sleep restriction-induced desensitization of the 1A system, suggesting that other mechanisms are involved as well (Roman et al. 2006). The gradual desensitization of the serotonin-1A receptor system as a consequence of restricted sleep might in part be a consequence of serotonin itself, that is, a chronically enhanced serotonergic load on the receptors. Indeed, the release of serotonin is higher during wakefulness and sleep deprivation than it is during sleep (Park et al. 1999; Penalva et al. 2003) and continued or frequent stimulation of the receptors may gradually diminish their functional reactivity (Kreis and Lucki 1992; Li et al. 1999). Additionally, the desensitization of the serotonin-1A receptor population after chronic sleep restriction may be in part an indirect consequence of cross talk between this receptor system and other neurotransmitter systems such as the adenosine system. Adenosine is a metabolite of ATP, the main source of energy in the brain and body, and it is considered to be an important homeostatic molecule that signals neuronal activity and wakefulness (Basheer et al. 2004; Porkka-Heiskanen et al. 2002). Release of adenosine, and subsequent binding to its widespread G-protein-coupled A1 receptors, inhibits neuronal activity and promotes sleep, thereby protecting the brain against over activity. However, an increase in adenosine turnover under conditions of chronic sleep restriction and prolonged overstimulation of the receptors could result in desensitization, which might not only involve the receptors themselves but downstream elements of the signaling pathway as well. This could then affect the serotonin-1A receptor system because in various brain regions, adenosine A1 and serotonin-1A receptors are colocalized and share the G-proteins via which these receptors act on intracellular signaling cascades (Zgombick et al. 1989). A downregulation of the intracellular signaling pathways coupled to the adenosine A1 receptors might thus ultimately contribute to a reduction in serotonin-1A responses.
Reduced serotonergic signaling may be directly involved in disturbed mood (for reviews, see Cryan and Leonard 2000; Sobczak et al. 2002; Stockmeier 2003), but it may also impair plasticity processes, including neurotrophic signaling and neurogenesis, which in turn may affect neuronal circuitries involved in the regulation of mood (see next sections, Fig. 1).
4.3 Attenuated cAMP Signaling and Neuronal Plasticity
While depression was traditionally viewed as a neurochemical disorder, particularly a disorder of impaired and attenuated monoaminergic neurotransmission (i.e., serotonergic and noradrenergic signaling), this view has gradually shifted to depression being a disorder associated with altered neuronal plasticity and neuronal connectivity (Castren et al. 2007; Duman 2002; Licznerski and Duman 2013; Nestler et al. 2002). This modern view is supported by findings of structural changes in the brains of depressed patients, regional reductions in the number of synapses and neurons, and alterations in signaling pathways that are crucially involved in plasticity processes (discussed in detail below).
At the basis of such altered neuronal plasticity might be an impaired regulation of the intracellular second messenger cyclic adenosine monophosphate (cAMP) and/or one of its downstream targets (Duman et al. 1997, 2002 . Cyclic AMP activates protein kinase A (PKA) , which by means of phosphorylation controls the activity of various other downstream effector proteins. The latter includes phosphorylation and activation of the transcription factor cAMP response element-binding protein (CREB) that regulates the expression of a wide range of plasticity-related genes (Lonze and Ginty 2002).
Support for attenuated cAMP signaling and impaired regulation of neuronal plasticity in depression comes from analysis of postmortem brain samples of suicide victims showing reduced levels and activity of PKA and reduced levels of CREB in the prefrontal cortex and hippocampus (Dwivedi et al. 2003a, 2004). Moreover, antidepressant treatment stimulates components of the cAMP pathway. Particularly, expression of CREB was found to be increased in the hippocampus of depressed patients treated with antidepressant as compared to untreated patients (Dowlatshahi et al. 1998). Also in rodent models, treatment with various antidepressants was found to significantly increase mRNA and protein levels of CREB, phosphorylation of the CREB protein, and CREB-mediated gene transcription in several regions of the brain, including the cerebral cortex, hippocampus, and amygdala (e.g., Nibuya et al. 1996; Thome et al. 2000). This upregulation of CREB levels and activity only occurs with chronic, but not acute, treatment, in agreement with the slow onset of a therapeutic response in humans. Together, these findings suggest that at some level the regulation of the cAMP–PKA–CREB pathway may be impaired in depression and that reversal of this impairment may be a key in the treatment of the disorder (Blendy 2006; Duman et al. 1997; Duman 2002).
With respect to a potential role of insufficient sleep in this context, data on chronically restricted or disrupted sleep are limited, but consequences of acute sleep deprivation support a suppression of cAMP signaling in critical brain regions such as the hippocampus. Recent studies in mice have shown that brief sleep deprivation for only 5 h reduces cAMP levels in the hippocampus and thereby impairs plasticity processes and cognitive function (Vecsey et al. 2009; Havekes et al. 2014).
Such attenuated cAMP–PKA signaling as a consequence of sleep loss may directly affect phosphorylation and thereby the expression of glutamate receptor subunits at the cell membrane, which in turn determines neuronal excitability and the strength of neuronal connections. Indeed, studies in rats have shown that sleep deprivation for 6–12 h reduces hippocampal glutamate AMPA receptor phosphorylation (Hagewoud et al. 2010). A reduction in AMPA receptor phosphorylation and function has also been reported after multiple days of sleep disruption (Ravassard et al. 2009). Other studies have reported reduced hippocampal glutamate NMDA receptor surface expression after prolonged sleep deprivation of 24–72 h (Chen et al. 2006; McDermott et al. 2006). In agreement with these findings are several studies reporting that sleep deprivation impairs the induction of hippocampal long-term potentiation, which depends in part on glutamate receptors (e.g., Campbell et al. 2002; Chen et al. 2006; Marks and Wayner 2005; McDermot et al. 2006; Vescey et al. 2009).
Additionally, impaired cAMP–PKA signaling as a consequence of sleep loss may result in lower levels of CREB phosphorylation and activity, which is supported by studies in laboratory rodents showing that brief sleep deprivation for only 5–6 h can attenuate basal levels of CREB phosphorylation in the hippocampus (Vescey et al. 2009) and may also attenuate the increase in CREB phosphorylation normally associated with learning and memory processes (Alhaider et al. 2011, Hagewoud et al. 2011). While fairly brief sleep deprivation is capable of reducing phosphorylation and activity of CREB in the hippocampus, prolonged 48-h sleep deprivation or fragmentation was even found to reduce basal levels of CREB expression (Guzman-Marín et al. 2006).
Among the many genes, of which the expression is under control of CREB, are several genes that give rise to growth factors and neurotrophic factors, including brain-derived neurotrophic factor (BDNF) (Lonze and Ginty 2002). In rats, prolonged sleep deprivation or fragmentation was found to cause a reduction in BDNF mRNA and protein levels, in parallel to a reduction in CREB expression (Guzman-Marin et al. 2006). BDNF is an important promoter of neurogenesis , neuronal survival, and synaptic plasticity (Huang and Reichardt 2001; Park and Poo 2013). While reduced BDNF signaling in itself may not result in depression directly, it may impair plasticity processes and thereby alter connectivity within networks of brain regions involved in the regulation of mood (Castren et al. 2007; Nestler et al. 2002). In agreement with this are findings of reduced mRNA and/or protein levels of BDNF in the hippocampus and prefrontal cortex of suicide subjects (Dwivedi et al. 2003b). Moreover, antidepressant medication appears to restore BDNF levels to a normal range. Expression of BDNF was found to be increased in the hippocampus of depressed patients treated with antidepressant as compared to untreated patients (Dowlatshahi et al. 1998; Chen et al. 2001).
Importantly, BDNF often works in concert with serotonin to regulate gene expression and neuronal plasticity processes (Martinowich and Lu 2008; Mattson et al. 2004). Moreover, the BDNF and serotonin signaling systems mutually affect each other (Martinowich and Lu 2008). BDNF can stimulate the growth and plasticity of serotonergic neurons (Mamounas et al. 1995) and, conversely, enhanced serotonergic signaling through antidepressant medication promotes BDNF expression (Nibuya et al. 1996). These reciprocal interactions and the finding that both BDNF and serotonergic signaling may be impaired by prolonged sleep restriction add to the complexity of potential mechanism through which insufficient sleep may lead to impaired brain function and altered mood regulation.
4.4 Hippocampal Neurogenesis
It has been hypothesized that depression may in part result from a suppression of hippocampal neurogenesis and that successful antidepressant treatment requires an enhancement of neurogenesis (Eisch and Petrik 2012; Jacobs et al. 2000; Lucassen et al. 2010; Sahay and Hen 2007). One popular argument in favor of the neurogenic hypothesis is the common finding that depressed patients have a reduced hippocampal volume , which might in part be explained by lower levels of neurogenesis (see next section). Secondly, chronic treatment with antidepressants has been found to increase hippocampal cell proliferation in humans (Boldrini et al. 2012) and animals (Czéh et al. 2001; Malberg et al. 2000; Santarelli et al. 2003).
In recent years, various studies in laboratory rodents have examined how the production of new cells and their development into neurons is affected by sleep deprivation or sleep fragmentation (for review, see Meerlo et al. 2009). While interfering with sleep for a period shorter than a day appears to have little effect on the basal rate of cell proliferation , prolonged restriction or disruption of sleep may have cumulative effects leading to a major decrease in hippocampal cell proliferation, cell survival , and neurogenesis(e.g., Guzman-Marin et al. 2007; Mueller et al. 2008; Roman et al. 2005b; for review see Meerlo et al. 2009).
Hippocampal neurogenesis is regulated and affected by a wide variety of molecular factors, including trophic factors, cytokines, hormones, and a range of neuromodulators and neurotransmitters (Abrous et al. 2005; Ming and Song 2005). Some of these factors are also modulated by sleep deprivation or sleep disruption and may therefore provide a link between insufficient sleep and reduced neurogenesis (Fig. 1).
The effect of restricted or disrupted sleep on neurogenesis might be driven in part by stress hormones , particularly glucocorticoids , which have been found to suppress cell proliferation and survival (Wong and Herbert 2004, 2005). However, a number of controlled studies in rats showed that blocking glucocorticoid effects by means of adrenalectomy did not prevent the reduction in cell proliferation seen with 4–7 days of sleep fragmentation or partial sleep deprivation (Guzman-Marin et al. 2007; Mueller et al. 2008; for a critical discussion, see Meerlo et al. 2009). Alternatively, the effect of insufficient sleep on neurogenesis could be caused in part by reduced serotonergic signaling . The generation of new cells in the hippocampus is promoted by serotonin via the serotonin-1A receptor (Banasr et al. 2004; Radley and Jacobs 2002), and restricted sleep gradually desensitizes the 1A receptor system (Novati et al. 2008; Roman et al. 2005). The reduction in serotonin-1A sensitivity with sleep curtailment is not immediately evident but only develops in the course of prolonged sleep restriction, which is in agreement with the finding that suppression of hippocampal cell proliferation does not occur after short sleep deprivation but only after prolonged sleep deprivation. Moreover, also BDNF facilitates hippocampal cell proliferation and survival (Lee et al. 2002; Scharfman et al. 2005) and hippocampal expression of BDNF was found to be reduced after 48 h of sleep deprivation (Guzman-Marin et al. 2006).
Ultimately, the mechanisms by which chronically restricted or disrupted sleep reduces hippocampal neurogenesis may involve a complex and interacting set of factors, and some of these factors may selectively affect different stages of the neurogenic process, from cell proliferation to differentiation and maturation, to incorporation of new neurons in the existing hippocampal network. Because newly generated neurons are thought to play an important role in hippocampal function, and ultimately the regulation of cognitive function and emotion, a reduction of neurogenesis as a consequence of disrupted sleep may explain some of the symptoms of depression (Meerlo et al. 2009).
4.5 Structural Changes in the Brain
Several postmortem and imaging studies have reported structural changes in the brains of depressed patients, particularly reductions in the volume of the hippocampus (Campbell et al. 2004; Videbech and Ravnkilde 2004) and subregions of the prefrontal cortex (Rajkowska et al. 1999; Drevets 2000). In the prefrontal cortex, this reduction in volume is associated with a decrease in the size and density of both glia cells and neurons (Rajkowska et al. 1999), and a reduction in the number of synapses (Kang et al. 2012). Similar cellular changes may underlie the reduction in hippocampal volume , but this has not been unequivocally proven (Czeh and Lucassen 2007). Additionally, a smaller hippocampus might be partly the result of reduced neurogenesis (Czeh and Lucassen 2007; Lucassen et al. 2010). Both the hippocampus and prefrontal cortex are important parts of the brain network that regulates cognitive functions and mood, and the molecular or cellular changes underlying the volume reduction may explain the key symptoms of depression (Drevets 2000; Licznerski and Duman 2013).
Importantly, structural changes in the very same brain regions have also been reported in patients suffering from insomnia, i.e., reduced gray matter in prefrontal cortex subregions (Altena et al. 2010; Joo et al. 2013) and a smaller hippocampus (Neylan et al. 2010; Riemann et al. 2007; Joo et al. 2014). A number of studies suggest that, in particular, smaller hippocampi correlate with the severity or duration of insomnia (Neylan et al. 2010; Noh et al. 2012) and also with poorer cognitive function in a variety of tasks, including verbal and visual memory (e.g., Noh et al. 2012; Joo et al. 2014). Not all studies were able to replicate these findings, which might be due to the heterogeneity and age of the study population, as well as variation in insomnia duration and severity (Noh et al. 2012; Spiegelhalder et al. 2013).
A reduction in hippocampal volume has also been reported for other sleep disorders such as sleep apnea (Morrell et al. 2003) and narcolepsy (Joo et al. 2012). Moreover, in healthy children, a significant positive correlation was found between hippocampal volume and habitual sleep duration on weekdays (Taki et al. 2012).
While in humans it is difficult to assess whether subtle differences in brain structure are causally related to sleep duration or sleep disturbance, a recent experimental study in rats suggests this may be the case (Novati et al. 2011). Rats subjected to a schedule of sleep restriction allowing them only 4 h of sleep per day for a period of a month, as compared to the usual 10 h per day, had a 10 % decrease in hippocampal volume. This decrease could not be fully explained by a reduction in neurogenesis and may have been caused by other cellular changes, which might include neuronal shrinkage, dendritic atrophy , and loss of synapses as a result of impaired cAMP–PKA–CREB signaling and neurotrophic signaling discussed in previous sections. Additionally, a reduction in hippocampal volume might be related to reductions in the number and size of glia cells . Evidence is limited, but in one rat study proliferation of cells that were presumed to give rise to glia was reduced in response to sleep deprivation (Roman et al. 2005b), while another study suggested that proliferation of specific oligodendrocyte precursor cells was reduced (Bellesi et al. 2013).
Together, the findings presented in this section support the hypothesis that structural changes in the brain of depressed patients may at least be partly a result of chronically disrupted sleep.
5 Brain Region-Specific Consequences of Disrupted Sleep
Remarkably, the literature discussed in previous sections strongly suggests that among the brain regions that most frequently display structural and functional abnormalities in depressed patients are also the ones that appear to suffer most from inadequate sleep, particularly the prefrontal cortex and the hippocampus . This parallel may imply a general vulnerability of these brain regions to any kind of disturbance, but it also supports the view that disrupted sleep plays a causal role in the structural and functional changes that are seen in depression.
One obvious example of regional variation in sensitivity to the consequences of insufficient sleep is the reported suppression of neurogenesis (Meerlo et al. 2009), which in adulthood is largely restricted to the hippocampus (Abrous et al. 2005; Ming and Song 2005). Also other aspects of hippocampal plasticity and function appear to be more sensitive to sleep loss than is the case for some other brain regions. For example, neurotrophic signaling may be more severely affected in the hippocampus than in some other parts of the brain, as demonstrated by a study in rats reporting a suppression of BDNF after prolonged 48-h sleep disturbance in the hippocampus but not the neocortex (Guzmán-Marín et al. 2006). Moreover, multiple days of sleep disturbance prior to acquisition impairs subsequent hippocampus-dependent contextual fear conditioning but not amygdala-mediated tone-cued fear conditioning (McDermott et al. 2003; Ruskin et al. 2004). In humans, a single night of mild sleep disruption was found to attenuate hippocampal activation and subsequent memory in a hippocampus-dependent declarative task, while it had no effect on a hippocampus-independent memory task (Van der Werf et al. 2009). Together, these findings clearly demonstrate that insufficient sleep has a negative effect on plasticity processes and cognitive function particularly when it involves the hippocampus.
Because the consequences of disrupted sleep may vary between brain regions, this may alter the connectivity and communication between the regions that are most affected and the ones that are more resistant. Such may contribute to an unbalanced activity in brain networks involved in the regulation of mood and thereby ultimately contribute to mood disorders.
6 Conclusions and Future Perspectives
Depression is a complex disorder that most likely is the result of various interacting factors such as sensitive genes, developmental environment, lifestyle, and adult stress. Mounting data suggest that restricted or disrupted sleep may be an important causal factor as well. The mechanisms underlying such a relationship between insufficient sleep and depression may include activation of neuroendocrine stress systems and altered serotonergic signaling. Additionally, chronically insufficient sleep might contribute to depression through negative effects on signaling pathways involved in neuronal plasticity and neurogenesis. Clearly, as sleep is considered to play a crucial role in regulating neuronal plasticity and synaptic strength, chronically insufficient sleep may very well contribute to depression through a gradually developing impairment of the way neuronal networks in the brain are organized. The fact that, for reasons not understood, some brain regions are more sensitive to sleep loss than others may contribute to a disbalance in connectivity and communication between areas involved in mood regulation.
In recent years, major advances have been made in our understanding of the role of sleep in neuronal plasticity and the molecular consequences of acute sleep loss, which provide a framework for hypotheses on the mechanisms underlying the relationship between disrupted sleep in mood disorders . Yet, there is still a paucity of experimental data on the molecular and cellular consequences of insufficient sleep as a chronic condition, as it often occurs in real life. Many of the changes in plasticity processes that have been reported occur after fairly brief sleep deprivation and often normalize after subsequent recovery sleep. Future studies are required to assess, one, whether more chronically restricted or disrupted sleep has similar and perhaps more persistent effects on neuronal plasticity, and two, whether the consequent alterations in plasticity signaling contribute to changes in mood regulation.
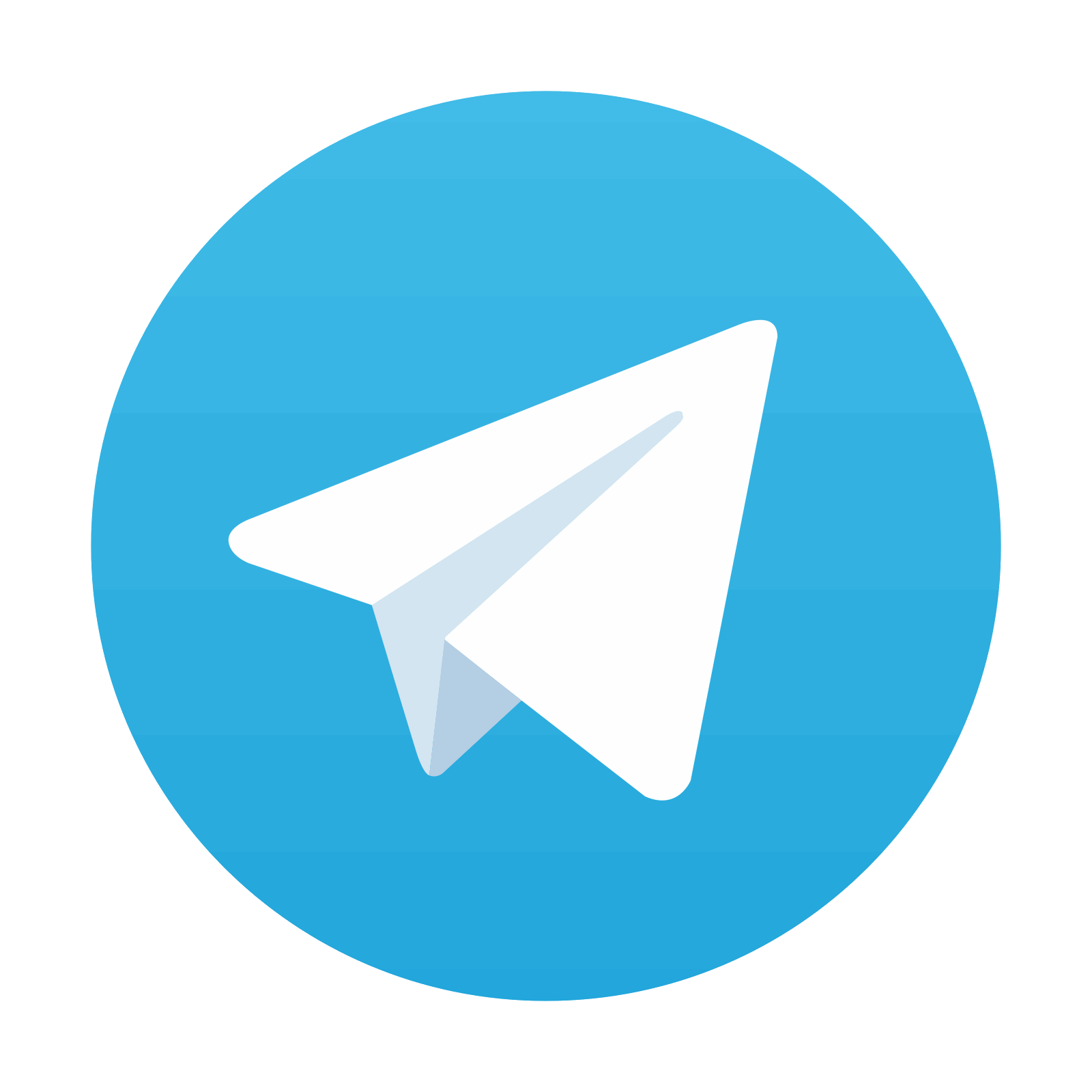
Stay updated, free articles. Join our Telegram channel

Full access? Get Clinical Tree
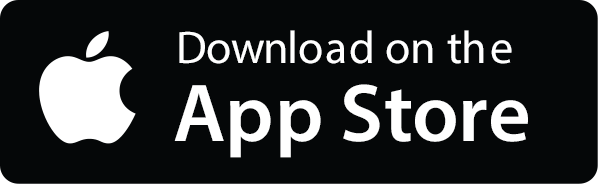
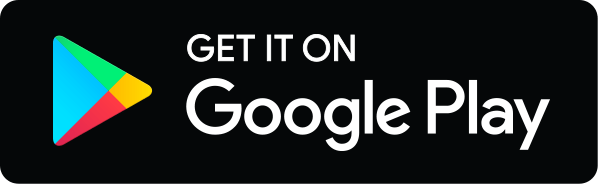