Sexual Differentiation of the Nervous System
Genes and Hormones Determine Physical Differences Between Males and Females
Chromosomal Sex Directs the Gonadal Differentiation of the Embryo
Gonads Synthesize Hormones That Promote Sexual Differentiation
Sexual Differentiation of the Nervous System Generates Sexually Dimorphic Behaviors
A Sexually Dimorphic Neural Circuit Controls Erectile Function
A Sexually Dimorphic Neural Circuit Controls Song Production in Birds
A Sexually Dimorphic Neural Circuit in the Hypothalamus Controls Mating Behavior
Environmental Cues Control Some Sexually Dimorphic Behaviors
Sexual Dimorphism in the Human Brain May Correlate with Gender Identity and Sexual Orientation
FEW WORDS ARE MORE LOADED WITH meaning than the word “sex.” Sexual activity is a biological imperative and a major human preoccupation. The physical differences between men and women that underlie partner recognition and reproduction are obvious to all of us, and their developmental origins are well understood. In contrast, our understanding of behavioral differences between the sexes is primitive. In many cases their very existence remains controversial, and the origins of those that have been clearly demonstrated remain unclear.
In this chapter we first briefly summarize the embryological basis of sexual differentiation. We then discuss at greater length the behavioral differences between the two sexes, focusing on those differences or dimorphisms for which some neurobiological basis has been found. These dimorphisms include physiological responses (erection, lactation), drives (maternal behavior), and even more complex behaviors (gender identity). In analyzing these dimorphisms we will discuss three issues.
First, what are the genetic origins of sexual differences? Human males and females have a complement of 23 chromosomal pairs, and only one differs between the sexes. Females have a pair of X chromosomes (and are therefore “XX”), whereas males have one copy of the X chromosome paired with a Y chromosome (XY). The other 22 chromosome pairs, called autosomes, are shared between males and females. We will see that some genetic determinants arise from the presence of a Y chromosome, while others arise from sex-specific patterns of autosomal gene expression that exert their impact during development.
Second, how are differences in genes and gene expression translated into differences between the brains of men and women? We will see that key intermediates are the sex hormones, a set of steroids that includes testosterone and estrogens. These hormones act during embryogenesis as well as postnatally, first organizing the physical development of both genitalia and brain regions, and later activating particular physiological and behavioral responses. Hormonal regulation is especially complex because the nervous system, which is profoundly influenced by sex steroids, also controls their synthesis. This feedback loop may help to explain how the external environment, including social and cultural factors, can ultimately shape sexual dimorphism at a neural level.
Third, what are the crucial neural differences that underlie sexually dimorphic behaviors? Clear physical and molecular differences between the brains of men and women have been found. These differences imply that neural circuitry differs between the sexes, and in a few cases these distinctions in connectivity are directly related to behavioral differences. In other cases, however, sexually dimorphic behaviors appear to result from differential usage of the same basic circuits.
Before proceeding we must define the usage of two words that are commonly confused with each other: sex and gender. As a descriptor of biological differences between men and women, the word sex is used in three ways. First, anatomical sex refers to overt differences including the differences in the external genitalia as well as other sexual characteristics such as the distribution of body hair. Gonadal sex refers to the presence of male or female gonads, the testes or ovaries. Finally, chromosomal sex refers to the distribution of the sex chromosomes between females (XX) and males (XY).
Whereas sex is a biological term, gender encompasses the collection of social behaviors and mental states that typically differ between males and females. Gender role is the set of behaviors and social mannerisms that is typically distributed in a sexually dimorphic fashion within the population. Toy preferences in children as well as distinctive attire are some examples of gender roles that can distinguish males from females. Gender identity is the feeling of belonging to the category of the male or female sex. Importantly, gender identity is distinct from sexual orientation, the erotic responsiveness displayed toward members of one or the other sex.
Are gender and sexual orientation genetically determined? Or are they social constructs molded by cultural expectations and personal experience? As the examples in this chapter will illustrate, we are still far from untangling the contributions of genes and environment to such complex phenomena. However, our recognition that genes and experience interact to shape neural circuits gives us a more realistic framework with which to answer this question compared to our predecessors, who were constrained by the simplistic view that genes and experience acted in mutually exclusive ways.
Genes and Hormones Determine Physical Differences Between Males and Females
Chromosomal Sex Directs the Gonadal Differentiation of the Embryo
Sex determination is the embryonic process whereby chromosomal sex directs the differentiation of the gonadal sex of the animal. Surprisingly, this process differs in fundamental ways within the animal kingdom and even among vertebrates. In most mammals, including humans, however, an XY genotype drives differentiation of the embryonic gonad into testes, whereas an XX genotype leads to ovarian differentiation. Hormones produced by the testes and ovaries subsequently direct sexual differentiation of the nervous system and the rest of the body.
It is the presence of the Y chromosome rather than the lack of a second X chromosome that is the crucial determinant of male differentiation. This was first evident in rare individuals born with two or even three X chromosomes in addition to a Y chromosome (XXY or XXXY). These individuals are men who exhibit male-typical traits. In fact, female cells do not have two active X chromosomes. Early in embryogenesis one of the two X chromosomes in each female cell is chosen at random for inactivation and the genes on it are rendered transcriptionally silent. Thus both male and female cells have a single active X chromosome and male cells also have a Y chromosome.
The sex-determining activity of the Y chromosome is encoded by the gene SRY (sex-determining region on Y) whose activity is required for masculinization of the embryonic gonads (Figure 58-1). Inactivation or deletion of SRY leads to complete sex reversal: Individuals are chromosomally male (XY) but externally indistinguishable from females. Conversely, in rare instances SRY translocates to another chromosome (to the X chromosome or an autosome) during spermatogenesis. Such sperm can fertilize eggs to produce individuals who are chromosomally female (XX) but externally male. However, such XX sex-reversed men are infertile, as many of the genes required for sperm function are located on the Y chromosome.
Figure 58-1 The role of the SRY gene in sex determination in humans. SRY, the sex-determining locus (blue domain), resides on the nonhomologous region of the short arm of the Y chromosome. The presence of SRY is determinative for male differentiation in many mammals, including primates and most rodents. Normally X- or Y-bearing sperm fertilize an oocyte to generate XX females or XY males, and the resulting phenotypic sex is concordant for the chromosomal sex. Rarely SRY translocates to the X chromosome or an autosome (not shown). In such cases XXSRY offspring are phenotypically male while XYΔSRY offspring (the Δ indicating a gene deletion) are phenotypically female. (Modified, with permission, from Wilhelm, Palmer, and Koopman 2007.)
How does SRY instruct the undifferentiated gonads to develop into testes? The female differentiation program appears to be the default mode; patterning genes prime the body and gonads to develop along female-specific pathways. The SRY gene encodes a transcription factor that induces expression of genes, some of which prevent execution of the default program and initiate the process of male gonadal differentiation. One of the best-studied targets of the SRY transcription factor is another transcription factor, SOX9, which is required for differentiation of the testes. SOX9 in turn activates a variety of genes required for formation of testicular Sertoli cells. Thus SRY initiates a cascade of inductive interactions that ultimately lead to male- specific gonad development.
Gonads Synthesize Hormones That Promote Sexual Differentiation
The chromosomal complement of the embryo directs sexual differentiation of the gonads and in turn the gonads determine the sex-specific features of the nervous system and the rest of the body. They do this by secreting hormones. Gonadal hormones have two major roles. Their developmental role is traditionally referred to as organizational because the early effects of hormones on the brain and the rest of the body lead to major, generally irreversible, aspects of cell and tissue differentiation. Later some of the same hormones trigger physiological or behavioral responses. These influences, generally termed activational, are reversible.
One example of an organizational role of gonadal hormones is seen in the differentiation of structures that connect the gonads to the external genitalia. In males the Wolffian duct gives rise to the vas deferens, the seminal vesicles, and the epididymis. In females the Müllerian duct differentiates into the oviduct, the uterus, and the vagina (Figure 58-2). Initially both female (XX) and male (XY) embryos possess Wolffian and Müllerian ducts. In males the developing testes secrete a protein hormone, the Müllerian inhibiting substance (MIS), and a steroid hormone, testosterone. MIS leads to a regression of the Müllerian duct and testosterone induces the Wolffian duct to differentiate into its mature derivatives. In females the absence of MIS permits the Müllerian duct to differentiate into its adult derivatives, and the absence of circulating testosterone causes the Wolffian duct to resorb. Thus the Y chromosome overrides a female default program to generate male gonads, which in turn secrete hormones that override a female default program of genital differentiation.
Figure 58-2 Sexual differentiation of the internal genitalia. Embryos of both sexes develop bilateral genital ridges (the gonadal anlagen) that can differentiate into either testes or ovaries; Müllerian ducts, which can differentiate into oviducts, the uterus, and the upper vagina; and Wolffian ducts, which can differentiate into the epididymis, the vas deferens, and the seminal vesicles. In XY embryos the expression of the SRY gene in the genital ridge induces differentiation of this tissue into testes and of the Wolffian ducts into the rest of the male internal genitalia, while the Müllerian ducts are resorbed. In XX embryos the absence of SRY permits the genital ridges to develop into ovaries and the Müllerian ducts to differentiate into the rest of the female internal genitalia; in the absence of circulating testosterone the Wolffian ducts degenerate. (MIS, Müllerian inhibiting substance.) (Modified, with permission, from Wilhelm, Palmer, and Koopman 2007.)
The action of MIS is largely confined to embryos, but steroid hormones exert effects throughout life—that is, they have activational as well as organizational roles. All of the steroid hormones derive from cholesterol (Figure 58-3). The sex steroids can be divided into androgens, which generally promote male characteristics, and the estrogens plus progesterone that promote female characteristics. The testes produce mostly the androgen testosterone, while the ovaries produce mostly progesterone and an estrogen, 17-β-estradiol. The menstrual cycle is a good example of the activational function of estrogen and progesterone.
A glance at the metabolic relationships among steroid hormones (Figure 58-3) reveals a surprise. The female hormone progesterone is the precursor of the male hormone testosterone, and testosterone is the direct precursor of the female hormone 17- β-estradiol. Thus the enzymes that convert one hormone to the other control not only the level of the hormone but also the “sign” (male or female) of the hormonal effect. Aromatase, the enzyme that converts testosterone to estradiol, is present at high levels in the ovaries but not in the testes. Differential expression of aromatase is the reason for sexual dimorphism in circulating testosterone and estrogen. Aromatase is also expressed in various regions of the brain (Figure 58-4A), and many of the effects of testosterone on neurons are thought to occur after its conversion to estrogen. Testosterone is also converted by the enzyme 5α-reductase into another androgenic steroid, 5α-dihydrotestosterone (DHT), in various target tissues, including the external genitalia. In these tissues DHT is responsible for induction of secondary male characteristics such as facial and body hair and growth of the prostate. Later in life DHT is the culprit in male pattern baldness.
Figure 58-4 Aromatase and estrogen receptors are expressed in specific regions of the brain.
A. The enzyme aromatase, which catalyzes the conversion of testosterone into estrogen (see Figure 58-3), is expressed in discrete neuronal populations in the brain. Aromatase labeled with a reporter protein (blue) in transgenic mice is shown here in three coronal planes of the brain: in neurons in the preoptic hypothalamus (1), in the bed nucleus of the stria terminalis or BNST (2), and in the medial amygdala (3). These areas contain sexually dimorphic neurons that regulate sexual behavior, aggression, and maternal behaviors. (Modified, with permission, from Wu et al. 2009.)
B. This midsagittal section of an adult rat brain shows binding of estrogen to cells in various hypothalamic regions, including the preoptic area, which is sexually dimorphic. Additional estrogen binding is seen in the septum, hippocampus, pituitary, and midbrain. Other, more lateral areas such as the amygdala (not shown) also contain estrogen receptors.
Figure 58-3 Steroid hormone biosynthesis. Cholesterol is the precursor of all steroid hormones and is converted via a series of enzymatic reactions into progesterone and testosterone. Testosterone or related androgens are obligate precursors of all estrogens in the body, a conversion that is catalyzed by aromatase. The expression of 5-α-reductase in target tissues converts testosterone into dihydrotestosterone, an androgen.
As one can imagine, mutations in genes encoding enzymes involved in steroid hormone biosynthesis have far-reaching consequences. The phenotypes dramatically illustrate both the organizational and activational effects of steroid hormones, as well as the difficulty of neatly distinguishing the two. Here we describe three disorders (Table 58-1).
The first, congenital adrenal hyperplasia or CAH, is a genetic deficiency in the synthesis of corticosteroids by the adrenal glands that results in overproduction of testosterone and related androgens. This condition is autosomal recessive and occurs once in 10,000-15,000 live births. In girls born with CAH, excess androgens lead to some masculinization of the external genitalia, a process called virilization. Virilization clearly reflects the organizational roles of steroids. This condition can be diagnosed at birth and resolved by surgical intervention. Treatment with corticosteroids reduces testosterone levels, permitting these females to undergo puberty and to be fertile.
A second genetic disorder, 5α-reductase II deficiency, can also affect sexual differentiation (Table 58-1). In male fetuses 5α-reductase II is expressed at high levels in the precursor of the external genitalia, where it converts circulating testosterone into DHT. The high local concentrations of DHT virilize the external genitalia. Clinical 5α-reductase II deficiency is inherited in an autosomal recessive manner, and males present at birth with ambiguous (under-virilized) or overtly feminized external genitalia. In many instances, therefore, chromosomally male patients (XY) with this condition are mistakenly raised as females until puberty, at which time the large increase in circulating testosterone virilizes the body hair, musculature, and, most dramatically, the external genitalia.
Steroid Hormones Act by Binding to Specific Receptors
The critical role of steroid receptors in controlling sexual differentiation is well illustrated by patients with a third disorder, complete androgen insensitivity syndrome or CAIS (Table 58-1). Testosterone, estrogen, and progesterone are hydrophobic molecules that are able to diffuse across cell membranes, enter the bloodstream, enter cells in many organs, and bind to intracellular ligand-specific receptors. The receptors for these hormones are encoded by distinct but homologous genes.
Table 58-1 Three Clinical Syndromes That Highlight the Role of Androgens in Masculinization in Humans
A single gene encodes a receptor that binds the androgens testosterone and DHT. The androgen receptor binds DHT approximately threefold more tightly than testosterone, accounting for the greater potency of DHT. There is also a single receptor for progesterone (progesterone receptor), whereas two genes encode receptors that bind estrogens (estrogen receptors (α and β). The estrogen receptors are present in many tissues of the body, including the brain (Figure 58-4B).
These receptor proteins are transcription factors that bind specific sites in the genome and modulate transcription of target genes. They contain several signature motifs, including a hormone-binding domain, a DNA-binding domain, and a domain that modulates the transcriptional activity of target genes (Figure 58-5A). Hormones activate the transcriptional activity by binding to the receptor. In the absence of ligand the receptors bind to protein complexes that sequester them in the cytoplasm. Upon binding of ligand the receptors dissociate from the complex and enter the nucleus, where they dimerize and bind to specific sequence elements in the promoter and enhancer regions of target genes, modulating their transcription (Figure 58-5B).
Figure 58-5 Steroid hormone receptors and their mechanism of action.
A. The canonical receptors for steroid hormones are ligand-activated transcription factors. These receptors have an N-terminal domain, which contains a transcriptional transactivator domain; a central DNA-binding domain; and a C-terminal ligand-binding domain, which may contain an additional transcriptional transactivator domain.
B. Sex steroid hormones are hydrophobic and enter the circulation by diffusing across the plasma membrane of steroidogenic cells in the gonads. They enter target cells in distant tissues such as the brain by passing through the plasma membrane and bind their cognate receptors. The steroid hormone receptor typically exists in a multiprotein complex with chaperone proteins in the cytoplasm of hormone-responsive cells. Ligand-binding promotes dissociation of the receptor from the chaperone complex and translocation into the nucleus. In the nucleus the receptor is thought to bind to hormone response elements as a homodimer to modulate transcription of target genes. (Modified, with permission, from Wierman 2007.)
Patients with CAIS are chromosomally XY but carry a loss-of-function allele of the X-linked androgen receptor that abolishes cellular responses to testosterone and DHT. Because the pathway of sex determination via SRY remains functional, these patients have testes. However, because of androgen signaling, the Wolffian ducts do not develop, the testes fail to descend, and the external genitalia are feminized. In adulthood most of these patients opt for surgical removal of the testes and hormonal supplementation appropriate for females.
Sexual Differentiation of the Nervous System Generates Sexually Dimorphic Behaviors
Sex-specific behaviors occur because the nervous system differs between males and females. These differences arise from a combination of genetic factors, such as components of the sex determination pathways, as well as environmental factors, such as social experience. In many cases both genetic and environmental inputs act through the steroid hormone system to sculpt the nervous system. Many anatomical instances of sexual dimorphism have been documented, including differences in the numbers and size of neurons in particular structures as well as differences in the pattern and number of synapses.
It is challenging to trace the chain of causality from environmental or genetic factors to the development of neural dimorphisms and to link these differences to sex-specific behaviors. In this section we examine a few cases in which studies in experimental animals have provided insights. In later sections we ask whether similar mechanisms underlie sexually dimorphic behaviors in humans.
However, before proceeding we note that the ways in which chromosomal mechanisms of sex determination are linked to the cellular processes of sexual differentiation in the central nervous system vary widely among species. In insects sex differences in behavior are independent of hormonal secretion from the gonads, and instead rely exclusively on a sex determination pathway within individual neurons. This mode of sexual differentiation of the brain and behavior is particularly well understood in the fruit fly, where it has been demonstrated that the sex determination cascade initiates expression of a single transcription factor, fruitless, that specifies the entire repertory of male sexual behaviors (Box 58-1).
A Sexually Dimorphic Neural Circuit Controls Erectile Function
The lumbar spinal cord of many mammals, including humans, contains a sexually dimorphic motor center, the spinal nucleus of the bulbocavernosus (SNB). Motor neurons in the SNB innervate the bulbocavernosus muscle, which plays an important part in penile reflexes in males and vaginal movements in females.
In adult rats the male SNB contains many more motor neurons than the female SNB. In addition, male SNB motor neurons are larger in size and have larger dendritic arbors, with a corresponding increase in the number of synapses they receive. Like the SNB motor neurons, the bulbocavernosus muscle is larger in males than females; it is completely absent in the females of some mammalian species. SNB motor neurons also innervate the levator ani muscle, which is involved in copulatory behavior and is also larger in males than females.
How do these differences arise? Initially the circuit is not sexually dimorphic. At birth male and female rats have similar numbers of neurons in the SNB and similar numbers of fibers in the bulbocavernosus and levator ani muscles. In females, however, many motor neurons in the SNB and many fibers in the bulbocavernosus and levator ani muscles die in early postnatal life. Thus this sexual dimorphism arises not by male-specific generation of cells but rather by female-specific cell death.
Perinatal injections of testosterone or DHT can rescue a significant number of the dying neurons and muscle fibers in the female rat. Conversely, treatment of male pups with an androgen receptor antagonist increases the number of dying neurons and muscle fibers. So at a deeper level we see that the dimorphism results from male-specific preservation of motor neurons and muscle fibers that would die in the absence of hormone.
Where does testosterone act to establish this structural dimorphism? Is it primarily a survival factor for the motor neurons, with muscle fibers dying secondarily because they lose their innervation? Or does testosterone act on muscles, which then provide a trophic factor to support the survival of SNB motor neurons? This issue has been examined in rats carrying a mutation of the androgen receptor (tfm allele) that reduces binding of ligand to 10% of normal. The receptor resides on the X chromosome, so all males that carry a mutant gene on their one and only X chromosome are feminized and sterile. For female heterozygotes, the situation is more complicated. As described earlier, one of the X chromosomes is randomly inactivated in each XX female.
Female heterozygotes are therefore mosaics: some cells express a functional androgen receptor allele, others the mutated allele. Each muscle fiber has many nuclei, so most bulbocavernosus muscle fibers in the heterozygous female express functional androgen receptors. Motor neurons have a single nucleus, however, so each neuron is either normal or receptor-deficient. If androgen receptors were required in the neuron, one would expect only receptor-expressing SNB motor neurons to survive, whereas if receptors were required only in muscles, one would expect surviving motor neurons to be a mixture of wild type and mutant.
In fact, the latter situation occurs, indicating that survival of SNB motor neurons does not depend on a neuron-autonomous function of the androgen receptor. Rather, these neurons receive a trophic cue from the androgen-dependent bulbocavernosus and the levator ani muscles (Figure 58-7A). These cues may include the ciliary neurotrophic factor (CNTF) or a related molecule, because mutant male mice lacking a CNTF receptor exhibit a decreased number of SNB motor neurons, typical of females.
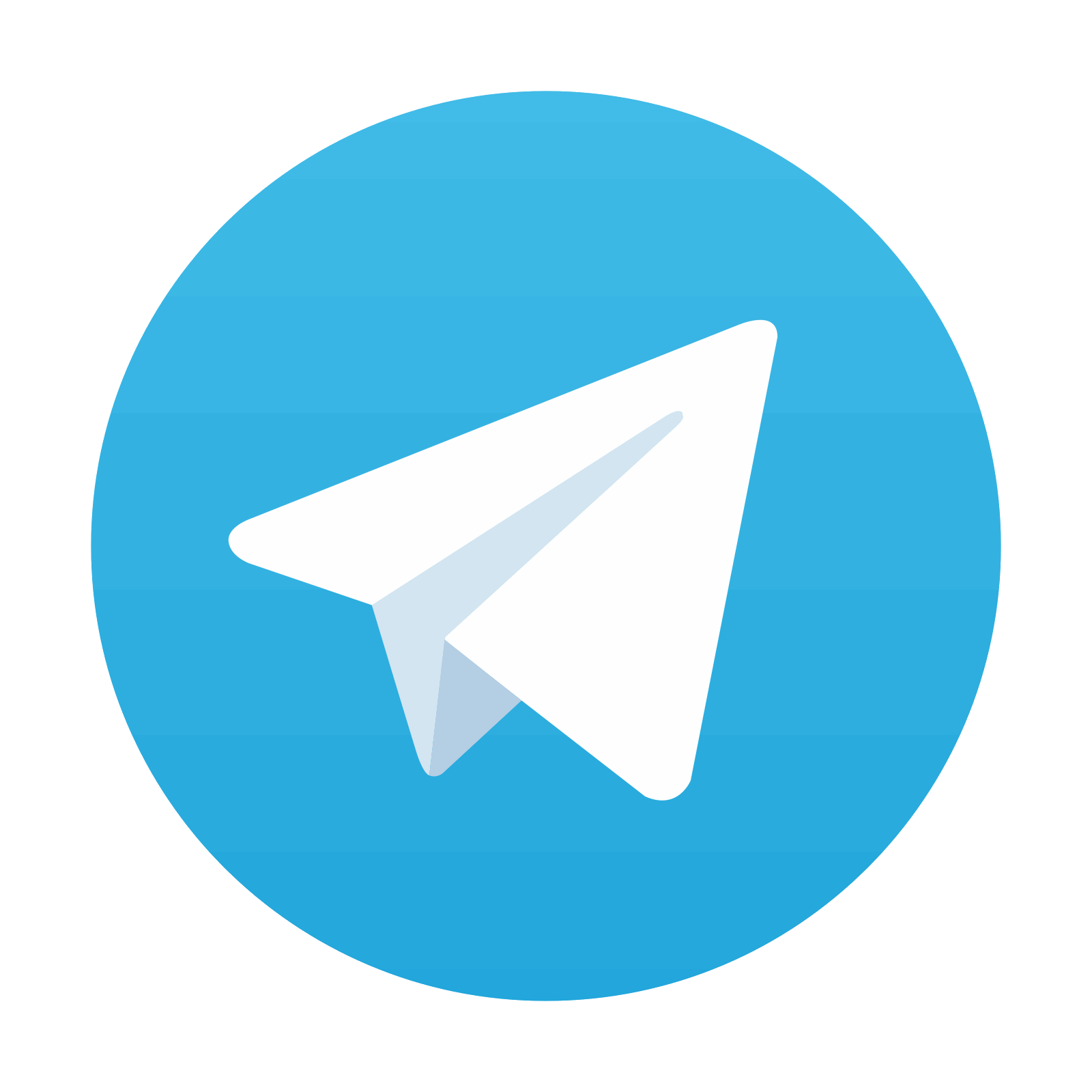
Stay updated, free articles. Join our Telegram channel

Full access? Get Clinical Tree
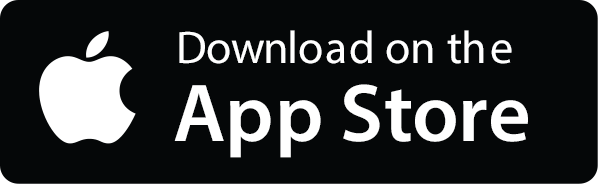
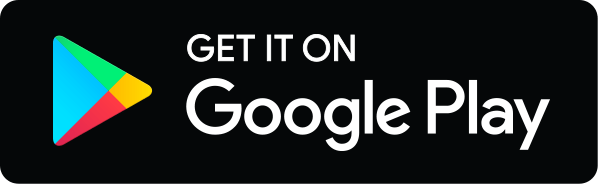