Fig. 1
Innervation of the nucleus accumbens. This figure highlights the principal pathways that innervate and project from the nucleus accumbens core and shell. NACs nucleus accumbens shell; NAcC nucleus accumbens core; VTA ventral tegmental area; dPFC dorsal prefrontal cortex; cPFC ventral prefrontal cortex; VP ventral pallidum; HIPP hippocampus; PPTg pedunculopontine tegmental nuclei; LTg lateral tegmental nuclei. The figure is adapted from Vanderschuren and Kalivas (2000)
2 The Effects of Nicotine on DA Release from Mesolimbic DA Neurons In Vivo
There is considerable evidence from electrophysiological and microdialysis studies that nicotine falls into the category of addictive drugs which stimulate DA release in the nucleus accumbens. Studies in the mid-1980 showed that injections of nicotine stimulate midbrain DA neurons (Grenhoff et al. 1986). Imperato et al. (1986) were among the first to report that nicotine injections stimulate DA overflow in the nucleus accumbens when measured using microdialysis. In the years following, other studies confirmed these initial observations (Benwell and Balfour 1992; Brazell et al. 1990; Damsma et al. 1989; Mereu et al. 1987; Nisell et al. 1994b; Schilstrom et al. 2003). The nucleus accumbens is composed of two principal subdivisions, the accumbal shell and the accumbal core, which are anatomically distinct, project to different areas of the brain and are thought to subserve different physiological and behavioral functions (Heimer et al. 1991; Zahm and Brog 1992). Acute injections of drugs of dependence elicit greater increases in DA overflow in the shell subdivision of the accumbens that the accumbal core (Di Chiara and Bassareo 2007). The acute administration of nicotine to drug-naïve animals also results in a preferential increase in DA overflow in the accumbal shell (Cadoni and Di Chiara 2000). Thus, the effects of acute nicotine on DA overflow in the nucleus accumbens closely resemble those observed for other drugs of dependence (Balfour 2004; Di Chiara 2000; Pontieri et al. 1996).
The DA neurons which project from the VTA express neuronal nicotinic receptors on both their somatodendric membranes and the membranes of the nerve terminals and peri-terminal regions of the axons (Livingstone and Wonnacott 2009). The molecular composition of these receptors is diverse and is considered in some detail by Fasoli and Gotti (see chapter entitled Structure of Neuronal Nicotinic Receptors; this volume). However, there is compelling evidence that the effects of systemic nicotine administration to rats depend upon the stimulation of one or more of these receptor populations (Livingstone and Wonnacott 2009). It is also important to remember that a number of the neuronal pathways which project to the VTA or the nucleus accumbens and influence the activity of DA neurons also express neuronal nicotinic receptors, and it is widely accepted that stimulation of these receptors also contributes significantly to the effects of systemic nicotine on DA release in the terminal fields of the mesolimbic system (Markou 2008). The effects of nicotine on DA overflow in the nucleus accumbens are attenuated by the prior administration of nicotinic receptor antagonists that penetrate into the brain (Benwell et al. 1995). Furthermore, the local administration of a nicotinic receptor antagonist into the VTA, but not the nucleus accumbens, also attenuates the increase in DA overflow evoked by a systemic injection of nicotine, results which support the conclusion that the changes in DA overflow evoked by nicotine depend upon the stimulation of nicotinic receptors in the VTA resulting in increase impulse flow to the terminal field (Nisell et al. 1994a, b). Additional studies suggest that the reinforcing properties of nicotine, measured using a self-administration paradigm, also depend upon the stimulation of the DA neurons in the VTA which project to the accumbens (Corrigall et al. 1994). These results support the conclusion that effects of self-administered nicotine on DA release in the forebrain, which underpin nicotine reinforcement, depend upon its ability to stimulate impulse flow to the terminal fields.
A single acute injection of nicotine elicits a sustained increase in the concentration of DA in the extracellular space of the accumbens shell that persists for up to 60 min. However, there is evidence that many of receptors on the DA neurons in the VTA rapidly desensitize upon the exposure to the drug (Pidoplichko et al. 1997). In order to explain this conundrum, Mansvelder and McGehee (Mansvelder et al. 2002; Mansvelder and McGehee 2000, 2002) suggested that sustained increases in firing of the neurons were maintained by the differential desensitization of the nicotinic receptors located the DA neurons and the terminals of afferent GABA and glutamate neurons that regulate the activity of the neurons. They argued that this generates a triphasic response to the drug. When nicotine is administered, it initially stimulates the nicotinic receptors located on the somatodendritic membranes of the DA neurons. This leads to an initial short-lived stimulation of the neurons. Nicotine also stimulates nicotinic receptors on GABA terminals facilitating the release of this inhibitory transmitter. This results a short-lived inhibition of the DA neurons following the initial stimulation. These authors suggested that receptors on the DA neurons and GABA terminals are composed of α4β2 subunits and readily desensitize within seconds or minutes of exposure to the drug. A third population of nicotinic receptors, located on glutamate terminals, is composed predominantly of α7 subunits. These receptors are more resistant to desensitization and remain active over a longer period of time, facilitating the release of glutamate in the VTA. The authors suggest that the sustained increase in neuronal activity and DA overflow, evoked by the acute administration of nicotine, depends upon this sustained release of glutamate and that this mechanism is important to the role of DA in nicotine dependence (Fagen et al. 2003).
The paragraphs above have focused on the DA responses to acute nicotine administered to drug-naïve animals. These studies have generated convincing evidence that nicotine injections stimulate the mesoaccumbens DA neurons that have been implicated in drug dependence. However, the development of nicotine dependence is the product of chronic or repeated exposure to the drug, and if we are to understand the role of these projections in dependence, then it is essential that the changes in the neural responses to nicotine that occur with chronic exposure to the drug are fully characterized. Studies in our laboratory were the first to explore the effects of repeated daily injections of nicotine on DA overflow in the nucleus accumbens (Benwell and Balfour 1992). These experiments showed that, whereas acute injections of nicotine had little or no effects on DA overflow in the core subdivision of the nucleus accumbens, repeated administration of the drug, on as few as five occasions, was sufficient to elicit sensitization of its effects on DA overflow in this region of the brain. Some years later, this observation was confirmed by Cadoni and Di Chiara (2000) who also demonstrated that sensitization was only observed in the core subdivision of the accumbens. Repeated administration of nicotine does not result in sensitization of the DA overflow evoked by nicotine in the accumbal shell. Indeed, in this subdivision of the nucleus accumbens, repeated nicotine administration results in the development of tolerance to the response to nicotine (Cadoni and Di Chiara 2000), an observation subsequently confirmed in our own laboratory (Iyaniwura et al. 2001; Tronci and Balfour 2011). Furthermore, repeated nicotine administration does not result in sensitization of DA overflow in the dorsolateral striatum (Benwell and Balfour 1997). Repeated daily administration of other drugs of abuse also results in a regionally selective sensitization of their effects of DA overflow in the core subdivision of the accumbens (Cadoni and Di Chiara 1999; Cadoni et al. 2000).
The extent to which the electrophysiological responses to nicotine are modified by chronic or repeated exposure to the drug remains to be clarified. However, there is evidence that pretreatment with nicotine diminishes the regulation by D2 auto-receptors of DA release in the nucleus accumbens (Balfour et al. 1998). By contrast, nicotine pretreatment does not alter the regulatory effects of these receptors on DA overflow in the dorsoalateral striatum, data which suggest that the regionally selective sensitization of the response to nicotine in the accumbal core could be associated with diminished autoreceptor regulation of the neurons. Other studies in our laboratory demonstrated some years ago that both the development and expression of the sensitized DA responses to nicotine, observed in the accumbens core, are inhibited by pretreating the animals with an NMDA receptor antagonist (Balfour et al. 1996; Shoaib et al. 1994). These results imply that the enhanced overflow of DA in the accumbal core depends upon the co-stimulation of the glutamatergic receptors. The anatomical location of the receptors that mediate the sensitized responses to nicotine has not been identified although some data support the conclusion that the receptors are likely to be located in the VTA and to modulate the effects of nicotine on impulse flow to the terminal field (Fu et al. 2000; Schilstrom et al. 1998). This conclusion is consistent with the evidence that the sustained increases in DA overflow, evoked by a nicotine injection, are maintained by the release of glutamate in the VTA (Mansvelder et al. 2002). The sensitized DA response is also attenuated by pretreatment with the indole alkaloid, ibogaine, an effect that persists for at least 24 h after administration of the alkaloid (Benwell et al. 1996). The compound has been reported to attenuate the increases in DA overflow evoked by other drugs of dependence, and this property is thought to underpin the potential of this compound and its synthetic derivative 18-methoxycoronaridine as treatments for drug dependence (Glick and Maisonneuve 2000). Ibogaine is an antagonist at α4β2 nicotinic receptors, and its effects at this receptor are long lasting. Thus, this could explain the inhibition evoked by the drug. However, if this is the mechanism, it is surprising that pretreatment with ibogaine does not also attenuate the increase in locomotion evoked by nicotine. Ibogaine is also an NMDA receptor antagonist, and since its effects on the responses to nicotine resemble more closely those of other NMDA antagonists, it seems more likely that its effects at this receptor explain the interaction with nicotine in our experiments (Benwell et al. 1996).
For a majority of the studies described in the previous paragraph, DA overflow was measured in the absence of an inhibitor of the neuronal DA transporter. It is assumed, therefore, that the measures reflect changes in the extracellular DA concentration that occur in vivo in response to the administration of the drug. However, the electrophysiological studies suggest that the effects of nicotine on the neuronal firing are not restricted to the neurons that project to the accumbal shell. Some years ago, our group investigated the effects of nicotine on catecholamine overflow measured in the presence of a drug, nomifensine, which inhibits the neuronal DA and noradrenaline transporters (Benwell and Balfour 1997). In the presence of this inhibitor, acute injections of nicotine to drug-naïve animals also increase DA overflow in the accumbal core and dorsolateral striatum, whereas no changes are observed if the experiment is performed in the absence of the inhibitor. These data imply that the acute systemic administration of nicotine exerts a more generalized stimulation of the activity of DA neurons in the VTA and the substantia nigra but that the effects are masked by the efficient reuptake of the monoamine by the transporters. The conclusion is supported by more recent studies which suggest that the increase in DA release evoked by changes in phasic burst firing of the neurons can be detected in the presence of an inhibitor of the transporters (Floresco 2007). Thus, it is possible that the regionally selective increase in DA overflow in the accumbal shell, measured in response to acute nicotine administration, could reflect differences in nature of the effects of the drug on the activity of the DA neurons that project to this subdivision of the accumbens. Jones et al. (1996), however, have highlighted evidence that the characteristics and regulation of the DA transporters in the accumbal shell differ from those found in the core subdivision of the structure and that this may explain the regional differences in the DA responses in the accumbens.
3 Tonic and Phasic Firing of Mesolimbic Dopamine Neurons
DA neurons have been shown to fire in two distinct modes—as irregular single spikes and as short periods of burst firing (Grace and Bunney 1984a, b). Stimulation of the burst firing of the neurons evokes a substantial increase in the DA release into the synaptic cleft and generally leads to only a transitory increase in extracellular DA (Heien and Wightman 2006). By contrast, the concentration of DA in the extracellular space is governed primarily by the tonic spike activity of the neurons (Floresco 2007; Floresco et al. 2003; Heien and Wightman 2006) although a recent study by Owesson-White et al. (2012) suggests that transient phasic firing of the neurons may also contribute to changes in the concentration of DA in the extracellular compartment of the nucleus accumbens. Nirenberg et al. (1997) used high-resolution electron microscopic immunocytochemistry to demonstrate that a majority of the neuronal DA transporters are located extra-synaptically on plasma membranes of both the accumbens shell and core. DA transporters are similarly predominantly located outside synapses in the dorsal striatum (Hersch et al. 1997). These observations support the conclusion, first mooted by Garris and Wightman (1994), that the primary role of DA transporters in the nucleus accumbens is to regulate the concentration of DA in the extracellular space. The release of DA into the extracellular space could reflect overflow from synapses. However, Nirenberg et al. (1997) noted that the DA fibers that project into the nucleus accumbens are beaded, having varicosities along their length. This observation led Balfour et al. (2000) to propose that tonic DA release may reflect release directly into the extracellular space from these varicosities and that this DA serves a different functional role from that released by terminals that form tight synaptic contacts.
An important putative role for extracellular DA in the VTA may be autoreceptor-mediated inhibition of DA release evoked by phasic firing of the neurons (Grace 2000). Thus, the level of DA tone may influence the level of response to conditioned stimuli whose salience has been established by pairing with a rewarding outcome. There is also evidence that DA released into the extracellular compartment may be implicated in volume transmission, a form of neurotransmission that allows the transmitter to elicit a tonic regulation of “hard-wired” neuronal pathways which may be close to or some distance from the DA neurons (Fuxe et al. 2010; Garris and Wightman 1994). In this way, the transmitter may serve a “paracrine-like” role as a gate which influences the intensity of the neural traffic within fibers of passage. This possibility is consistent with the role attributed to the DA projections to the accumbens suggested by Sesack and Grace (2010). This chapter will focus on the possibility that DA release into the extracellular space of the accumbens and dorsal striatum may play complementary roles in the development of nicotine dependence.
There is also a considerable and growing body of evidence to suggest that the different modes of firing of the DA neurons which project to the nucleus accumbens mediate different components of the behavioral responses which depend upon the increased DA release in this area of the brain. Under normal conditions, many of the DA-secreting neurons in the VTA are inhibited by very active GABA neurons which project from a number of anatomical sites. The GABA projections from the ventral pallidum seem to play a particularly important role in suppressing the spontaneous activity of mesencephalic DA neurons (Floresco et al. 2001, 2003). As a result, approaching 50 % of the DA neurons in the VTA is not spontaneously active. Inactivation of the ventral pallidum releases this inhibitory effect and allows more of the neurons to fire spontaneously (Floresco et al. 2003; Lodge and Grace 2006a). Furthermore, excitation of glutamate afferents in the ventral subiculum of the hippocampus also inhibits the GABA projections from the ventral pallidum to the VTA by stimulating inhibitory GABA projections from the nucleus accumbens to the ventral pallidum. As a result, the DA neurons within the VTA are disinhibited, resulting in an increase in the proportion of the cells which are spontaneously active (Grace et al. 2007; Lodge and Grace 2006a). Phasic burst firing of the neurons is promoted by stimulation of NMDA receptors located on the cells (Chergui et al. 1993). Stimulation of these receptors is not alone sufficient to generate burst firing of the neurons. Burst firing is generated by the coincidental stimulation of the NMDA receptors and other stimulatory receptors located on the neurons. Other studies suggest that co-stimulation of a projection from the laterodorsal tegmentum is also required to elicit burst firing of the neurons (Lodge and Grace 2006b). The nature of the neurotransmitter released by the neurons that project from the LTDg remains unclear although some evidence suggests that the neurons may be cholinergic in nature and that the acetylcholine released by these neurons acts on nicotinic receptors putatively located on the DA cells (Mameli-Engvall et al. 2006). Thus, the LDTg seems to provide a cholinergic-dependent permissive “gate” that allows stimulation of the glutamate receptors to result in the expression of burst firing. The source of the glutamatergic pathways which elicit burst firing also remains to be established with certainty although there is evidence that glutamate projections from the pedunculopontine tegmentum (PPTg) directly regulate burst firing of DA neurons in the VTA. The PPTg receives projections for other sites within the brain (e.g., the prefrontal cortex and extended amygdala) and is, therefore, ideally suited to the integration of the effects of a range of sensory inputs on the burst firing of VTA DA neurons (Grace et al. 2007).
Selective ablation of the cholinergic neurons in the PPTg reduces nicotine self-administration (Lanca et al. 2000). Furthermore, this group also showed that microinjections of the β2 subunit-selective nicotinic receptor antagonist, dihydro-β-erythroidine, into the PPTg exert a similar effect on nicotine self-administration. The data, when taken together, support the hypothesis that stimulation of cholinergic neurons within the PPTg is required to stimulate the DA neurons in the VTA and, thus, mediate the reinforcing properties of nicotine. Subsequent studies suggested that various pharmacological manipulations of neurons within the PPTg exert common effects on nicotine and cocaine self-administration although GABAergic neurons within the structure appear to exert a selective effect on the self-administration of nicotine (Corrigall et al. 2001, 2002). A later study by Alderson et al. (2006) found that lesioning the posterior PPTg with the excitotoxin, ibotenic acid, increased nicotine self-administration. By contrast, similar lesions of the anterior PPTg were without effect. The significance of these findings lies in the fact that the neurons in the posterior PPTg project to the VTA, whereas those in the anterior PPTg project preferentially to the substantia nigra. The effects of the posterior PPTg lesions on nicotine self-administration were hypothesized to reflect either a reduction in intrinsic nicotine reward value or enhancement of associative incentive salience. The results, however, do not preclude the possibility that the lesions ablate GABA neurons in the posterior PPTg that are implicated in the regulation of DA neurons in the VTA.
The phasic activity of mesencephalic DA neurons is stimulated by exposure to external stimuli and signals the relationship between an external stimulus and the outcome of a behavioral response. The system is most effective at encoding information about rewards although some DA neurons within the VTA also respond to aversive outcomes and may encode information about stimuli associated with these outcomes (Schultz 2010). Phasic firing of the neurons is initially stimulated by exposure to an unanticipated reward (i.e., when the presentation of a stimulus first results in the presentation of a reward such as a sweetened pellet). As the animal learns the association, the stimulus takes on the properties of a conditioned stimulus and the presentation of the stimulus results in phasic activity rather than the presentation of the reward. A failure to present the anticipated reward results in a depression of phasic activity, an outcome described as a prediction error. The magnitude of the phasic response is influenced by the magnitude of the reward presented and by the length of the delay between the stimulus and the presentation of the reward. Thus, the degree of phasic firing seems to code for the subjective value of the reward rather than its objective value (Schultz 2010). If the presentation of the conditioned stimulus prompts a behavioral response (e.g., a lever press) that delivers the reward, then it can be seen that an important role of phasic firing of the neurons is to promote a behavioral response which is advantageous to the animal. Schultz has proposed that this process facilities economic decision making—the selection of the best response to the stimuli presented (Schultz 2007, 2010). Schultz (2011) argues that chronic exposure the drugs of abuse elicits changes in the regulation of midbrain DA neurons and postsynaptic DA receptors and the morphology of DA-receptive cells that impair the ability of the individual to discriminate between rewards. Furthermore, chronic exposure to the drugs increases the effects of prediction errors (when a reward predicted by a stimulus is not presented) and enhance neuronal responses to reward-predicting stimuli.
3.1 The Effects of Nicotine on Phasic and Tonic Firing of Dopamine Neurons
Early studies suggested that the acute injections of nicotine to experimental animals increased both the population spike activity and burst firing of the DA neurons that project from the both the substantia nigra and VTA (Grenhoff et al. 1986). More recent studies have shown that the administration nicotine to experimental animals predominantly increases the number, duration, and frequency of phasic burst firing events evoked in the DA neurons of the VTA and substantia nigra (Zhang et al. 2009). These authors also found that there are fundamental differences in the frequency-dependent release of DA from terminals in the accumbal shell and dorsolateral striatum which favor DA overflow in the accumbal shell. As a result, the increase in phasic burst firing, evoked by an injection of nicotine, resulted in a preferential increase in DA release in the shell, results that are consistent with the results of earlier microdialysis studies (Benwell and Balfour 1997; Cadoni and Di Chiara 2000). This group also reported that nicotine injections substantially diminish DA release evoked by low-frequency tonic activity of the neurons. Thus, phasic DA activity and release is favored following an injection of nicotine and, as a result, nicotine injections enhance the “signal-to-noise relationship” within the terminal fields. A more recent study (Li et al. 2011) suggests that an acute nicotine injection also results in the development of synchronous firing of a subset of DA neurons within the VTA, a factor that may also contribute to the substantial increase of DA overflow into the extracellular space of the accumbal shell.
The administration of cocaine is also reported to elicit a preferential enhancement of phasic burst firing of the DA neurons that project to the accumbal shell and that this contributes to the increase in DA overflow evoked by the drug (Aragona et al. 2008). An earlier study from the same group showed that both non-contingently administered cocaine and contingent self-administration of the drug enhance phasic burst firing of the mesolimbic DA neurons that project to the nucleus accumbens (Stuber et al. 2005). Non-contingent cocaine administration to both cocaine-naïve rats and rats with previous experience of the drug evoked a substantial increase in the transient release of DA which was not time-locked to specific environmental stimuli. The contingent administration of the drug also evoked the changes in phasic release which were not time-locked to a stimulus. However, a subset of the phasic release events were time-locked to the lever-pressing responses, results which are consistent with the evidence that these transients relate the lever-pressing responses to conditioned stimuli that predict drug delivery (Phillips et al. 2003). Similar studies have yet to be performed with nicotine. However, the data support the working hypothesis that the administration of psychostimulant drugs of abuse elicits characteristic changes in the firing pattern of mesoaccumbens DA neurons. Furthermore, a significant proportion of the enhanced bursting activity of the neurons does not appear to be time-locked to the presentation of relevant conditioned stimuli and may, thus, subserve a different function putatively related specifically to dependence.
4 The Role of Mesoaccumbens Dopamine Projections in Locomotor Responses to Nicotine
The DA projections from the VTA area play a complex role in the regulation of behavior. However, two aspects of behavior, stimulation of locomotor activity and responding for rewarding stimuli or outcomes, have been particularly associated with increased DA release from the mesolimbic DA neurons that project to the nucleus accumbens. The acute administration of nicotine to rats causes a dose-dependent reduction in locomotor activity during the first 20 min of the trial if the animals are placed in the test arena immediately after the injection (Clarke and Kumar 1983). However, if the animals are left in the activity cage for 80 min, the activity is increased in a dose-dependent manner during the 40–60- and 60–80-min subtrials as the animals habituate to the test environment. In a subsequent microdialysis study, Benwell and Balfour (1992) used a similar paradigm to explore the effects of acute nicotine on DA overflow in the core subdivision of the accumbens in conscious freely moving rats tested in an activity box. In this study, the animals were left for 80 min to generate stable baseline levels of extracellular DA and habituate to the box before nicotine was administered to the rats. When compared with the responses measured in control animals given the saline vehicle, an acute injection of nicotine caused a dose-dependent increase in locomotion although increased DA overflow could only be detected in these rats in the presence of an inhibitor of the DA transporter (Benwell and Balfour 1997). Pretreatment with nicotine for several days prior to the test day results in sensitization of locomotor response to the drug and evidence that the animals have become tolerant to the initial inhibitory effects of the drug on activity (Clarke and Kumar 1983). If DA overflow is measured in rats sensitized to nicotine in this way, a substantially enhanced DA response to the drug is also observed (Benwell and Balfour 1992). This observation was confirmed in a subsequent study which showed that the sensitized DA response to the drug was observed in the accumbal core but not the shell (Cadoni and Di Chiara 2000).
Other studies have shown that motor sensitization to nicotine is also observed if rats are given daily microinjections of subthreshold doses of nicotine directly into the VTA (Panagis et al. 1996). Furthermore, selective lesions of the DA projections to the nucleus accumbens are reported to attenuate or block the increases in activity observed in nicotine-tolerant rats (Clarke et al. 1988; Louis and Clarke 1998; Panagis et al. 1996). A subsequent study showed that the sensitized locomotor response to nicotine and the locomotor stimulation evoked by amphetamine are selectively attenuated by lesions of the DA projections to accumbal core but not the medial shell (Boye et al. 2001). Similar studies with amphetamine suggest that the locomotor stimulant properties of the drug depend upon the increased DA release in the accumbal core whereas the reinforcing properties of the drug, measured using a place preference paradigm, depend upon the increased DA overflow in the medial shell of the accumbens (Sellings and Clarke 2003). These observations imply that the rewarding and locomotor-activating properties of stimulants are mediated by anatomically distinct components of the accumbens and appear consistent with the hypothesis that accumbal core is implicated in the regulation of motor activity, whereas the accumbal shell forms an integral component of the limbic systems of the brain (Heimer et al. 1991).
The results summarized in the previous paragraph would appear to provide convincing support the hypothesis that the development and expression of sensitized locomotor responses to nicotine depend upon the enhanced stimulation of the DA neurons that project from the VTA and an associated increase of DA overflow in the core subdivision of the accumbens (Benwell and Balfour 1992; Cadoni and Di Chiara 2000; Heimer et al. 1991). However, a number of studies in this laboratory have cast significant doubt on this working hypothesis. The expression of the sensitized DA responses in the accumbal core is antagonized if the injections of nicotine are preceded by an injection of an NMDA receptor antagonist (Balfour et al. 1996). By contrast, pretreatment with receptor antagonists does not cause a significant attenuation of the sensitized locomotor responses to the drug. Similarly, the attenuation of the sensitized DA responses to nicotine seen in animals pretreated with ibogaine is also not associated with a reduction in the hyperlocomotion evoked by nicotine. Furthermore, pretreating animals with an NMDA receptor antagonist during the sensitization phase of the experiment also attenuates the expression of the sensitized DA response, but not the hyperlocomotion (Shoaib et al. 1994). The more recent studies have explored the effects of 6-methyl-2-(phenylethynyl)-pyridine (MPEP) on the locomotor and DA responses to nicotine. MPEP is a negative allosteric modulator at metabotropic receptor 5 (mGluR5) receptors (Rodriguez et al. 2010). These experiments suggest that the locomotor response to nicotine is also unaffected if the animals are pretreated with MPEP at a dose that attenuates the effects of nicotine on DA overflow in the accumbal shell (Tronci et al. 2010). Thus, these data suggest that the locomotor stimulation, evoked by nicotine, is not related to increased DA overflow in either subdivision of the accumbens. Other studies suggest that the sensitized motor responses to nicotine may be associated with enhanced postsynaptic responses to DA in the nucleus accumbens (Birrell and Balfour 1998; Suemaru et al. 1993). These observations have led our group to propose that the locomotor stimulant properties of nicotine may be associated with increased DA release in synapses in the nucleus accumbens, whereas the extracellular DA in this region of the brain is more concerned with other aspects of nicotine psychopharmacology, including specifically nicotine dependence (Balfour et al. 2000).
5 The Role of Mesolimbic Dopamine in Nicotine Dependence
5.1 Studies with Animal Models of Nicotine Reinforcement
There is convincing evidence that the rewarding or reinforcing properties of nicotine, measured using a self-administration paradigm, depend upon the DA release from mesoaccumbens DA neurons. This conclusion is supported by the evidence that selective lesions of the DA projections to the accumbens result in a substantial reduction of intravenous nicotine self-administration in animals that have been trained in an operant chamber to press for nicotine (Corrigall et al. 1992). Furthermore, the local administration of nicotine receptor antagonists into the VTA evokes a similar attenuation of self-administration, data that support the view that the reinforcing properties of nicotine, measured using this paradigm, depend upon the stimulation of the DA neurons that project from the VTA (Corrigall et al. 1994). It is important to remember, however, that these observations may not be as compelling as they appear because lesioning procedures, similar to those adopted to attenuate nicotine self-administration, also attenuate the stimulatory effects of repeated nicotine on locomotor activity (Clarke et al. 1988; Louis and Clarke 1998). Moreover, the effects of intra-VTA nicotine on locomotion are inhibited by the prior administration of a nicotinic receptor antagonist (Reavill et al. 1990).
A most recent study by Cadoni et al. (2009) has shown that the self-administration of nicotine to inbred Lewis rats has a greater effect on DA overflow in both the nucleus accumbens shell and core than in the accumbens of inbred Fischer 344 rats. The study also showed that the Lewis rats were also more sensitive to the increase in locomotor activity, evoked by low doses of nicotine. A previous study suggested that, whereas Lewis rats can be trained to self-administer nicotine, Fischer 344 rats do not readily acquire this response (Brower et al. 2002). Furthermore, the Lewis rats that acquired nicotine self-administration responded preferentially on the active lever. The authors suggested that the data supported the conclusion that the Lewis strain may be genetically susceptible to the reinforcing properties of nicotine that underpin dependence. It is important to note, however, that Bower and colleagues used an extended unlimited access paradigm in which the animals were able to respond for nicotine for 23 h. By contrast, an earlier study by Shoaib et al. (1997), in which self-administration was measured over 1 h daily sessions, showed that neither Fischer nor Lewis rats readily acquired nicotine self-administration. Thus, the potential association between the genetic effects on DA overflow and reward, as measured using intravenous self-administration, should be treated with some caution.
The rewarding properties of nicotine can also be explored using a conditioned place preference paradigm in which one compartment of the apparatus is repeatedly paired with a nicotine injection. There is evidence that nicotine-induced conditioned place preference is attenuated if the DA projections to the nucleus accumbens are selectively lesioned (Di Chiara 2000). A more recent study showed that attenuation of nicotine-induced conditioned place preference could be evoked by selective lesions of the DA terminals in the accumbal shell, whereas lesions of the terminals in the accumbal core resulted in increased preference for the nicotine-paired compartment (Sellings et al. 2008). These authors concluded that the DA projections to the shell mediated the “rewarding” properties of the drug, whereas the DA projections to the accumbal core played a role in mediating the aversive properties of nicotine. However, as will be made clear later in this chapter, other roles have also been attributed to the DA neurons that project to the accumbal core.
Other researchers have employed more indirect approaches to explore the role of DA in the psychopharmacological responses to nicotine. For example, there is evidence that constituents of tobacco smoke cause inhibition of monoamine oxidase (MAO) activity in the brain and this effect is thought to enhance the addictive potential of nicotine when delivered in tobacco smoke (Brody 2006; Fowler et al. 2003). This hypothesis is supported by results reported by both Guillem et al. (2005) and Villegier et al. (2007) in which nicotine self-administration was shown to be enhanced by pretreating the animals with a drug that inhibits monoamine oxidase. A subsequent study (Guillem et al. 2006) demonstrated that this enhancement was particularly marked in animals pretreated with a selective MAO-A inhibitor but not in animals pretreated with an MAO-B inhibitor. In contrast to human brain, in which both isoforms of the enzyme are implicated in DA metabolism, in rat brain DA is preferentially metabolized by MAO-A (Saura et al. 1992). Thus, results with experimental animals are consistent with the possibility that the enhanced motivation to respond for nicotine is associated with the potentiation of DA. In other studies, Cohen et al. (2002) showed that pretreating rats with the cannabinoid CB1 receptor antagonist, rimonabant, attenuated both the effects of nicotine on DA overflow in the nucleus accumbens shell and nicotine-seeking behavior in a self-administration study. These data provide further support for the hypothesis that increased DA overflow in the accumbal shell plays a central role in nicotine-reinforced behavior.
5.2 Studies with Other Psychostimulant Drugs of Dependence
In order to be able to interpret the results of studies with nicotine more clearly, it is instructive to consider how studies with other psychostimulant drugs of dependence have contributed to our understanding of the role of mesolimbic DA in addiction. Studies with cocaine have shown that rats can be trained to self-administer cocaine directly into the shell subdivision of the accumbens, whereas they cannot be trained to self-administer the drug directly into the accumbal core (Rodd-Henricks et al. 2002). Furthermore, this response was attenuated by the co-administration of the DA receptor antagonist, sulpiride, results which suggest that the reinforcing properties of cocaine delivered into the accumbal shell depends upon the stimulation of D2 receptors in this subdivision of the accumbens. Other studies have shown that the rewarding properties of amphetamine, measured using the place preference paradigm, are attenuated by selective lesions of the DA terminals in the accumbal shell (Sellings and Clarke 2003). Other anatomical loci have also been implicated in the reinforcing properties of psychostimulant drugs. Ikemoto (2003) noted that the DA neurons that project to the olfactory tubercle also seem to play a role in the reinforcing properties of cocaine. Furthermore, Sellings et al. (2006) extended these reported that the reinforcing properties of methylphenidate area associated with increased DA release in the medial olfactory tubercle. Thus, there is a need to be cautious when attributing the locomotor stimulant and reinforcing properties of nicotine solely to the events in the two principal subdivisions of the nucleus accumbens.
While the evidence that DA neurons play a central role in the reinforcing properties of psychostimulant drugs that underpin dependence is reasonably compelling, some studies suggest that the relationship is not simple. For example, Rocha et al. (1998) showed that transgenic mice lacking function neuronal DA transporters were hyperactive and could still learn to self-administer cocaine in manner that seemed similar to that seen for wild-type animals. The authors concluded that the reinforcing properties of cocaine cannot depend solely on its ability to inhibit the DA transporter and that other neural responses also play a key role in cocaine reinforcement. Studies with other drugs of abuse also suggest that DA may play a specific role in drug reinforcement. There is evidence that mesolimbic DA neurons may facilitate acquisition of opiate self-administration in rodents (Bozarth and Wise 1984; Singer and Wallace 1984). However, Pettit et al. (1984) demonstrated that, once the response had been acquired, lesions of the DA projections to the accumbens have no significant effects of heroin self-administration, whereas the lesions did attenuate established cocaine self-administration. Similar findings were reported by Gerrits and Van Ree (1996) who suggested that the results argued against a critical role for mesolimbic DA in the motivational mechanisms underpinning drug dependence. A subsequent study by Cannon and Palmiter (2003) showed that transgenic mice lacking tyrosine hydroxylase, and therefore the ability to synthesize DA, could still learn to respond for a sucrose reward. Thus, the data taken together support the possibility that increased DA release in the nucleus accumbens may facilitate the acquisition of responding for rewards or reinforcers but that it is not an absolute requirement.
5.3 Does Extracellular Dopamine in the Accumbal Shell Have a Specific Role in Nicotine Dependence?
Since a majority of drugs of dependence stimulate DA release and/or sustain DA overflow into the extracellular space, it is long been argued that this property of the drugs contributes significantly to neuropharmacological mechanisms which underpin the development of dependence (Di Chiara 1995; Wise 1987). Drug dependence is a chronic relapsing condition and it follows that the changes in the activity of mesolimbic DA neurons which occur as a consequence of chronic exposure to these drugs is likely to contribute to the mechanisms that underpin the transition to dependence. As already discussed, daily injections of nicotine result in sensitization of DA overflow in the core subdivision of the accumbens (Benwell and Balfour 1992; Cadoni and Di Chiara 2000; Iyaniwura et al. 2001). By contrast, the repetitive administration of nicotine, using the same protocol, does not elicit sensitization of DA overflow in the accumbal shell or dorsolateral striatum (Benwell and Balfour 1997; Cadoni and Di Chiara 2000; Iyaniwura et al. 2001). Indeed, some studies have shown that the effects of nicotine on DA overflow in the shell subdivision exhibit tolerance to nicotine if the drug is administered repeatedly (Cadoni and Di Chiara 2000; Tronci and Balfour 2011). If the animals are allowed to self-administer nicotine, a different pattern of responses is observed in the two principal subdivisions of the accumbens (Lecca et al. 2006). During the first week of nicotine self-administration, the effects of the drug on DA overflow in the nucleus accumbens shell are clear and significant. By contrast, the effects on DA overflow in the accumbens core are modest and do not achieve statistical significance. Thus, the effects of self-administered nicotine on DA overflow in the principal subdivisions of the accumbens resemble those seen in animals treated acutely with nicotine. During the third week of nicotine self-administration, there is a modest but statistically significant sensitization of the DA overflow in the accumbal core. However, in contrast to the effects of non-contingent nicotine, three weeks of self-administered nicotine result in sensitization of its effects on DA overflow in the accumbal shell. Similar sensitization of DA overflow in the accumbal shell is also observed in animals trained to self-administer cocaine or heroin (Lecca et al. 2007a, b). These observations support the view that preferential sensitization of the increase in DA overflow in the accumbal shell is a specific characteristic associated with the acquisition of contingent drug self-administration and play an important role in behaviors associated with the drug-taking behavior.
The putative behavioral significance of the changes in extracellular DA remains to be established. Grace (2000) has argued that the primary role of extracellular DA is to regulate phasic DA release through the DA autoreceptors and that the persistent increase in extracellular DA, evoked by chronic exposure to drugs of abuse, results in a sustained suppression of phasic responses. He posits that the craving to take drugs of abuse reflects a need to overcome this increased inhibitory effect and, thus, restore normal phasic DA release. Others have argued that increased extracellular DA, especially in the accumbal shell, plays a more direct role in drug-seeking behavior (Balfour 2009; Di Chiara et al. 2004). For nicotine, this hypothesis receives support from a number of studies which report that treatments which inhibit the increase in extracellular DA in the nucleus accumbens shell, evoked by the drug, also attenuate nicotine self-administration and conditioned place preference (Balfour 2009; Cohen et al. 2005, 2002; Di Chiara et al. 2004; Scherma et al. 2012, 2008; Tronci and Balfour 2011). Furthermore, in animals in which responding for the drug has been extinguished, the reinstatement of nicotine-seeking behavior, evoked by a non-contingent injection of nicotine, is also attenuated by a treatments that attenuate the increase in DA overflow in the accumbal shell evoked by nicotine (Mascia et al. 2011; Scherma et al. 2008). None of the studies have sought to determine whether the treatments act selectively or preferentially on phasic or tonic firing of the neurons.
The results presented above are consistent with the hypothesis that, like other psychostimulant drugs of abuse, increased DA overflow in the accumbal shell mediates the “rewarding” properties of nicotine. However, Salamone and colleagues (Salamone and Correa 2013; Salamone et al. 2012) have summarized a considerable body of evidence which suggests that responding for natural rewards, such as food, does not depend upon the stimulation of the DA projections to the nucleus accumbens. These pathways, however, do enhance behavioral activation, the motivational salience of the rewards, and effort-related choice. This hypothesis is consistent with an earlier study which demonstrated that transgenic mice, lacking a key enzyme (tyrosine hydroxylase) in the biosynthetic pathway for DA, still exhibit robust preference for sucrose over water although the transgenic animals consumed less sweetened solution than the wild-type controls (Cannon and Palmiter 2003). The authors concluded that the mice lacking DA exhibited a deficiency in goal-directed behavior rather than an impaired perception of the reward. Thus, there is a growing consensus that the DA projections to the accumbens influence the motivation to respond for a reinforcer, especially in an instrumental paradigm, rather than mediating their rewarding properties per se. It also seems reasonable to suggest, therefore, that compulsive drug-seeking and drug-taking behaviors reflect the effects of addictive drugs on these processes (Balfour 2009; Salamone and Correa 2012; Salamone et al. 2007). Salamone (Nunes et al. 2013; Salamone et al. 2012) and his colleagues have focused on the possibility that increased DA overflow into the extracellular space of the nucleus accumbens, including that evoked by drugs of dependence, enhances behavioral activation and effort-related choice behavior for rewards. By contrast, psychopathological conditions, such as depression, are posited to be associated with reduced DA release in this area of the brain and that this effect mediates the psychomotor slowing, anergia and apathy that characterize these conditions.
The increases in extracellular DA in the nucleus accumbens, evoked by the administration of nicotine and other drugs of dependence, are generally sustained for periods up to an hour or more. Thus, it seems reasonable to posit that they mediate the effects of sustained diffuse stimuli on drug-seeking or drug-taking behavior that are not time-locked to specific-conditioned stimuli (Balfour 2009). Studies in a number of laboratories have shown that nicotine self-administration is attenuated by pretreating the animals with an mGluR5 receptor antagonist (Palmatier et al. 2008; Paterson et al. 2003; Tessari et al. 2004). Tronci and colleagues (Tronci and Balfour 2011; Tronci et al. 2010) showed that this attenuation of nicotine self-administration is evoked at doses of antagonist that also attenuate the increase in DA overflow evoked by nicotine in the accumbens shell (Fig. 2). These data would appear to support the hypothesis that increased DA overflow in the accumbal shell mediates nicotine reinforcement in this instrumental paradigm and that the response to nicotine depends upon the co-stimulation of mGluR5 receptors. A parallel study by D’Souza and Markou (D’Souza and Markou 2011) showed that the microinfusion of an mGluR5 receptor antagonist directly into the VTA or accumbal shell attenuated nicotine self-administration. These results provide further support for a role for the DA projections to the accumbal shell in the reinforcing effects of nicotine and implicate mGluR5 receptors in these areas of the mesolimbic system in the response. However, the microinfusions into the VTA, but not the accumbal shell, also attenuated responding for a food reward. Systemic injections of moderate doses of MPEP, which attenuate nicotine self-administration, do not inhibit responding for a palatable food reward (Bespalov et al. 2005; Tronci and Balfour 2011) although it does attenuate the motivation to respond for food as determined using a progressive ratio paradigm (Paterson and Markou 2005). The effects of MPEP microinjections into the accumbal shell, therefore, most closely mimic the effects of systemic drug on nicotine self-administration, and it seems reasonable to conclude that the accumbal shell may be the primary site of the antagonists when they are administered systemically.
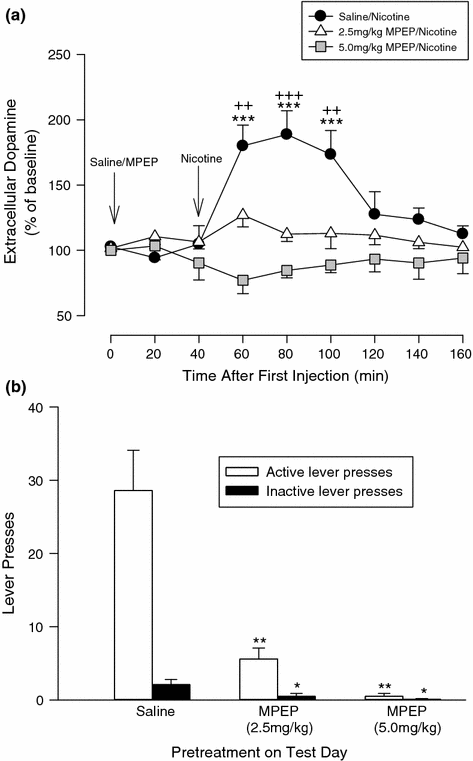
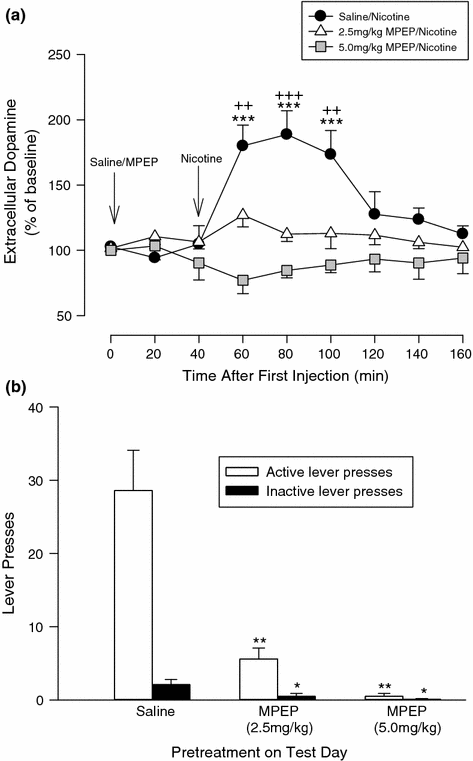
Fig. 2
The effects of MPEP on dopamine overflow in the nucleus accumbens and nicotine IVSA. Panel a show the effects of pre-injecting animals with MPEP (2.5 or 5.0 mg/kg IP) or its saline vehicle on the increase in DA overflow in the shell subdivision of the nucleus accumbens evoked by an acute SC injection of nicotine (0.4 mg/kg). Panel b shows the effects of the same doses of MPEP on lever-pressing in rats trained to respond for IV nicotine (30 µg/kg). The results are presented as means ± sem. Significantly different from rats pretreated with saline (**p < 0.01; ***p < 0.001). The figure is adapted from Tronci and Balfour (2011)
The reinforcing properties of nicotine that underpin dependence have been related to its ability to enhance brain reward function, as measured using an intracranial self-stimulation paradigm (O’Dell and Khroyan 2009) and to enhance the salience of other non-pharmacological reinforcers (Caggiula et al. 2002; Chaudhri et al. 2006; Donny et al. 2003; Palmatier et al. 2006). The reward-enhancing properties of nicotine are also observed in animals trained to self-administer the drug directly into the VTA, data which suggest that this property of the drug depends upon the stimulation of neurons, putatively DA neurons, that project from this area of the brain (Farquhar et al. 2012). Pretreatment with an mGluR5 receptor antagonist, however, is reported to have no effects on the reward-enhancing properties of nicotine (Kenny et al. 2003; Palmatier et al. 2008). Thus, although these facets of the nicotine psychopharmacology may be associated with stimulation of the DA projections from the VTA, it seems unlikely that they are dependent on the increase in extracellular DA evoked by the drug in the accumbal shell.
In experimental animals, both sensitization of the locomotor stimulant properties of nicotine and nicotine-seeking behavior in a self-administration paradigm can be conditioned to the environment in which the drug is administered (Bevins and Palmatier 2003; Diergaarde et al. 2008; Reid et al. 1998; Wing and Shoaib 2008). Furthermore, there is evidence that distal cues of this nature exert a significant influence on the craving to smoke tobacco (Le Foll and Goldberg 2005; Van Gucht et al. 2010). In the nicotine self-administration studies reported by Tronci and Balfour (2011), pretreatment with the mGluR5 negative allosteric modulator, MPEP, also suppressed responding on the inactive lever (Fig. 2). This observation implies that the drug does not act selectively on the reinforcing properties of nicotine. Moreover, as the dose of MPEP was increased, a higher proportion of the rats tested made no lever-pressing responses at all during the 1-h session (Table 1). This aspect of the response to MPEP, therefore, cannot be attributed to a neural interaction between nicotine and the mGluR5 antagonist. Moreover, studies on the effects of MPEP on locomotor activity indicated that the deficits in responding on the instrumental task did not reflect a general reduction in activity. MPEP pretreatment, however, selectively attenuated contextually conditioned hyperactivity in rats repetitively exposed to a maze following each daily injection of nicotine while having no significant effects on context-independent pharmacological sensitization of the locomotor response to the drug (Fig. 3) (Tronci et al. 2010). These observations seem most consistent with the possibility that a principal effect of MPEP is the attenuation of contextually conditioned behavioral responses to nicotine. Thus, MPEP seems to inhibit behavioral responses to nicotine in two ways. It attenuates the stimulation of DA overflow evoked in the nucleus accumbens by nicotine and, thereby, behaviors such as self-administration that depend upon this neural response. It also attenuates the effects of distal contextual cues that act, putatively through the hippocampus, to modulate the effects of nicotine on mesolimbic function and to promote nicotine-seeking behavior.
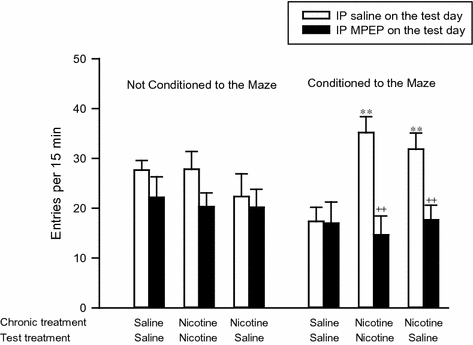
Table 1
The influence of MPEP on nicotine intravenous self-administration
MPEP dose (mg/kg) | Percentage of rats responding for nicotine |
---|---|
Saline | 100 |
2.5 | 88 |
5.0 | 38 |
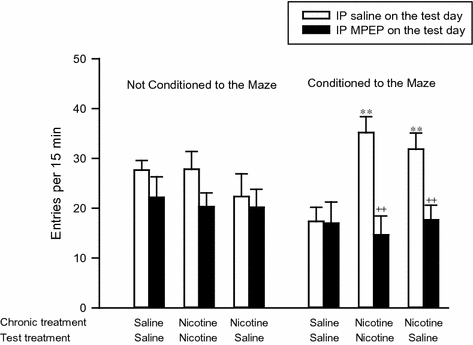
Fig. 3
The influence of MPEP on contextually conditioned locomotor sensitization to nicotine. Groups of rats was given daily injections of saline or nicotine (0.4 mg/kg SC) for 16 days. Half the rats in each treatment group were returned to their home cages; the remainder were place in a 4-arm maze for 15 min. On days 17 and 21, the rats were given IP saline (open columns) or MPEP (5 mg/kg; filled columns) using a counter-balanced design. The chronic treatment and the treatment on the test day are shown along the x-axis of the graphs. The figure shows the activity of the rats (entries into the arms of the maze) expressed as mean ±sem of 6 observations for rats that were habituated to saline or nicotine in the home cage or habituated to the maze after each injection. ** significantly different from saline/saline group: p < 0.01; ++ significantly different given IP saline: p < 0.01. Taken from Tronci et al. (2010) with permission
The effects of MPEP on the reward-enhancing properties of nicotine have also been explored using a paradigm similar to that described by Palmatier et al. (2007). MPEP pretreatment attenuated the enhanced responding for a non-pharmacological complex visual reinforcer seen in the animals given nicotine but also attenuated the moderate level of responding for the non-pharmacological reinforcer seen in animals trained with saline. As with the nicotine self-administration study, the effects of MPEP were not selective for the active lever. The data generated by this study, therefore, would appear consistent with the hypothesis that MPEP also attenuates contextually conditioned responding for this non-pharmacological reinforcer (Tronci et al. 2010). However, the data presented above contrast with those reported by Palmatier et al. (2008) who found no effects of MPEP on responding for the non-pharmacological reinforcer. The reason for the difference between the results of the two groups remains unclear although there are important differences in the experimental designs employed by the two groups which may explain why contextually conditioned responding contributed more significantly to the study performed by Tronci and Balfour (2011).
5.4 The Role of Hippocampal Projections in Dopamine Responses to Nicotine and Conditioned Contextual Stimuli
Studies using a place preference paradigm have shown that appetitive conditioning using a sucrose reward suggest that the neural circuitry that mediates the effects of conditioned spatial contextual cues on reward-seeking behavior differ from the effects of discrete conditioned stimuli (Ito et al. 2008). Significantly, these studies revealed that excitotoxic lesions of the accumbal shell attenuated the effects of the contextual cues, whereas lesions of accumbal core attenuated responding for discrete conditioned stimuli paired with presentation of the reward. The role of the accumbal shell depended upon the intact neural connections to the hippocampus. These results support the conclusion that limbic corticostriatal network plays a central role in spatial contextual conditioning. Moreover, studies with drugs of dependence suggest that the effects of spatial contextual stimuli on drug-seeking behavior are associated particularly with increased DA release in the accumbal shell (Bossert et al. 2007; Chaudhri et al. 2010; Crombag et al. 2008; Ito et al. 2000). DA overflow in the nucleus accumbens is increased by stimulation of glutamatergic projections from the hippocampus (Floresco 2007; Floresco et al. 2001; Legault and Wise 1999; Taepavarapruk et al. 2000). It has been proposed that these glutamatergic pathways play a pivotal role in the effects of distal contextual cues on behavior (Crombag et al. 2008). Crombag and colleagues focused on the role of glutamatergic projections from the dorsal hippocampus to the nucleus accumbens, whereas Grace and colleagues (Grace et al. 2007) have emphasized the role of the projections from the ventral hippocampus. The latter system is reported to enhance DA overflow in the nucleus accumbens shell via a circuit which projects through the ventral pallidum to the VTA by increasing the proportion of DA neurons that are tonically active. These observations imply that distal contextual cues may enhance the release of DA into the extracellular space of the shell of the accumbens by increasing the tonic activity of DA the neurons in the VTA. Stimulation of the glutamatergic afferents from the ventral subiculum to the nucleus accumbens increases both DA overflow and locomotor activity (Taepavarapruk et al. 2000). Studies to date have implicated both NMDA and non-NMDA glutamatergic receptors in this circuit (Floresco 2007; Floresco et al. 2001; Legault and Wise 1999; Taepavarapruk et al. 2000). There is a paucity of studies that have sought to explore the putative role of the hippocampus and its connections to the nucleus accumbens on nicotine-evoked contextual conditioning and, currently, there is no direct evidence that mGluR5 receptors are also implicated in the role of hippocampal afferents on DA release in the nucleus accumbens. However, the accumbal neurons that innervate the ventral pallidum are rich in mGluR5 receptor RNA, whereas only 50 % of the neurons that project directly to the VTA express these receptors (Lu et al. 1999), a finding that provides some indirect evidence for a role for mGluR5 receptor involvement in the circuit.
5.5 The Role of the Extracellular Dopamine in the Accumbal Core
The repetitive non-contingent administration of drugs of nicotine results in a subregionally selective sensitization of its effects on DA overflow into the extracellular space of the accumbal core, an effect that nicotine shares with other drugs of dependence (Cadoni and Di Chiara 1999, 2000; Cadoni et al. 2000; Iyaniwura et al. 2001). Both the acquisition and expression of this sensitized DA response are attenuated by pretreatment with drugs that antagonize NMDA glutamatergic receptors, data which suggest that the sensitized response depends upon the co-stimulation of these receptors (Balfour et al. 1996; Shoaib et al. 1994). Other data support the conclusion that the sensitized DA response is caused by increased phasic burst firing the neurons that project to the accumbal core (Balfour et al. 1998; Schilstrom et al. 1998). This hypothesis is supported by a more recent study which demonstrated that increased phasic burst firing of the DA projections to the accumbal core depends upon the co-stimulation of nicotinic receptors composed of α6β2 subunits and NMDA receptors located on the DA neurons (Wickham et al. 2013). Data presented earlier in the chapter excluded the possibility that the increase in DA overflow, evoked by nicotine in the accumbal core, mediates the sensitized locomotor response seen in these animals when they are challenged with nicotine. These findings pointed to the likelihood that the sensitized DA responses mediate some other aspects of nicotine psychopharmacology, putatively associated with dependence (Balfour 2009; Balfour et al. 2000) although the nature of this role remains a matter of speculation (Di Chiara 2002; Di Chiara and Bassareo 2007). The administration of an NMDA receptor antagonist has, however, been shown to attenuate both nicotine self-administration and the increased brain reward function elicited by a nicotine injection (Kenny et al. 2009). Reduced nicotine self-administration and nicotine-facilitated brain reward function has also been observed in animals in which the antagonist was delivered locally into the VTA (Kenny et al. 2009). Thus, the reinforcing and reward-enhancing properties of nicotine can speculatively be associated with increased burst firing of mesolimbic DA neurons, but not necessarily those which project to the accumbal core. Indeed a subsequent study (D’Souza and Markou 2014) has shown that the responding for a nicotine-associated conditioned cue is enhanced by the local administration of an NMDA receptor antagonist into the accumbal core.
Discrete conditioned stimuli, repetitively paired with the self-administration of cocaine, substantially enhance self-administration of the drug and selectively activate neurons in the accumbal core (Hollander and Carelli 2007; Ranaldi and Roberts 1996). Selective excitotoxin-evoked lesions of the accumbal core have little effect on schedule-controlled cocaine self-administration but substantially inhibit drug-seeking behavior maintained by drug-associated conditioned cues (Ito et al. 2004). Other studies, reported by the same group, have shown that cocaine-seeking behavior, maintained by cocaine-paired conditioned stimuli, has no effects on DA overflow in the accumbal core or shell, but that non-contingent presentation of these stimuli results in a regionally selective increase in DA overflow in the core (Ito et al. 2000). In contrast, operant responding for cocaine-associated conditioned stimulus is associated with increased extracellular DA in the dorsal striatum, whereas the non-contingent presentation of the cue had no significant effects on extracellular DA in this region of the brain (Ito et al. 2002). These observations, the authors argued, suggest that increased extracellular DA in the accumbal core might be implicated in the reinstatement of drug-seeking behavior, evoked by exposure to drug-associated cues, whereas increased DA overflow in the dorsal striatum may be implicated in the maintenance of habitual cocaine-seeking behavior. These conclusions are consistent with recent results, employing an optogenetic approach, which show that cue-evoked reinstatement of cocaine-seeking behavior seems to be associated specifically with stimulation of the indirect pathway from the accumbal core to VTA that passages through the dorsoventral pallidum (Stefanik et al. 2013).
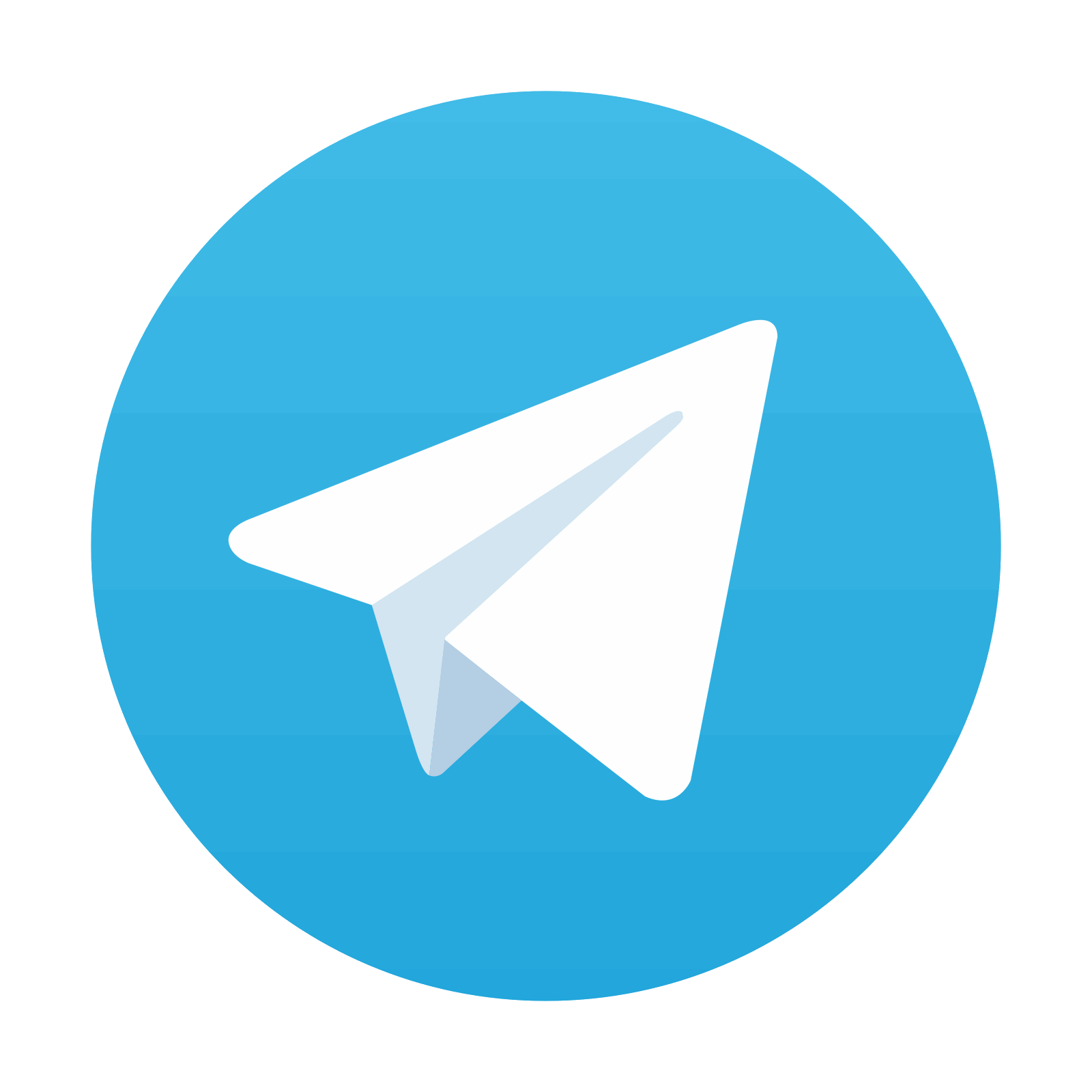
Stay updated, free articles. Join our Telegram channel

Full access? Get Clinical Tree
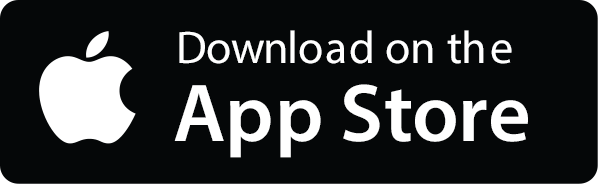
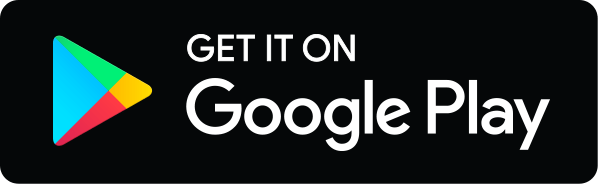