Screening of New Compounds and the Role of the Pharmaceutical Industry
H. Steve White
Roger J. Porter
Harvey J. Kupferberg
Introduction
The search for new antiepileptic drugs (AEDs) requires the appropriate choice of a number of models for the identification of new therapeutic agents for the treatment of epileptic seizures. The animal models used in the search of new anticonvulsant drugs are varied and have been the subject of several recent reviews.50,88,97,102,104,107,111,201
Different approaches can be used in the discovery of new therapeutic agents for the treatment of seizure disorders. The model choice can be specific for a seizure type, such as complex partial,44,104 absence,24,163,194 and status epilepticus (SE).94,196 It can be based on a mechanism of action, such as decreased neural excitability33 or enhanced inhibition.103 Alternatively, a seizure model in which the compound blocks the final expression of the experimental seizure can be used; for example, a “mechanism-independent” model such as the maximal electroshock seizure (MES).179,181
Several restrictions and conditions are inherent in the choice of model for identifying a new drug. It would be ideal and more efficient if the model were capable of screening large numbers of compounds without requiring large amounts of any one compound. Neither should the complexity and technical expertise required for the development and implementation of such a model be difficult.
Validation is a criticism often raised about new mechanistic seizure models. In the validation process, the new model must be evaluated against current, clinically effective therapeutic agents. The question then arises as to whether a model that is capable of identifying clinically effective compounds whose mechanisms are known will be able to identify unique compounds.
A second question that often arises is whether a given model reflects the pathophysiologic processes and symptomologies of the epilepsies. Currently, a number of model systems are highly predictive of efficacy against generalized tonic–clonic seizures (e.g., the MES test), generalized absence or spike-and-wave seizures (e.g., the genetically absence epileptic rat of Strasbourgh, the Lh/Lh mouse, γ-hydroxybutyrate induced spike-wave seizures), and partial seizures (e.g., the kindled rat).
No single approach can be used to identify an effective compound for the treatment of epileptic seizures. Furthermore, no ideal animal model would effectively identify a drug active against all forms of seizures, regardless of its mechanism of action. Therefore, new approaches must be undertaken for the discovery of new therapeutic agents.
In this era of molecular biology, when targets have become important in drug development, target validation using in vivo animal models is appropriate. As unique structural compounds are identified using basic screening procedures, they should be immediately evaluated in molecular target screening procedures. The reverse of this process should also occur.
When lead compounds are identified, additional information must be obtained before the development process begins. These include the establishment of a compound’s pharmacologic and toxicologic profile and in vitro studies designed to ascertain proposed mechanisms of action. Other parameters such as absorption, distribution, excretion, metabolism, and drug–drug interactions must also be characterized.
Loscher et al. have published seven critical reviews dealing with the role of technical, biologic, and pharmacologic factors in the laboratory evaluation of anticonvulsant drugs. These seminal reviews should be read before undertaking the task of evaluating new chemical entities for their potential in the treatment of seizures. The reviews discuss in detail the influence of administration vehicles on seizure threshold107; the nuances of the various MES seizure models97 and pentylenetetrazol (PTZ) seizure tests102; and the calculation and utility of protective indices106; the consequences of seasonal influences on amygdala kindling,204 MES, PTZ,99 and mouse models of generalized seizures.100
The objective of the initial part of this chapter is to discuss the historical background of the discovery and utilization of seizure models, then describe those models and methods that have direct applicability to the discovery of new anticonvulsant drugs and their mechanisms of action.
Historical Perspective
Prior to the discovery and development of phenytoin, anticonvulsant compounds such as phenobarbital and bromides were used for the treatment of seizures in patients without any preclinical experimental information. Although methods for producing seizures were known, Merritt and Putnam130 first used a systematic approach in evaluating the potential of a series of compounds as antiseizure agents (see White for review and references199).
They studied the ability of a series of chemicals to raise convulsive threshold by using graded electrical stimulation in cats. They knew that phenobarbital was a clinically effective compound in treating seizures, whereas other barbiturates were comparatively ineffective or toxic (e.g., produced narcosis). A number of Parke Davis compounds were submitted for evaluation. Six were analogs of phenobarbital. A few were hydantoins, one of which was 5,5 diphenylhydantoin (diphenylhydantoin, phenytoin).
Several significant observations were made. They found that diphenylhydantoin, acetophenone, and several phenones and ketone derivatives had an anticonvulsant effect equivalent to that of phenobarbital, but without producing sedation.
The majority of compounds evaluated by this procedure were inactive.
The majority of compounds evaluated by this procedure were inactive.
They also found that carbon dioxide raised seizure threshold when hypercapnia was observed. For example, it was impossible to produce convulsions with 40 milliamperes of current. When animals were allowed to breathe air for a few moments, the seizure threshold returned to normal. This result predicted the use of carbonic anhydrase inhibitors in treating seizures.
During the 1940s and 1950s, new compounds were synthesized, and structure-activity relationships (SARs) were investigated for anticonvulsant and hypnotic activity.3,8 This approach, using methodology similar to that of Merritt and Putnam, gave rise to mesantoin, nirvanol, trimethadione, methylphenylsuccinimide, and phenacemide. Because these compounds are known to be chemically similar to phenyt-oin, their therapeutic potential was not unexpected. Vida summarized the anticonvulsant activity of a large number of compounds.195
During the mid 1940s to early 1950s, a number of papers appeared describing the properties of electrically induced seizures and the effect of chemical agents on them. Two basic mechanisms were proposed to explain how anticonvulsant drugs prevented seizures: by raising seizure threshold or by preventing seizure spread. Once the seizure threshold was exceeded by more than 20%, the pattern and duration of the seizure was relatively constant and independent of the intensity or duration of the stimulus.187
No matter whether a seizure was chemically or electrically induced, the drug effect on seizure pattern would be modified in an identical manner. Therefore, agents that inhibit seizure spread would attenuate the extensor tonic component of the seizure, independent of how the maximal seizure is produced. Toman and Goodman186 summarized the “state-of-the-art” methodology for identifying new therapeutic agents. They concluded that a single test could adequately define the anticonvulsant properties of a drug.
Chen and Ensor20 determined the anticonvulsant activity of nine known drugs by testing in both an electrical and chemical model. They then compared the results with the known clinical utility of these same drugs. The two methods ranked the potency of the drugs in a similar manner. This was done to see if similar qualitative and quantitative data could be obtained by using two methodologies. By 1951, phenytoin had opened a new era for the treatment of generalized tonic–clonic seizures.
The discovery of trimethadione and paramethadione for the “petit mal triad” soon followed. An animal model for evaluating therapeutic agents for the treatment of partial seizures was lacking. Toman et al.185 described a “psychomotor” test in which mice are subjected to low-frequency stimulation to produce seizures resembling those seen in humans. A rectangular pulse of 6 Hz, 1 msec. pulse width, and a current of 32 mA is delivered for 3 seconds via electrodes placed on the cornea. The mice display an abnormal behavior for 25 seconds, which can best be described as “stunned.” They remain motionless and do not respond to painful stimuli. These types of in vivo screening models are still used for the identification of new therapeutic agents for the treatment of seizures.
Animal Models for Drug Discovery
Genetic Models
Because of their seizure predisposition, genetic animal models of epileptic seizures have been used to identify and profile potential anticonvulsant compounds. These models are thought to mimic the clinical situation more closely than traditional electrically or chemically induced seizures in experimental animals.1,105 The genetic models include the photosensitive baboon (Papio papio)127,128; several strains of audiogenic seizure mice, such as the DBA/2,16 SJL/J,54 Frings, and O’Grady25; genetically epileptic prone rats, GEPR-3 and GEPR-935,93; Mongolian gerbil (Meriones unguiculatus)101,113,116; chickens74; tottering mice (tg/tg strain)64; and dogs.112
Audiogenetic Mice
Audiogenic seizure–susceptible mice exhibit wild running followed by generalized tonic seizures when exposed to high-intensity sound. The intensity of sound usually is in the range of 90 to 120 dB with frequencies between 11 and 16 kHz. Seizure susceptibility in some stains is enhanced if the mice are exposed to high-intensity sound following a priming stimulus.25 Strain difference in seizure susceptibility occurs with age, usually reaching a maximum level between 2 and 4 weeks of life.154 These seizure-prone mice have been used to identify a number of different types of central nervous system (CNS)-active agents.
The Frings mice maintain their susceptibility to sound stimulation over their entire adult life. Swinyard described similar activity in both Frings and O’Grady strains of mice for phenytoin, phenobarbital, and trimethadione.180 Unfortunately, non-anticonvulsant drugs will also attenuate sound-induced seizures. Ataraxic agents, muscle relaxants, chlorpromazine, and reserpine will attenuate the sound-induced seizures of Frings mice in nontoxic doses.140
Several experimental compounds were evaluated for anticonvulsant activity using the Frings strain of audiogenic seizure–susceptible mice. MDL 27,266 was found to have similar values in the Frings and DBA/2 strains, with ED50s of 5.0 and 5.1 mg/kg, respectively.200 Valrocemide (TV-1901), a valproic acid analog, was found to be more potent than valproic acid when administered intraperitoneally to Frings mice.69
The most commonly used strain of audiogenic seizure mice is the DBA/2. Chapman et al.16 reviewed the literature for the use of DBA/2 mice in evaluating the pharmacologic activity of known anticonvulsant drugs. All of the commonly used anticonvulsant drugs, such as benzodiazepines, γ-aminobutyric acid (GABA) agonists, GABA-transaminase inhibitors, GABA-uptake inhibitors, competitive33,136 and noncompetitive excitatory amino acid antagonists,17,18,19 and calcium entry blockers,40 were active in suppressing sound-induced seizures in DBA/2 mice.
Several currently marketed anticonvulsants have been profiled for anticonvulsant activity in the DBA/2 strain. Pregabalin’s anticonvulsant activity was profiled in a number of animal models. Its anticonvulsant ED50 in DBA/2 mice was 2.7 mg/kg following oral administration.193 Felbamate produced dose-dependent effects for suppression of sound-induced tonic, clonic, and wild-running phases using ED50s of 23.1, 48.4, and 114.6 mg/kg, respectively.39 Retigabine was found to be active in many of the rodent models. The ED50 in the DBA/2 is 2.3 mg/kg following intraperitoneal administration.147
El Mouse
The El mouse is an electroencephalographically authentic animal model of epilepsy.155,176,177 Seizures are induced by vestibular stimulation and become spontaneous after the mice experience several (10–15) induced seizures. The seizures appear to originate in the hippocampus or deep temporal lobe structures and are characterized by excessive salivation and automatism of the head and limbs. Paroxysmal electroencephalographic discharges are seen in the temporal lobe. King and LaMotte87 published a comprehensive review of the El mouse as a model
of focal epilepsy. These seizures can be inhibited by phenytoin at 40 mg/kg twice daily for 3 days.119
of focal epilepsy. These seizures can be inhibited by phenytoin at 40 mg/kg twice daily for 3 days.119
Fueta et al. studied the antiepileptic action induced by a combination of vigabatrin and tiagabine in the El mouse.52,53 They used brain slices from the El and the control ddY mouse and found that no synergistic effects took place. An α-amino-3-hydroxy-5-methyl-4-isoxazole propionic acid (AMPA) antagonist, YM928, in doses of 5 mg/kg or 10 mg/kg, administered intraperitoneally, significantly increased the number of stimulations required to elicit generalized seizures.207 The novel anticonvulsant compound BW534U87, which is active in several electrically and chemically induced seizure models, is also active in preventing the focal seizures in the El mouse. The anticonvulsant activity is partially reversed with a selective adenosine A1 receptor antagonist.166 Competitive N-methyl-D-aspartate (NMDA) antagonists have been shown to inhibit NMDA whole-cell currents in mouse spinal neurons as well as to block seizures in a dose-dependent manner in the El mouse.34
Several of the clinically effective anticonvulsant drugs, including ethosuximide, inhibited seizures induced by the intravenous administration of pentylenetetrazol.174 The results were compared to those obtained by original sensory-induced seizures. The authors felt that PTZ-induced seizures were a more precise measure of anticonvulsant activity in the El mouse.
Tottering (tg/tg) and Quaking Mouse
The tottering mouse exhibits bilaterally synchronous spike-and-wave (6–7 Hz) discharges132,133 that are sensitive to drugs used to treat absence seizures in humans.64 The discharges are accompanied by movement arrest, fixed stare, twitching of the vibrissae, and myoclonic jerks of the head and jaw. Behaviorally, this genetic strain appears to exhibit both simple partial seizures with motor symptoms and absence seizures. Microscopically, there appears to be cellular loss and vesiculations of the cytoplasmic membranous structures in the cerebellum. The quaking mouse, like the tottering mouse, has an autosomal recessive genetic disorder that produces tonic–clonic seizures.
The homozygous mice exhibit tonic–clonic seizures when lifted by the tail and slowly rotated 180 degrees; approximately 85% of the control mice handled in this manner exhibit seizures. Drugs used to treat human absence seizures, such as ethosuximide, are ineffective in this seizure model, whereas drugs for the treatment of generalized tonic–clonic seizures and partial seizures are effective.184
The clinically effective anti-absence drugs diazepam, phenobarbital, and ethosuximide were effective against the absence-like seizures in the tottering mouse.64 In contrast, phenytoin did not significantly reduce the incidence of the absence seizures in doses up to 60 mg/kg. The CNS-stimulant caffeine, in doses of 5 to 15 mg/kg, significantly decreased absence-like seizures 4 hours following intraperitoneal administration.90
The quaking mouse, like the tottering mouse, has an autosomal recessive genetic disorder that produces tonic–clonic seizures. The homozygous mice exhibit tonic–clonic seizures when lifted by the tail and slowly rotating them 180 degrees. Carbamazepine and phenytoin were effective in controlling the seizure, whereas ethosuximide and diazepam were ineffective. Valproate completely controlled the seizure when a dose of 400 mg/kg was administered.184 Tonic–clonic convulsions in this genetic species were attenuated by both competitive and noncompetitive NMDA antagonists when administered by the intracerebroventricular route of administration.131
Unfortunately, most genetic models of spontaneous seizures are presently impractical as a means of identifying potential candidates in primary screens due to the limited availability of test animals and/or irregular seizure frequency.110
Audiogenetic Rats
Two separate and distinct colonies of genetically epilepsy-prone rats, GEPR-9 and GEPR-3, have been bred and are used to identify anticonvulsant drugs.35 The two colonies differ in the convulsion intensity following a sound stimulus. The GEPR-9 rats exhibit a seizure pattern similar to the DBA/2 mice, characterized by a terminal complete tonic phase. The seizure pattern of the GEPR-3 consists of an initial running phase followed by clonic seizures. Changes in sound pressure and frequency are important factors to be considered when establishing this model in a laboratory.183
Several neurochemical deficits occur in the GEPR that may have human correlates. Both noradrenergic (NE) and serotonergic deficits appear to be important in the seizure disposition of the GEPR. Browning et al. found that a NE deficit greatly enhances the incidence of tonic convulsions and supports the hypothesis that an increase in excitatory neurotransmitters in the GEPR inferior colliculus may act to initiate audiogenic seizures, whereas the NE deficit may allow expression of the tonic components of the seizure seen in some GEPR.12
The GEPR-9 is extremely sensitive to electrically induced seizures.13 The threshold current (mA) needed to produce tonic seizures in the GEPR-9 is one-fourth that of the GEPR-3 or normal rat. In fact, forelimb or facial clonus could not be elicited in the GEPR-9 at any current. A complete description of the ontogenetic development, neurochemistry, and seizure disposition of the GEPR has been summarized by Jobe et al.71
All the established AEDs attenuate the sound-induced seizures in the GEPR. In 1997, Consroe and Wolkin26 compared the anticonvulsant profile of phenytoin, cannabidiol, delta 9 tetrahydrocannabinol, and cannabinol to clinically effective anticonvulsants in both the MES test and GEPR rats. They found that cannabidiol was relatively more potent than phenytoin. In general, phenytoin and carbamazepine, on a mg/kg basis, are more potent in blocking the tonic phase in the GEPR-9 than in attenuating the clonic seizure in the GEPR-3. Phenobarbital and ethosuximide are, however, equally potent in both strains of rats.35
Valproate is more potent in the GEPR-3 than the GEPR-9. Antidepressants such as imipramine and amitriptyline act both as anticonvulsants and proconvulsants in these models. This dichotomy can be explained by the fact that antidepressants and antiarrhythmics lower the seizure threshold while at the same time preventing seizure spread.
NMDA receptor antagonists significantly decreased AGS severity in the GEPR-9 rat. Systemic administration of the competitive NMDA antagonists 3-[(±)-2-carboxy-piperazin-4-yl]-propyl-1-phosphonate (CPP) and 2-amino-7-phosphonoheptanoic acid and the noncompetitive antagonist dizocilpine (MK-801) were effectively anticonvulsant in the GEPR-9 rat.38
The selective neuronal GABA uptake inhibitor NNC-771 has been found to protect against both the clonic and tonic seizure phases at doses of 0.23 mg/kg following intraperitoneal administration.175 Bilateral injections of tiagabine, a GABA uptake inhibitor, into the inferior colliculus of GEPR-9 rats significantly reduced seizure severity.48 These results suggest that GABA is an important inhibitor of acoustical transmission in this brain structure.
Epileptic Fowl
Seizure susceptibility in domestic fowl (Gallus gallus) is due to a homozygous autosomal recessive trait.29 Spontaneous seizures occur at the time of hatching and continue to occur throughout the bird’s life. Seizures can be induced by photic stimulation using an optimal frequency of 14 flashes per second.30 Heterozygotes do not respond to the stimulus. The seizures are
characterized by an upward and backward tonic extension of the head and neck, followed by loss of leg muscle control. A final phase consists of violent wing flapping with clonic leg movements. The interictal electroencephalogram (EEG) is characterized by high-voltage, slow-wave activity.
characterized by an upward and backward tonic extension of the head and neck, followed by loss of leg muscle control. A final phase consists of violent wing flapping with clonic leg movements. The interictal electroencephalogram (EEG) is characterized by high-voltage, slow-wave activity.
Seizure incidence and severity was evaluated following the administration of several of the clinically effective anticonvulsant drugs. Phenobarbital, primidone, phenytoin, and valproic acid reduced seizure susceptibility at plasma concentrations approximating those considered within the therapeutic range for controlling generalized and focal seizures in humans.36,73,74,75,76,77 Ethosuximide was the only ineffective anticonvulsant in this model.36
Several experimental GABA mimetic compounds, GABA-selective transport inhibitors, and tetrahydrocannabinol were also active in attenuating the induced seizures.73,153,206 Pedder et al.137 demonstrated that both competitive (CPP and APH) and noncompetitive NMDA antagonists (MK-801) delayed the onset of seizures in a dose-dependent manner. An antiparasitic agent, ivermectin, attenuated photic stimulation-induced seizures in a dose-dependent manner.31
Light-sensitive Epileptic Baboon
Killam et al.86 found 60 of 100 baboons (Papio papio) exhibited paroxysmal responses to the first exposure of flashing light. Intermittent light stimulation (25 Hz) produces a characteristic EEG and motor paroxysmal response. In some animals, self-sustained epileptiform discharges appeared prior to the motor seizures.85 Myoclonus of the eyelids, face and neck, trunk, and limbs characterized the motor seizures. The effect and duration of drugs can be evaluated in a dose-response relationship by scoring the severity of the seizure pattern. The administration of a subconvulsant dose of allylglycine (200 mg/kg intravenously) 2 to 9 hours prior to the photic stimulation produces a stable level of sustained generalized myoclonic responsiveness.128
A number of clinically effective and experimental anticonvulsants have been evaluated in this model of epilepsy. Benzodiazepines and phenobarbital are extremely effective in attenuating the light-induced epileptiform seizures.85,128,138 GABAergic compounds produce mixed responses. Valproate partially attenuated seizures at high, clinically therapeutic plasma concentrations,125 whereas muscimol and 4, 5, 6, 7 tetrahydroxyisoxazolo (4, 5, c) pyridine 3-ol (THIP) are proconvulsant.124,138 Vigabatrin completely blocked the generalized myoclonus for several hours.126 Phenytoin, carbamazepine, and ethosuximide are relatively ineffective in attenuating the myoclonic seizures.127,172
Electrically Induced Seizures
Maximal Seizure Pattern Test
Electrically induced seizures are perhaps the most frequently used model system for the identification of anticonvulsant activity. The type and intensity of the seizure is related to the intensity of the stimulus current and site of stimulation.139 The electrical stimulus can be delivered via the cornea or ear clips. As the current is increased, the behavioral characteristics become more severe. The lowest effective current produces a “stunned response.” Higher currents produce “twitching of the vibrissae” and facial clonus. The behavioral responses continue to proceed to forelimb clonus and rearing. Finally, a current is reached that produces tonic flexion-extension of the fore- and hind-limbs.
The change in threshold current to produce tonic hind-limb extension in 50% (CC50) of the rodents can be used as a primary screen for anticonvulsant activity. Loscher et al.97 demonstrated that ethosuximide was the only anticonvulsant that did not raise the threshold for tonic seizures. Phenytoin and carbamazepine tripled the current required to produce a tonic hind-limb seizure. Diazepam, valproic acid, and primidone were far less active in raising the CC50. It is therefore not surprising that these less potent drugs are relatively poor in preventing seizure spread in the supramaximal seizure pattern (MES) test.
It is also important to characterize a compound’s effect on raising seizure threshold. In the early studies, a large number of animals were required to determine the CD50 for a given population. Loscher then described a direct cortical ramp-stimulation model to determine the changes in CD50 in rats following administration of known anticonvulsant drugs. This methodology allows for repeated determination of seizure threshold at short-time intervals in individual rats without inducing postictal threshold changes.91 The changes in current needed to produce hind-limb extension using this method for carbamazepine, phenytoin, and valproate were similar to the earlier methods using a large population of animals. Thus, this approach offers distinct savings in animal costs and requires less compound.
When groups of mice are used to establish changes in seizure threshold, a “staircase” procedure can be used to define the population threshold.49 The stimulus intensity of the next animal is determined by the response of the animal just tested. The stimulus intensity is increased to the next higher increment if the previous animal failed to exhibit a seizure or the next lower increment if the animal exhibits a seizure.178 Using this information, the investigator can then calculate the current necessary to produce a seizure in 50% of the population; that is, the CC50.
The MES test is used to identify drugs that prevent seizure spread, and it is often employed as a primary screen for anticonvulsant activity. Phenytoin and carbamazepine are extremely active in this animal model. The electrical current for the supramaximal MES test in the CF1 mouse is 50 mA at 60 Hz for 0.2 seconds. This is delivered via corneal electrodes,179,188 and is four to five times greater than the threshold current needed to produce a tonic hind-limb extension seizure.
The choice of mouse strain is extremely important in the MES test. When evaluating the strain, the CD97 is usually determined first, then the final current for the test can be estimated. For the supramaximal test, a current that is three to five times that of the CC97 is typically used. Because death doesn’t usually occur in the CF1 male mouse following the tonic phase of the seizure,189 this strain has been historically employed by the NINDS-sponsored ADD Program at the University of Utah.202 In contrast, approximately 10% of young rats and a higher percentage of older rats may fail to display a tonic extension at a supramaximal stimulation (e.g., 150 mA, 0.2 sec.).
In most cases, electroshock stimulation is delivered through corneal electrodes, although other stimulation sites can be used. For example, transauricular stimulation in rats also produces a similar seizure pattern.11 There are, however, differences in some seizure parameters. Transauricular stimulation is more effective in eliciting tonic convulsions, whereas facial and forelimb clonus is lost. The threshold for clonus is lower when transcorneal electrodes are used.
Direct electrical stimulation to various brain structures produces the identical behavioral responses seen following corneal stimulation. The studies of Esplin et al.45,46 demonstrated that direct electrical stimulation of the spinal cord produces a seizure pattern similar to that seen following corneal stimulation. These observations suggest that the spinal cord serves as a conduction pathway for impulses generated from higher brain levels. Phenytoin had a minor effect on the tonic-extensor phase of the spinal cord–induced seizure. The anticonvulsant activity of phenytoin therefore must be due to its action on higher brain centers. Conversely, phenytoin, phenobarbital, and ethosuximide block the tonic hind-limb extension component of seizures induced by stimulation of the mesencephalic reticular formation of rats.21
Febrile Seizures
Febrile seizures are commonly observed in children; depending on their severity, the patient may be treated prophylactically with phenobarbital. Unfortunately, phenobarbital has been associated with negative effects on learning and behavior. Several animal models have been developed and validated for the purpose of identifying nonsedating anticonvulsants for the treatment of febrile seizures. Holtzman et al. developed a microwave-hyperthermia model that raises core temperature of 2- to 10-day-old rats and results in convulsions similar to febrile convulsions in human infants.67 In 1983, Hjeresen et al., using similar microwave technology, used 13- to 17-day-old rats to produce seizures. This heating procedure impaired neither brain growth nor performance during behavioral testing. There appears to be a decrease in seizure susceptibility with age in the rat pups following microwave hyperthermia.66
Similar studies were conducted using chicks that display a genetic epileptic seizure phenotype. Epileptic seizures are produced by elevating their body temperature using microwave diathermy.72 These hyperthermic seizures differ from those seen in photo-stimulated, epileptic-prone chickens. Hyperthermia does not produce seizures in adult epileptic chickens. Seizures in hyperthermia-induced epileptic chicks are attenuated by phenobarbital, whereas valproic acid and phenytoin have no effect.72
Hyperthermia can be produced in weanling rats (21 days old) by immersion in a 45°C water bath for a maximum of 4 minutes (four exposures over a 2-week period). The rat’s seizures increase in severity and progress to a stage 5 generalized seizures.135 This method was used to characterize the anticonvulsant activity of several drugs: clonazepam, MK801, valproic acid, remacemide, and phenobarbital. Remacemide and phenobarbital were inactive. Valproate actively decreased seizure severity and modestly attenuated seizure duration. It also reduced the number of animals experiencing seizures on test day one. Clonazepam effectively lowered seizure score and reduced the number of animals experiencing seizures during three of the four testing periods. MK801, at the dose used, caused behavioral side effects while reducing the maximum seizure grade.
Baram et al. were interested in the long-term outcome of febrile seizures in infants and young children.2 They developed a methodology to produce hyperthermic-induced seizures using the infant rat. The seizures were induced by a regulated stream of mildly heated air over 1- to 11-day-old rats; the presence of seizures was confirmed by behavioral and EEG analysis. Stereotypical seizure activity was observed in 94% of the heat-treated rat pups, with 11% mortality. Depth recording from the amygdala and hippocampus clearly showed epileptic activity. Thus, this model allows for long-term studies of the mechanisms and outcome of febrile seizures.
In their initial studies, hyperthermic seizures—but not hyperthermia alone—resulted in numerous argyrophilic neurons in discrete regions of the limbic system. Within 24 hours of seizures, a significant proportion of neurons in the central nucleus of the amygdala and in the hippocampal CA3 and CA1 pyramidal cell layer were affected. However, by 4 weeks after the hyperthermic insult, no significant neuronal damage was evident in these regions.191
Chemically Induced Seizures
The use of chemicals to induce seizures began over a century ago and is still used in epilepsy research and drug discovery today.37,173 Camphor, picrotoxin, and strychnine were used before the turn of the 20th century. The most commonly used chemoconvulsant, PTZ, was introduced in 1926.65
In addition to PTZ, other commonly used chemoconvulsants include bicuculline, picrotoxin, γ-hydroxybutyrate, NMDA, AMPA, kainate, and quisqualate. Other ictal- or seizure-producing compounds mentioned in the literature, but less frequently used in drug discovery, include pilocarpine, homocysteine/cobalt, flurothyl, 3-mercaptoproprionic acid, allylglycine, β-carboline, penicillin, zinc, alumina, and tetanus toxin.37
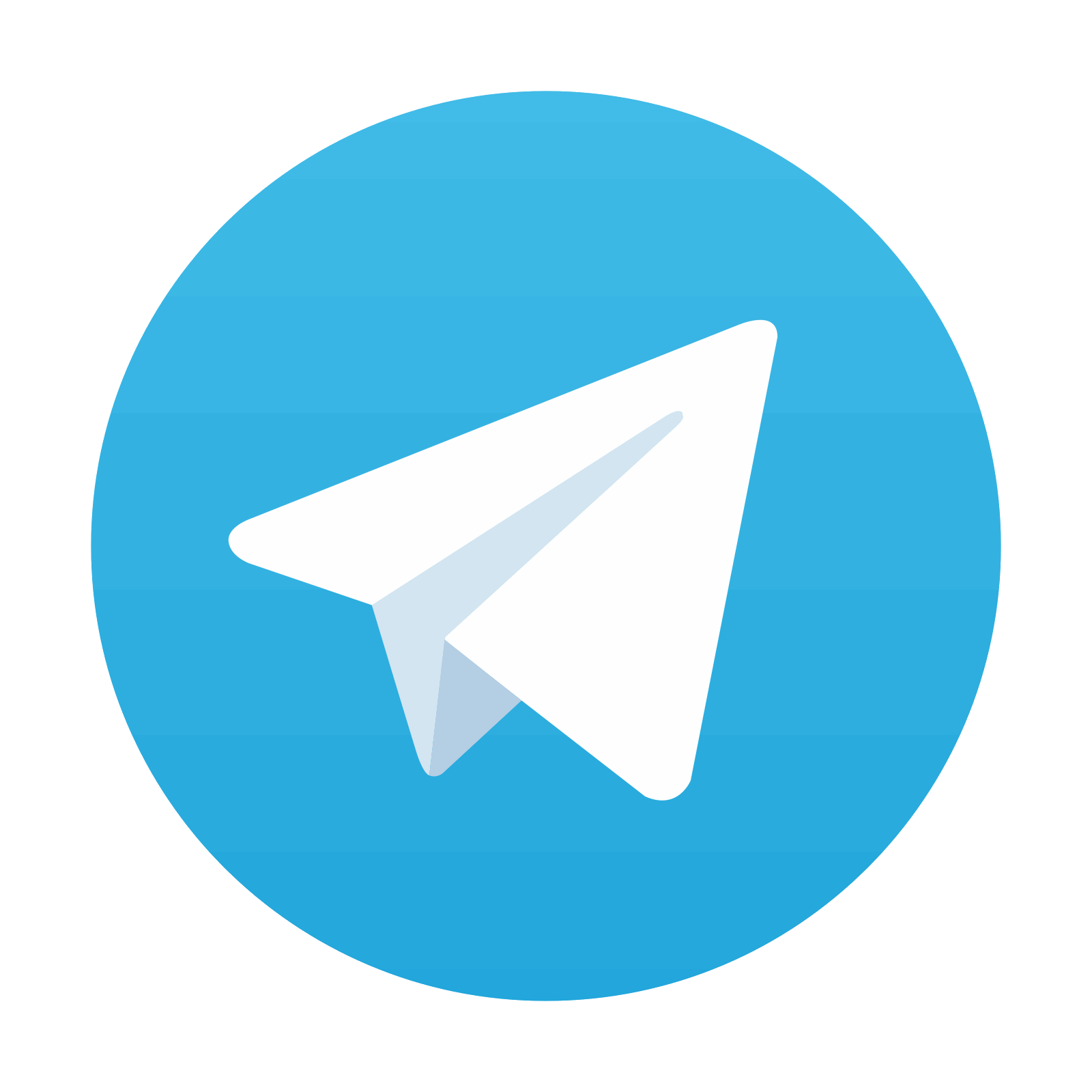
Stay updated, free articles. Join our Telegram channel

Full access? Get Clinical Tree
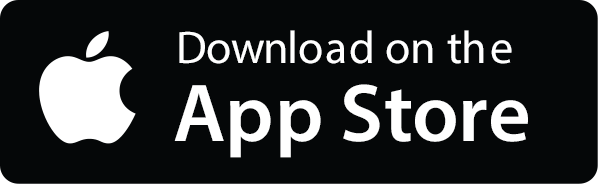
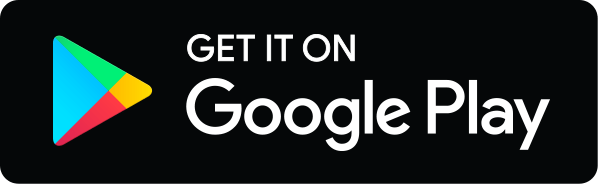
