Intraoperative image-guided (IG) navigation and robotic assistance in spine surgery have traditionally been used to improve pedicle screw placement accuracy, decrease radiation exposure for operating room staff and patients, help surgeon ergonomics, and improve overall surgical outcomes. More recently, however, the application of these technologies has expanded to include the resection of spinal column and intradural tumors, evacuation of infection, revision arthrodesis surgery, and deformity surgery.
Intraoperative IG navigation systems provide real-time image guidance via reconstruction of images to three-dimensional (3D) spine representations in addition to navigation via an anatomic reference and surgical instruments with reference points. Multiple computed tomography (CT) based intraoperative IG navigation systems are in use today. For example, the StealthStation™ navigation (Medtronic©, Dublin, Ireland), which is a 360-degree CT scanner, utilizes intraoperative CT, a spinous process or iliac crest reference clamp, and surgical instruments with reference points to allow for surgeon-controlled navigated drilling, tapping, and screw placement during surgery. Another system is the Airo® Mobile Intraoperative CT platform (Brainlab©, Munich, Germany), which is similar to the StealthStation with the exception of using a 360-degree CT scanner attached to the OR table. A robust body of literature has established the advantages of 3D navigation in spine surgery including improved screw placement accuracy. New alternative IG systems include the 7D Surgical System (7D Surgical©, Toronto, Canada), which utilizes camera-based imaging to register bony anatomy through machine vision technology. Such systems reduce radiation exposure by circumventing the need for CT or fluoroscopy intraoperatively and may can help streamline intraoperative workflow, although further studies are needed to evaluate this new technology.
Robotic-assisted spine surgery goes beyond intraoperative IG. It not only utilizes imaging for trajectory planning by the surgeon, but also provides an automated robotic arm that manipulates surgical instruments. To date, the FDA has approved three robotic systems for spine surgery: Mazor X™ (and its prior iterations Renaissance™ and Spine Assist™; Mazor Robotics©, Caesarea, Israel), ROSA® robot (Medtech Surgical©, Montpellier, France), and ExcelsiusGPS™ (Globus Medical©, Audubon, PA, USA). The Mazor X™ robot attaches to the OR table and has a surgical arm that moves to a predetermined trajectory, a camera with 3D spatial tracking, and a computer program that allows for preoperative screw trajectory planning and intraoperative trajectory verification. Several studies have shown greater accuracy in pedicle screw placement using Mazor Rotobitcs© compared to conventional fluoroscopy-assisted free-hand techniques. The ROSA® robot uses intraoperative planning and navigation to determine the surgical arm trajectory. Its camera system tracks a reference clamped to the patient and a reference attached to the robot; the tracking feedback is used by the robot to adjust its position if the patient moves. A retrospective study has shown improved accuracy of screw placement with ROSA® compared to the free-hand technique. Lastly, the ExcelsiusGPS™ provides intraoperative imaging, trajectory planning, and screw placement through a rigid external arm that allows for navigated drilling and navigated interbody cage placement without the use of K-wires. The robot does not attach to the OR table or patient and tracks its dynamic reference base with a second surveillance marker for movements. Several studies have shown accurate percutaneous and open pedicle screw placement with the ExcelsiusGPS™. In a recent meta-analysis of pedicle screw accuracy for all methods (robotic-assisted, CT-based navigation, fluoroscopy-assisted, and free-hand), CT-based navigation had the overall highest accuracy rate, although in the thoracic spine, robotic assistance showed the highest accuracy.
While the short-term benefits of robotic-assisted spine surgery have been well established, its definitive long-term clinical advantages have not yet been proven. Two recent randomized controlled trials evaluated the long-term (1- and 2-year) outcomes after robotic-assisted pedicle screw fixation with the Renaissance™ system versus the conventional free-hand technique. The patients reported similar quality of life measures across the groups. In one study, two patients in the free-hand group required revision surgery while none in the robotic group did. In the other study, the free-hand group had more adjacent segment degeneration. The long-term outcomes using robotic surgery need further investigation.
As work evaluating the new generation of robotics continues to unfold, a combined approach integrating navigation and robotic assistance may prove to be superior to the current standard of care. While the overwhelming majority of work done with robotics has focused on facilitating screw placement, the future may in fact see robots providing a much larger role in spine procedures. Here, we present two cases demonstrating the potential use of robotics for novel methods of screw placement and navigated assistance for corpectomy and interbody cage placement.
Case 1: Transvertebral Screw Placement
Here, we present our experience performing a robotic-assisted L4–S1 fusion for grade 2 spondylolisthesis. In this case, we demonstrate how the use of navigated-robotics technology allows us to place screws in an entirely novel trajectory (transvertebral, instead of through the pedicle) that would not otherwise be feasible without navigation or robotic guidance.
History and Clinical Presentation
A 66-year-old woman with a prior history of hypertension, anxiety, and sleep apnea presented with one year of severe low back pain radiating to the right lower extremity and bilateral lower extremity numbness. The patient was neurologically intact on examination.
Preoperative Imaging
The preoperative lumbar spine MR imaging demonstrated grade 2 L5/S1 spondylolisthesis with severe central and foraminal stenosis ( Fig. 10.1 ).

Surgical Technique Including Intraoperative Imaging
The patient underwent an L4–S1 robotic-assisted lumbar decompression and fusion using the ExcelsiusGPS robot. After standard prone positioning on the Jackson table and sterile preparation and draping, the ExcelsiusGPS™ Dynamic Reference Base (DRB) and surveillance markers were placed on the posterior superior iliac spines. The intraoperative CT (iCT) fixture was then attached, and the O-arm was brought into use. A 3D CT scan was rendered and sent to the robotic system for screw trajectory planning.
Due to the patient’s steep lumbosacral angulation and pedicle anatomy, we found that the trajectories of the L5 and S1 pedicles in this patient would overlap and the screw heads would collide. We therefore used our navigation technology to plan a more favorable transvertebral screw trajectory from the S1 vertebral body into the L5 vertebral body and crossing the disk space. This was planned bilaterally for the L5/S1 screws. We then removed the iCT fixture, exposed the spine in the routine manner, and used the ExcelsiusGPS™ robotic arm to place these screws in an open, robotic- or navigation-assisted fashion. The standard L4 pedicle screws were also placed using the ExcelsiusGPS™ robot ( Fig. 10.2 ).

After confirming good hardware placement, a routine lumbar decompression, including full laminectomy and bilateral foraminotomies, was performed. Rods were placed, the transverse processes and lamina were decorticated, and demineralized bone matrix was placed to promote fusion. A routine, multi-layered closure was performed.
Postoperative Imaging
The postoperative lumbar radiographs demonstrated the L4–S1 fusion construct with the L5/S1 transvertebral screws in excellent position ( Fig. 10.3 ).

Postoperative Recovery and Follow-up
At the two-month postoperative follow-up, the patient’s lower back and right lower extremity pain had resolved. She regained full strength in all muscle groups and ambulated independently without the use of an assistive device.
Procedural Concerns in Detail (Whether Navigation, Robotics, or Both)
Transvertebral screw placement is typically not performed via a free-hand technique due to a paucity of anatomic landmarks. There is particular concern regarding the possibility of entering the foramen and causing injury to the nerve root via a free-hand technique. However, with the real-time image guidance and preoperative planning provided by ExcelsiusGPS™, this procedure can be performed with a greatly reduced risk of complications. The advantages of the transvertebral screw technique include tri-cortical purchase and placement of a much longer screw than the routine lumbar pedicle screw trajectory.
Treatment Side Effects
This case was one of the first performed with the ExcelsiusGPS™ robot. At this stage in our robotics learning curve, we were performing O-arm spins intraoperatively, instead of preoperatively with intraoperative fluoroscopy. We were also obtaining O-arm spins after the screw placement to confirm screw accuracy and for research purposes. As a result, this case provided 31.13 Gy of exposure for the patient. For his robotics cases using the ExcelsiusGPS™ robot, senior author N.T. is now routinely doing a preoperative CT scan, with intraoperative fluoroscopy and no confirmatory O-arm spin, which results in significantly less exposure to patients on average.
Potential Downfalls
Although the robotic system allowed us to place a transvertebral screw in a unique trajectory, we did not reduce the patient’s grade 2 spondylolisthesis. We felt that we could obtain an adequate decompression of the nerve roots via a full laminectomy and bilateral foraminotomies in order to address the patient’s radicular symptoms. Moreover, the patient’s overall alignment was reasonable in terms of lumbar lordosis (LL), pelvic incidence (PI), and LL-PI mismatch, so we did not feel that a reduction was essential to improve the overall spinal global alignment.
In addition to the well-established risks of decompression and fusion lumbosacral surgery, cases involving decompression and fusion with or without the reduction of high-grade spondylolisthesis are especially technically challenging. These are associated with motor and sensory deficits. The incidence of neurologic deficit following distraction for reduction high-grade spondylolisthesis is reportedly from 10% to 31%, with the majority being transient.
Management of Complications
In cases of lower-grade spondylolisthesis without sagittal imbalance, one may consider not performing distraction for reduction purposes. It should be noted that if a reduction is pursued for the correction of spondylolisthesis, it is generally recommended to use interbody implants and bone grafting to reduce the risk of hardware failure and nonunion. Additionally, a study using cadaver models suggested that risk of stretch injury to the nerve root is higher in complete reduction compared to a partial reduction of high-grade spondylolisthesis. Thus, if a reduction is necessary, the surgeon should consider doing so partially.
Case 2: Navigated Corpectomy and Screw Placement
Here, we present our experience performing an intraoperative image-guided L2 corpectomy and T11–L4 posterior spinal fusion for metastatic cancer. The authors contend that future updates to spine robotic technology will enable us to perform these techniques in an even safer manner by incorporating robotics with the current navigation techniques.
Case Illustration
History and Clinical Presentation
A 62-year-old woman with multiple medical comorbidities including congestive heart failure, recent thymic cancer (status post-chemotherapy and post-radiation), and a remote history of colon cancer presented to the hospital with a 5-month history of severe lower back pain, preventing her from being able to walk for the past month.
Preoperative Imaging
Magnetic resonance imaging (MRI) revealed a pathologic fracture at L2 with enhancing tumor involving the L1 and L2 vertebral bodies and causing severe spinal cord compression at L2 ( Fig. 10.4A and B ). CT scans demonstrated the bony anatomy of the L1/L2 pathologic fracture with retropulsion into the canal ( Fig. 10.4C ). Given the spinal instability and severe cord compression, significant pain, and only moderate radiosensitivity of the presumed metastatic thymic cancer, we elected to perform a spinal decompression and stabilization with significant tumor debulking/resection.

Surgical Technique Including Intraoperative Imaging
After standard prone positioning on a Jackson table, sterile preparation, and draping, fluoroscopy was used to identify the T10 to L4 levels. A routine exposure and sub-periosteal dissection to expose the spinous processes, lamina, and transverse processes of T10 to L4 were completed. The StealthStation™ navigation tracker was placed on the spinous process of T10 and the O-arm was used to obtain a 3D CT scan. The CT data was transferred to the StealthStation™ for anatomical accuracy, registration, and pedicle trajectory planning to enable real-time image guidance during instrumentation. The pedicles were then cannulated bilaterally at T11, T12, L3, and L4. Initially, the high-speed drill was used to drill a pilot hole before using the navigated drill guide, navigated tap, and navigated screw inserter sequentially to place the appropriately sized screws at each level ( Fig. 10.5A and B ).

The extracavitary posterolateral approach to the L2 corpectomy was accomplished using full L1 and L2 laminectomies and medial facetectomies. We began the corpectomy via the left side, using the Leksell and navigated drill to take down the facet joints, L2 transverse process, and L2 pedicle to skeletonize the left L1 and L2 nerve roots. The navigated pointer was used to indicate the superior and inferior endplate ( Fig. 10.5C and D ) for the corpectomy, and to determine the ventral extent ( Fig. 10.5E and F ). Residual tumor remained within the left L2 pedicle, which was soft. The majority of the tumor was found to be located ventrally. Using a combination of navigated drills, curettes, and pituitary rongeurs, >50% of the L2 vertebral body was removed. The soft tissue specimen was sampled and pathology was consistent with metastatic thymic cancer.
We then used the navigated drill to take down the right L2 pedicle, facet joints, and transverse process to expose the right-sided L1 and L2 nerve roots. The spinal cord was fully decompressed ventrally bilaterally using the Woodsen, curettes, and pituitary rongeurs. The Woodsen was used to ensure there was no further anterior compression on the spinal cord. Stealth navigation was used to confirm that we had reached the anterior border of the vertebral body. The bilateral L1 and L2 nerve roots were well preserved and there were no changes in motor or sensory innervation.
A sizer was used to place an expandable titanium cage, filled with demineralized bone matrix, in the space of the corpectomy. Fluoroscopy was used to ensure good placement on both the anteroposterior and lateral x-rays. Appropriately sized titanium rods were inserted into position on the screw heads on the left and right sides. The set screws were placed and locked on both sides, and we compressed across T12 to L3 to secure the corpectomy cage in place. The set screws were then tightened using appropriate torque. The wound was closed in the usual fashion with a surgical drain in place, and the patient was transferred back to the intensive care unit in a stable condition.
Postoperative Imaging
Postoperative x-rays demonstrated good hardware placement ( Fig. 10.6 ).

Postoperative Recovery and Follow-up
The patient returned to the ICU after surgery, given her multiple medical comorbidities. She remained neurologically intact. Her postoperative course was notable for subsegmental pulmonary emboli requiring therapeutic lovenox, and she was transferred to an inpatient rehab facility 1 week after surgery. Within 5 days after surgery, she was able to stand out of bed, which she had not been able to do for a month before surgery.
Procedural Concerns in Detail (Whether Navigation, Robotics, or Both)
A major concern in performing corpectomies is properly identifying the anatomic landmarks which can be obscured in the setting of erosive, invasive tumor. First, the superior and inferior endplates should be identified so that the entire disks are resected in order to provide a good endplate upon which the corpectomy cage sits. The goal of the appropriate endplate preparation is to facilitate a solid fusion across the corpectomy site.
In addition, the nerve roots should be carefully identified and preserved, especially in the lumbar spine, where nerve roots are functionally important and cannot be sacrificed without a resulting motor deficit. Finally, it is important to identify the ventral aspect of the vertebral body, so that arterial and venous structures are not violated. At the L1/L2 level, the aorta is located ventrolateral slightly to the left side of the vertebral body and disk while the inferior vena cava is ventrolateral slightly to the right side. Farther down, the aortic bifurcation is at the L4 vertebra in a majority of patients, with possible superior migration when the patient is in the prone position. The iliocaval junction if often at the L4/L5 disk or L5 vertebra; however, this too can migrate superiorly in the prone position. The goal is to complete the corpectomy as far ventrally as possible so that the cage sits anteriorly, while avoiding a potentially catastrophic vascular injury.
Intraoperative navigation provides a safe and effective way to localize these anatomical landmarks to allow ideal corpectomy and cage placement. Future systems that incorporate intraoperative navigation with robotic surgery may allow for the fusion of preoperative CT scans and MR scans with angiography to establish “no-fly” zones that prevent the guided drill from going too far anteriorly and prevent it from violating the anterior vasculature or nerve roots bilaterally.
In addition, the future version of the navigated spine robotics may also establish a “no-fly” zone around the dura to help prevent a cerebrospinal fluid (CSF) leak. Perioperative CSF leaks are a known complication associated with spine surgery. Indeed, spine surgery carries a significant rate for unintended durotomies and CSF leaks, with an incidence of roughly 0.3% to 35% in the literature. A recent 10-year systematic review by Ghobrial et al. (2015) reported an overall 8.11% durotomy rate (range, 2%–20%) in patients undergoing lumbar degenerative spine surgery. Additionally, patients undergoing surgery for spine tumors may be at increased risk of CSF leaks due to the presence of an intradural tumor requiring durotomy and reduced healing secondary to poor nutritional status and adjuvant chemotherapy and radiation. Effective protocols for preventing and treating such leakage are essential to avoiding downstream adverse outcomes, including wound infection, intracranial hemorrhage, and meningitis, among others. Importantly, tumor seeding is a feared complication of postoperative CSF leak unique to patients undergoing resection of malignant spinal tumors.
Treatment Side Effects
For patients with metastatic spinal cancer undergoing surgical intervention, pseudoarthrosis can be a painful and highly morbid complication. Postoperative radiation and chemotherapy, common in this patient population, are risk factors for a failure to fuse, or nonunion, resulting in hardware failure. Thus, cancer patients require unique considerations when undergoing fusion surgery. First, a meticulous endplate preparation should be performed at the inferior and superior endplates so that the corpectomy cage has a good bony substance to oppose. In addition, the surgeon should carefully consider the corpectomy cage material. In particular, a bony allograft (such as cadaveric fibula) or titanium expandable cage should be considered rather than polyetheretherketone (PEEK) cages. Multiple studies of mid- to low-level evidence have evaluated whether PEEK or titanium is the superior cage and have overall concluded similar fusion rates. In a study of one-level anterior cervical discectomy and fusion, PEEK was found to have rates of pseudoarthrosis nearly five times as high as structural allograft, and twice as many revision surgeries. We prefer to use titanium cages in patients with cancer due the availability of expandable configurations which offer superior fit and load-bearing, increased cell adhesion characteristics and improved osseointegration.
Finally, careful attention must be paid to the material that is used to fill the corpectomy cage. Recombinant human bone morphogenetic protein (BMP)-2, which is being used increasingly in spinal fusion and especially in adult spinal deformity surgery (up to 61% according to studies using the ISSG dataset), may encourage tumor growth and is relatively contraindicated in patients with active cancer. Instead, various bone allografts, including cadaveric cancellous bone chips, and/or demineralized bone matrix (DBM), can be considered to effect an arthrodesis at the corpectomy site; the combination of allograft with DBM has been shown to have similar fusion integrity to that of iliac crest autograft.
Potential Downfalls
As discussed in the preceding sections, the potential downfalls of this procedure include vascular injury with an anterior breach or nerve root damage. A potential long-term complication is nonunion resulting in hardware failure, which is more common in cancer patients whose multiple rounds of chemotherapy and radiation collectively undermine fusion.
Management of Complications
As previously mentioned, CSF leaks are a known complication of these surgeries. The most important aspect of treating CSF leak is prevention, including preoperative risk factor evaluation and excellent intraoperative technique and manipulation. For example, residual bone spikes may result in postoperative puncture of the dural sac. Primary repair of durotomies intraoperatively is the preferred means to prevent postoperative CSF leaks; however, this method is reported to have a failure rate of 5% to 9%. A variety of dural repair techniques and materials may be used, including direct suturing, use of dural patch graft materials, and tissue sealants. The authors’ preferred method is primary repair with 5-0 prolene when possible, followed by Tisseel sealant. A small local muscle patch can also be used if necessary. A lumbar drain may be placed for several days for flow diversion; however, this should be avoided in patients with cancer due to risk of tumor seeding.
Summary and Future Direction
Current robotic and image-guided systems facilitate increased accuracy, precision, and consistency of pedicle screw placement in spine surgery. Such systems also provide systemic value by reducing radiation exposure to the surgical team, potentially streamlining the operative workflow, enabling minimally invasive applications, and improving patient outcomes in select cases.
Especially when first implemented, navigation and robotic systems can worsen workflow, due to the high learning curve, and may result in increased patient morbidity during this training period. Ultimately, the onus of learning how to safely and effectively use robotic and navigation technology lies with the surgeon. All companies provide hands-on training sessions for surgeons and provide significant personnel, particularly at the beginning, to assist with cases. In addition, a major barrier to widespread robotics adoption is cost, with some robots costing over $1 million per unit. Moreover, intraoperative robotics and navigation use require restructuring and changing the OR workflow, and training all OR staff including the nurses, scrub technicians, and radiology technologists.
As the use of navigation and robotics in spine surgery expands, these systems’ value in improving surgical accuracy must be considered against concerns over cost and workflow. Given the relatively recent adoption of robotics in spine surgery, studies that definitively establish the long-term benefits of such systems on clinical outcomes remain scarce. However, preliminary results are promising. As demonstrated in systematic reviews and a recent meta-analysis, robotic-assisted screw placement has been shown to have more consistent accuracy compared with traditional free-hand or fluoroscopy guided approaches. The additional benefits include reduction of radiation exposure. Further clinical and cost-related benefits likely correlate with the experience of the surgeon and the institution using robotic technology. Experienced groups have shown cost savings in the forms of reduced length of stay, decreased need for revision surgery, lower infection rates, and shorter operative times.
The two cases presented in this chapter demonstrate what we envision for the current and next generations of spine robots. These robots do not just allow us to place pedicle screws, a procedure which many surgeons feel comfortable performing free-hand without robotic or navigation assistance and which may contribute to the low adoption rates of such systems. The next generation of navigation and robotic assistance may enable us to perform surgeries that we would otherwise be unable to do, including placing screws in different trajectories that do not have good anatomic landmarks, such as the transvertebral screw. The ability to visualize the screw trajectory in multiple dimensions is important when placing transvertebral screws as the pedicle can be narrow in the axial plane and the screw must pass diagonally through the pedicle in the sagittal plane, allowing purchase of the superior vertebral body across the disk space. In our corpectomy case, we used both a navigated drill and a pointer to indicate the superior and inferior endplates for the procedure, and also to determine the ventral extent of the corpectomy. Such navigation can greatly minimize morbidity by providing surgeons with a real-time localization that helps avoid dangerous anatomy. It is easy to envision using these navigation tools to perform various types of osteotomies in spinal deformity surgery, in addition to the navigated tumor corpectomy described here.
Next-generation systems will combine the best of both robotic and navigation technologies. They will allow us to place navigated transforaminal lumbar interbody fusion (TLIF) or corpectomy cages. By fusing preoperative MRI and CT scans, the robots will generate “no-fly” zones for corpectomies or even simple TLIF cage placement, preventing the robotic arm (and hence the drill or other tool) from damaging the nerve roots, dura, or vasculature. Navigation systems can also be used to assist in planning osteotomies by modeling the resection plane and expected spinal correction. The improved precision offered by these systems could be integrated with robotics systems to further improve procedure accuracy. Pre-planning software, which is already being used with most robotics technologies, will continue to evolve and allow us to select screw trajectories, screw lengths, rod configurations and cage sizes preoperatively to decrease the amount of sterilized hardware needed for a case and improve workflow. Additionally, improvements in engineering technology will yield fluidity of movement in robots, resulting in increasing accuracy and efficiency during mechanical tasks. This will address surgeon fatigue and minimize impact of tremor. Additionally, next-generation robotic technologies will incorporate augmented reality (AR), technology that allows visual data is overlaid onto the surgical field for direct and immediate interaction. While AR has become transformative in the world of video gaming, it has yet to gain a solid footing in the realm of surgery. While these technologies are used in surgical training and anatomical study, its applicability has only recently been evaluated in spine surgery. This type of technology, when coupled to a robotic platform, has the potential to give the surgeon a much less distracting work environment and may allow them to “see through” the patient during complex percutaneous and minimally invasive procedures that will be done with robotic assistance.
Robotic spine surgery will continue to evolve, and the forefront is the application of artificial intelligence (AI) and machine learning (ML). In brief, AI is a discipline of computer science that simulates intelligent behavior in computers, while ML is a domain of AI that facilitates automatic analytical model building; that is, allowing the computer to learn patterns by studying data directly and subsequently to predict patterns of interest. Currently, AI and ML are used in diagnosis, prognosis, and outcome prediction in medicine. In the future, ML-based modeling will predict surgical candidacy by using preoperative patient demographics, comorbidities, and imaging characteristics. The era of robotic surgery, which will allow the surgeon to plan and rehearse surgery, will also benefit from complex ML algorithms that will help the operator by automatically planning best fit and alignment of screws which can be adjusted prior to surgery. Eventually, by gathering outcomes from hundreds and eventually thousands of planned surgical cases, these models will be able to determine the best procedure to help a given patient, to plan the surgery in advance (including optimal fusion levels, ideal screw placement, etc.), and to guide the surgeon in performing the surgery safely.
Although the future of robotic surgery is exciting, AI-based robots are unlikely to replace the human surgeon. However, the next generation of spine surgeons will be expected to adapt to and work side-by-side with evolving navigation and robotics technologies to improve surgical efficiency and patient outcomes.
References
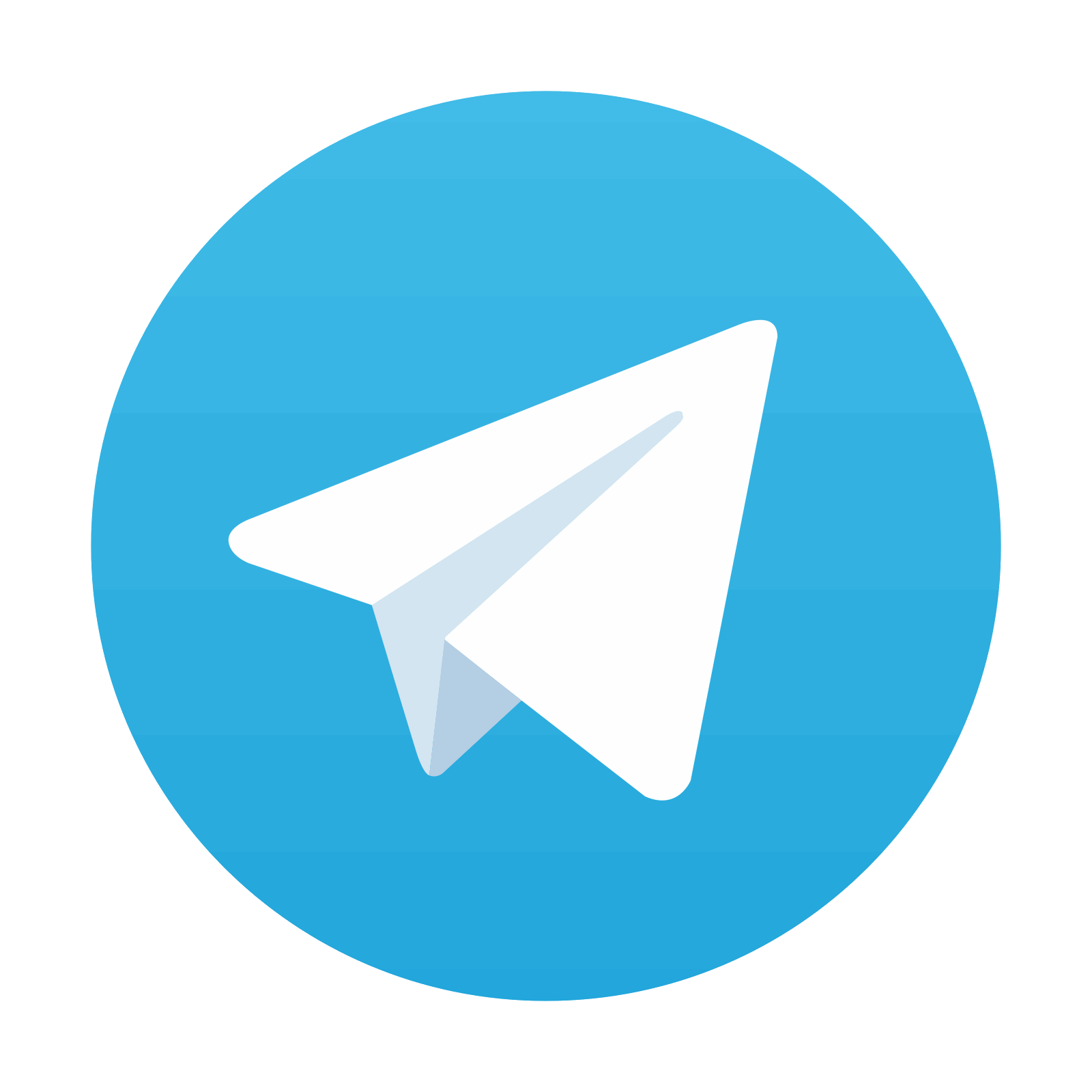
Stay updated, free articles. Join our Telegram channel

Full access? Get Clinical Tree
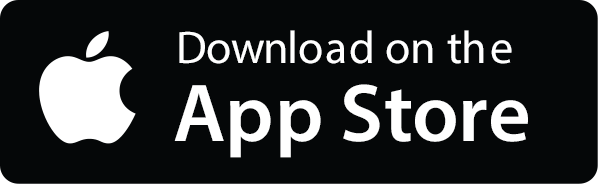
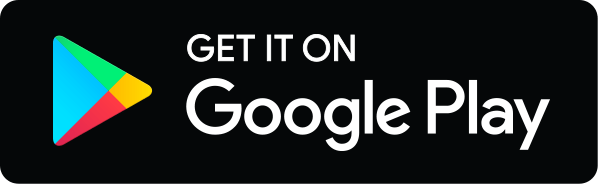
