Fig. 25.1
Spontaneous switch from unstable to stable NREM sleep. 5-min polysomnogram snapshot of stage N2, showing the relatively abrupt switch from a state of respiratory event and arousals to a state with stable breathing and the absence of arousals
Is an Arousal Necessary to Enable Airway Opening and Respiratory Recovery?
The standard teaching is that an arousal from sleep was necessary for airway opening. There is new information that only a proportion of respiratory recovery is arousal dependent and that blood gas changes can drive airway opening without an electrocortical arousal [11]. The degree of arousal does amplify associated autonomic features roughly proportional to the degree of alpha/beta intrusion [12]. However, stage and state modulation are almost certainly important. It is conceptually useful to consider NREM as manifesting bimodal stable versus unstable propensities and characteristics. For example, when sleep drive is high, and NREM state is stable, airway opening can likely occur without a classic electrocortical arousal, the high sleep drive masking EEG arousal recognition or simply preventing an arousal, allowing brain stem and chemoreceptor or mechanoreceptor-based respiratory reflexes to open the airway. When NREM sleep is in an unstable as reflected by CAP EEG or low frequency cardiopulmonary coupling, the networked driving of sleep state couples nearly all prevalent physiology and pathology. Thus, tidal volume fluctuations, EEG arousals, heart rate change and hemodynamic/autonomic oscillations, and even periodic motor activation are all coupled and occur nearly simultaneously. During such a condition, it is hard to be sure arousal is necessary, but arousals certainly occur, in close temporal relationship, to respiratory recovery. K complexes are frequent accompaniments of respiratory recovery and may reflect the interplay and interaction between sleep disruptive and restorative forces. Medications that increase slow wave, such as atypical anti-psychotics, can obscure the faster components of arousals, when clusters of slow waves may be the only visually evident marker of respiratory-related arousal. During REM sleep, respiratory fluctuations occur as part of the REM sleep state and independent of arousals (increase in EMG tone), and it is unknown whether these tidal volume and flow fluctuations when exaggerated but without EMG increases disrupt REM sleep. When REM-without atonia is also present, respiratory-related arousal and REM sleep scoring can be difficult and sometimes impossible.
Event Cycle Characteristics
Event Cycle Length
The cycle length (Figs. 25.2 and 25.3) of a respiratory event reflects the time to arousal, respiratory drivers, and effects of phasic suppression of muscle tone for REM sleep events and intrinsic network properties of the sleeping brain. The time to arousal is dependent on the input traffic to brain stem arousal centers, which may be from within (respiratory effort), somatic afferents from the upper airway, or visceral afferents (including respiratory chemoreflex sensing of O2 in the carotid body, and CO2 in the carotid body and retrotrapezoid nucleus neurons).



Fig. 25.2
Long-cycle periodic breathing. 5-min polysomnogram snapshot. Cycle times are close to 50 s, in this patient with congestive heart failure

Fig. 25.3
Short-cycle periodic breathing. 5-min polysomnogram snapshot. Cycle times are about 30 s
Respiratory driving may be reactive (response to upper airway obstruction) or active, when the respiratory chemoreflex arc is hyperactive as in congestive heart failure (CHF) [13], at high altitude [14], and after exposure to hypoxia (sensory long-term facilitation) [15]. Phasic effects of REM sleep can cause obstructive, central-looking, or pseudoperiodic patterns, the latter when eye movements occur with similar inter-phasic interval. This latter phenomenon can be incorrectly characterized as periodic breathing, especially if EEG is not available, as in home sleep testing.
The intrinsic network properties of the brain reflect the interactions between multiple components including the cortex, thalamus, various output systems such as respiratory, autonomic, and motor, and the afferent return from the latter systems through baroreflex and chemoreflex arcs. On the surface EEG, this network property is represented by CAP. The CAP cycle length imposes an overall structure of the timing of pathological and physiological integrated multi-component repeats.
Event cycle length is entrainable. The best examples are high-altitude (short, less than 30 s, often 15–20 s) and CHF (long, most characteristically 60 s or longer) cycles. Adaptive ventilation can convert a short cycle to a long cycle; I have never seen this therapy convert a long to a short cycle. Respiratory cycle length, the time from one point of an event to a corresponding point, e.g., peak recovery to preak recovery, can also lengthen with supplemental oxygen or use of a non-vented mask to minimize hypocapnia [16], by prolonging the time to arousal. It is plausible that other rhythmic and repetitive arousing stimuli such as experimental auditory arousals can entrain “CAP,” but there will be biological constraints on frequencies which can entrain sleep state. These biological constraints in the frequency of coupled multicomponent oscillations are determined by the network properties of the sleeping brain. Too frequent arousing stimuli will wake up the subject, but too infrequent stimuli will likely only transiently arouse the subject without entrainment to the cycle time of the arousing stimulus.
Event Cycle Timing
External driving of sleep respiration can occur with neurostimulators (e.g., vagal, nerve stimulator for epilepsy Fig. 25.4). These stimulation pulses will have a metronomic timing and can cause respiratory suppression with precise repeated timing. Outside experimental settings, metronomic auditory stimulation during sleep does not occur. Strong respiratory chemoreflex modulation, causing periodic breathing/CSR, is the most common cause of metronomic respiratory event timings usually accompanied by equally impressive (consistent timings) oximetry, heart rate, and blood pressure oscillations.


Fig. 25.4
Pseudoperiodic breathing from external driving. 10-min polysomnogram snapshot. Patient using a vagal nerve stimulator for refractory epilepsy. The block-like chin tone increase is a stimulation artifact. During the stimulation phase, there is respiratory suppression
Ultra-Short Respiratory Events
The typical respiratory event evolves over 30–40 s. A commonality of repeat duration is also shared by periodic motor activation and A-phases of CAP. However, when respiratory chemoreflex driving is strong in the setting of normal cardiac function, cycle times shorten and can be as short as 20 s. This pattern strongly resembles that seen at high altitude. Travelers to high altitudes often experience restlessness, frequent brief arousals, and unrefreshing sleep [17], at least in part due to periodic breathing. In contrast to heart failure, the cycle times are 15–25 s, characterized by 2–5 large tidal volume breath clusters followed by apneas of 5–15 s in duration. There is a marked increase in arousals and reduction in slow-wave sleep likely related to hypoxia-induced carotid body sensitization narrowing the PaCO2 reserve and increasing controller gain [18]. A male predominance has been reported [19]. In idiopathic CSA, similar to high altitude, cycles of central apneas are shorter (20–40 s) and not as gradual and smooth as in classical CSR [20, 21]. While 10 s is the standard minimum event length for scoring, shorter events with biological consequences should reasonably be tagged.
Ultra-Long Respiratory Events
Two types of long events are seen. In one pattern, there is prolonged and progressive flow limitation that is terminated by an arousal; this pattern is typical of the pattern called upper airway resistance syndrome. Though this entity has been folded into obstructive sleep apnea, the pattern is distinctive enough. The second pattern is seen in patients with CHF—long-cycle (>60 s) periodic breathing/CSR. One consideration when the event duration is long is that the apnea–hypopnea index/respiratory disturbance index (AHI/RDI) may underestimate severity as there are fewer but longer events.
Assessing Event Significance in Stable NREM Sleep—Periods of Stable Breathing
Respiration is stable during conventional slow-wave sleep. Though described from the early days of recognition of the clinical sleep apnea syndrome, the mechanisms have remained elusive. The state of the cortical sleep network seems important. Increased genioglossus tone and increases in CO2 occur during the periods of stable breathing [8], with overt hypoventilation and hypoxia if flow limitation is severe. These respiratory features are more likely when obesity or reduced cardiopulmonary reserve is present. In the pediatric age group, obstructive hypoventilation is a common pattern during slow-wave sleep. Spontaneous periods of stable breathing periods do not occur during REM sleep. However, a number of NREM-dominant apnea syndromes, including idiopathic central sleep apnea, periodic breathing, high-altitude sleep apnea, complex apnea/treatment-emergent central sleep apnea, and opiate-induced sleep apnea, show markedly improvements in stability during REM relative to NREM sleep. Metabolic control of respiration and CO2 dependence on respiratory drive predominates during NREM sleep only.
The clinical problem with associating stable breathing phenomenon exclusively with slow-wave sleep by any definition is that such stable periods occur predominantly in N2, including those with no N3. Figure 25.5a–d demonstrates the impact of these periods in creating stable periods as a component across a dynamic range of pathology. Stable periods dominate the rebound effects of successful PAP titration, and the majority of time is usually N2. Some clues to the nature of this phenomenon can be gained from the concept of NREM sleep bimodality. The first clue came from the description of CAP and non-CAP from Italian researchers in the mid-1980s [22]. CAP and non-CAP periods occur across NREM sleep; non-CAP occurs in N2 or N3 [2]. Subsequently, the autonomic and respiratory associations of CAP/non-CAP were described [13]. Finally, the description of the cardiopulmonary coupling technique showed that NREM sleep has bimodal characteristics in health and disease. High-frequency coupling and low-frequency coupling periods show spontaneous switching and in relation to delta power. That is, high-frequency coupling is associated with high delta power, non-CAP EEG, stable breathing, strong sinus arrhythmia, and blood pressure dipping [3]. Low-frequency coupling is associated with unstable breathing (reaching scorable thresholds of sleep apnea in disease), cyclic variation in heart rate, CAP EEG, and blood pressure non-dipping. Thus, stable breathing periods reflect natural integrated network states of the brain and are amplified and readily recognizable in sleep apnea patients as the two states are markedly different on visual inspection. Benzodiazepines and related drugs increase non-CAP [23, 24] and may be expected to increase stable breathing periods. Zolpidem increases blood pressure dipping [25] and could do so through the induction of stable NREM periods.


Fig. 25.5
a Effect of CPAP on high loop gain sleep apnea. Same subject as in Fig. 5.3. CPAP opens the airway, but the underlying rhythm continues. b Effect of non-adaptive bilevel PAP on high loop gain sleep apnea. Same subject as in Fig. 5.3. The cycle times prolong, likely due to worsening of hypocapnia. Events during titration often show minimal oxygen desaturation, or even signal reductions reaching thresholds for hypopnea, and may take atypical long, short, or ataxic patterns. c Stabilizing effect of “stable NREM” sleep on sleep apnea. Same subject as in Fig. 5.3. Conventional stage is N3. d Stabilizing effect of REM sleep on high loop gain sleep apnea. Same subject as in Fig. 5.3
The proportion of stable breathing periods will impact the computed apnea–hypopnea index, as these periods do not contribute to the metric. Varying proportions of stable breathing on a night-to-night basis can contribute to night-to-night variability of the apnea–hypopnea index. Successful positive pressure titration markedly increases stable breathing periods on the treatment night and can provide a false sense of success; using the apnea–hypopnea index on a given pressure can be incorrect due to this confound. No current metric captures this pathology, though quantifying the % of flow-limited breaths will provide one measure and is available in some device-related automated analyses. The duty cycle of respiration (% inspiration of the respiratory cycle) will increase during these periods from prolonged inspiration [26]. Audible snoring or recordable upper airway vibrations are frequently noted. The pattern of flow limitation (the shape of the flow-limited breath) may have individual characteristics (similar within the same individual). Analysis of respiratory cycle-related EEG changes during such periods show an impact on electrocortical activity [9].
Assessing Event Significance in REM Sleep
The natural tidal volume fluctuations that occur in REM sleep, especially during phasic REM sleep, can mimic “real” apneas and hypopneas. Of special concern during positive pressure titration, brief events that are not associated with arousals or oxygen desaturation should probably be ignored unless they are actual obstructive apneas.
NREM Versus REM Dominance
For practical purposes, periodic breathing and hypocapnic central apnea do not occur in REM sleep (exception, a patient with CHF who demonstrates periodic breathing during wake state). NREM dominance is well described in idiopathic central sleep apnea [27], periodic breathing associated with heart failure or stroke [28], opiate-induced sleep apnea [29], and high-altitude periodic breathing [30]. NREM dominance is also a feature of complex apnea/treatment-emergent central sleep apnea, regardless of the exact definition used [31].
The simplest method is the NREM versus REM AHI/RDI, but the following are confounds: (1) Body position: Only supine-to-supine comparisons are fair; (2) stage and state of sleep: Periods of stable breathing, which may be associated with N2 or N2 sleep, contribute little to the AHI/RDI, while REM sleep does not have a similar stable equivalent. Determining NREM dominance can be difficult in those with substantial obstruction, where two distinct patterns can coexist—periodic breathing during NREM sleep and obstructive events during REM sleep. The use of PAP usually clarifies the situation—REM disease is readily eliminated, while NREM disease is not.
Ataxic Respiration
When there is continuous variability of rate, volume, and rhythm of breathing during NREM sleep (nearly looking like respiration in REM sleep), often admixed with obstructive or central apneas of variable duration, the term may be used. The events may be so short, and the respiratory abnormality is devoid of predictable fluctuations that the standard rules may over score or underscore events. Opiate use is the most common cause [32, 33], but any brain stem neurological abnormality, such as multiple sclerosis, brain stem stroke, and craniovertebral junctional anomalies, may also exhibit these features. Opiate-associated ataxic breathing (Fig. 25.6) is usually accompanied by mild hypercapnia, and in general, ataxic breathing is sensitive to reductions in CO2 by increasing ventilation such as by applying continuous PAP (CPAP).


Fig. 25.6
Ataxic respiration from opiate use. 5-min polysomnogram snapshot. Note (1) relatively mild oxygen desaturation; (2) short but variable length central apneas; (3) arousals and periodic motor activation
Loop Gain—A Concept Relevant to Understanding Scoring Approaches and Limitations
Breathing during NREM sleep is under metabolic/automatic control and can be understood through interaction between its components: controller, plant, and mixer. The “controller” converts the signal from the chemoreceptors into a ventilatory drive for the plant (an anticipated ventilation for a given PaCO2). The “plant” refers to the lungs, blood, and the tissues where CO2 is stored. It is the effector of the controller that produces the change in PaCO2 for a given change in ventilation stimulus. Its performance depends on mechanical and biochemical properties of its components (e.g., lung compliance, ventilation–perfusion matching, hemoglobin concentration). For most of the awake individuals, eupneic PaCO2 ~40 mmHg and for sleeping individuals, it is ~45 mmHg. The “mixer” represents the time it takes for the capillary blood from the lungs to reach the chemoreceptors and is primarily a function of cardiac output.
Sensitivity (chemosensitivity) of each of the system’s components to a change in input dictates how responsive the entire system is. This responsiveness has been termed “gain” or (▲ output/▲ input), an engineering term used to describe how stable or unstable a system is. The higher the gain, the less stable the system is. The controller gain is the (▲ Ve/▲ PaCO2), and plant gain (▲ PaCO2/▲ Ve), and the mixing gain determines the speed with which changes in PaCO2 and PaO2 from plant are detected by the controller [34]. The overall gain of the system (loop gain, Ve disturbance/Ve production) determines the stability of the system and is the product of controller, plant, and mixing gains. Loop gain varies between individuals and with disease states [35]. High loop gain is found in systems with large swings in blood gases and ventilation, while low loop gain indicates a stable system but also one that may also not respond adequately to a change in ventilation.
The metabolic respiration control system is exquisitely sensitive and designed to maintain PaCO2 homeostasis rather than oxygenation. Two levels of PaCO2, the apneic and eupneic thresholds, are critical to its operation. As elegantly demonstrated by Bulow in the 1960s [36–38], apneic threshold represents a level of PaCO2 below which breathing stops. Eupneic threshold is defined above. Below eupneic threshold, ventilatory drive is reduced, while above it is increased [39]. During sleep, the apneic threshold is usually 2–6 mm Hg below the eupneic sleeping PaCO2 level, but only 1–2 mmHg below the wakefulness eupneic PaCO2 level [40, 41]. This small difference between awake eupneic and sleep apneic PaCO2 is one reason behind instability of the respiratory system during sleep. When loop gain is too high, periodic breathing, hypocapnic central apneas, and NREM dominance of sleep apnea emerge. When loop gain is too low, hypoventilation results. High loop gain protects respiration during REM sleep and may explain why NREM-mixed periodic breathing with obstruction improves or “disappears” during REM sleep.
Quantitative polysomnogram analysis has also been used to differentiate cardiac-related periodic breathing from non-cardiac causes and may help predict effectiveness of CPAP for CSA-CSB. In brief, loop gain can be determined from the ratio of ventilation length (VL) divided by the apnea length (AL) or (VL/AL). VL/AL > 1 [42] and cycle duration/length (CL = VL + AL) of > 45 s [43] are suggestive of CSA of cardiac cause. Loop gain (LG) can also be derived by the means of a duty ratio (DR = VL/CL) of periodic breathing, LG = 2π/(2πDR – sin2πDR) [44]. In a pilot study of 14 CHF-CSB patients, LG was significantly higher among the non-responders and value of >1.2 predicted lack of response to CPAP in 7/7 patients [44]. Although simple to determine and encouraging from pilot data, such findings are limited to patients with already clear periodic breathing and should be validated in a larger, prospective manner. Table 25.1 summarizes some of the differentiating features of obstructive and chemoreflex driving of respiratory events.
Polysomnographic feature | Relatively pure OSA | Chemoreflex-modulated SA |
---|---|---|
Periodic breathing, Cheyne–Stokes | Rare | Typical (often short cycle, <30 s in the absence of CHF) |
Respiratory event timing | Variable (each event tends to have different durations) | Self-similar/metronomic |
Severity during sleep state | Greater severity in REM | Minimal severity in REM |
Effort signal morphology | Well maintained during obstructed breath | Complete or partial loss between recovery breaths |
Flow–effort relationship | Discordant: Flow is reduced disproportionately to reduction in effort | Concordant: Flow and effort follow each other in amplitude |
Arousal timing | Early part of event termination | Crests event, often in the center of the sequence of recovery breaths |
Oxygen desaturation | Irregular, progressive drops, V-shaped contour | Smooth, symmetric, progressive drops rare |
Response to continuous positive airway pressure | Normalization of flow, sleep, oxygenation, and ventilation | Persistence or “emergence” of central apneas or periodic breathing |
Assessing Pathological Respiratory Chemoreflex Activation: Cheyne–Stokes Respiration and Central Hypopneas
The AASM scoring manual requires the following for scoring a CSR event: cycle length of 40 s or greater, a typical concordant waxing–waning of flow and effort, a minimum of 3 cycles with these characteristics for any single CSR event, and an overall CSR index of ≥5/h of sleep. The ICSD-3 specifies that these should make up ≥50 % of all scored events. Central hypopnea scoring is “optional” in the scoring standards, yet recognition of CSR is recommended.
The events that make up CSR are essentially strings of central apneas and hypopneas. However, events at both sea level (often) and high altitude (always), and in patients with positive pressure induced or amplified respiratory instability, have short cycles that are less than 30 s. If 40 s is a requirement, then these short-cycle events will be falsely characterized as obstructive.
Flow limitation excludes a “central hypopnea” in the scoring manual, yet this idea has been conclusively shown to be false from the following data: (a) At high altitude, a pure chemoreflex form of sleep apnea, flow limitation occurs frequently; (b) heart failure patients with otherwise classic CSR can demonstrate flow limitation; (c) the airway can close during polysomnographic central apnea [45, 46]; and (d) central hypopneas demonstrate flow limitation [47]. In clinical practice, snoring can be seen in association with CSR—but usually occurs associated with the arousal, while in more purely obstructive event, the snoring occurs during the event and transiently resolves during the arousal.
Recognition of CSA syndromes generally requires a full-night recording of standard polysomongraphy with special attention to inspiratory effort. Cardiopulmonary recordings may also be sufficient, with the limitation of inability to differentiate REM from NREM-dominant disease, which has diagnostic and therapeutic implications. The current gold standard to determine whether apnea is central (no inspiratory effort throughout the event) is the use of esophageal balloon catheter [48, 49]. Contrary to current clinician perceptions and practice, a survey of 799 patients who underwent esophageal balloon placement during their sleep study revealed that 85 % felt minimal, if any, discomfort and only 3 % of patients refused the catheter on second occasion [50]. Attempts at using “less invasive” techniques such as respiratory inductive plethysmography or strain gauges, as well as pulse wave artifacts in the oronasal flow signal, produce unreliable results [51, 48]. Pulse transit time measurements can provide supportive information regarding central genesis. The lack of esophageal balloon data complicates the detection of central hypopneas even further. The exact threshold of when effort is adequate versus not is also somewhat arbitrary. Alternative strategies, using PSG-based algorithms, have been developed, but show only marginal accuracy (68 % in one study) [52].
A consistent feature of hypocapnic, heightened chemoreflex-mediated central apnea is predominance of events during NREM sleep, especially during non-slow-wave stages [7, 28, 53–55, 56, 57]. A metronomic self-similar appearance (identical event length and morphology in a consecutive series of events; consecutive hypopneas appear like clones of the preceding and following ones), even if individual events fulfill obstruction criteria, is typical, except in opiate-induced CSA, where variability of expiratory phase is characteristic. In contrast to hypocapnic CSA, in OSA and in many cases of hypercapnic CSA, the disease worsens markedly during REM sleep, especially if the motor neurons of the diaphragm are involved [34, 54]. Notable exceptions are congenital central hypoventilation syndrome (CCHS) and opioid-induced CSA, where the disease is worse in NREM sleep.
Treatment-Emergent Central Sleep Apnea (TE-CSA) and Complex Sleep Apnea
The PaCO2 reserve is labile during NREM sleep [58] and arousals due to maladaption to PAP can occur and drive instability [31]. The compliance or pressure at which passive critical closing of the upper airway occurs (P crit) shows overlap between patients with OSA and controls [59]. Variations in P crit alone account for only a portion of variations in the AHI [60] or differences in phenotype [61].
In some patients with OSA, central apneas and periodic breathing emerge during the initiation of CPAP. This phenomenon is called treatment-emergent CSA in the ICSD 3rd edition and defined when there are 5 or greater central apneas and hypopneas per hour of sleep making up greater than 50 % of all respiratory events during titration of CPAP in those fulfilling obstructive apnea criteria during diagnostic polysomnography. The ICSD tries to clear some of the controversies over “complex sleep apnea” [62]. The existence of TE-CSA apnea as a unique entity has been controversial in the sleep world. The original description [58] noted a set of relatively unique features on diagnostic polysomnography and an incomplete treatment response that included induction of central apneas or persistent periodic breathing when CPAP was applied. The key feature is NREM-dominant central hypopneas or periodic breathing with obstruction, resolving spontaneously during REM sleep. That is, induction of central apneas was not required. A subsequent publication “defined” complex apnea proposing the currently deployed ICSD criteria [62], which also became the criteria used by medical insurance to qualify patients for the more expensive adaptive ventilators.
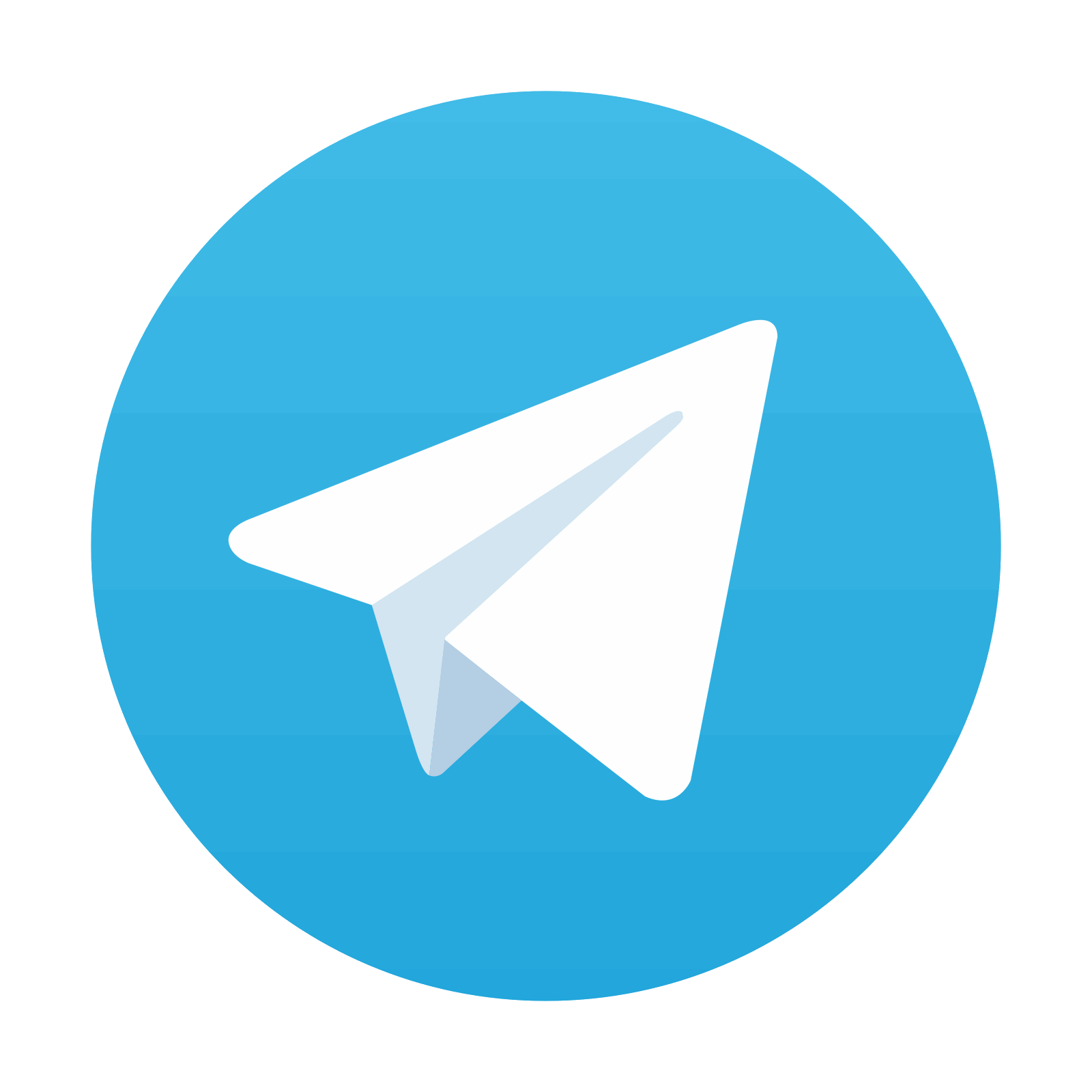
Stay updated, free articles. Join our Telegram channel

Full access? Get Clinical Tree
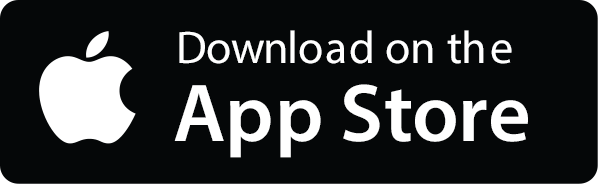
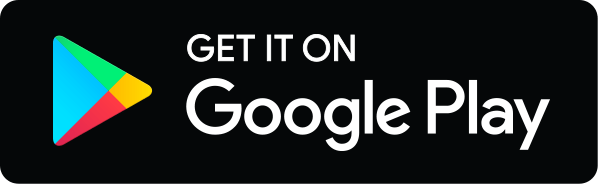