Shunt Infections
Hydrocephalus is the most common neurosurgical disorder managed by pediatric neurosurgeons and perhaps the one associated with greatest morbidity. In the United States alone, it has been estimated that there are between 38,200 and 39,900 admissions per year for pediatric hydrocephalus, accounting for more than 400,000 hospital days and costing between 1.4 billion and 2.0 billion dollars.1 Although in recent years there has been a resurgence of interest in alternative methods of treatment of hydrocephalus, including endoscopic third ventriculostomy and choroid plexus ablation, shunt devices continue to be the mainstay of hydrocephalus treatment. The complications and morbidity associated with the placement and maintenance of ventricular shunts are well documented in the neurosurgical literature, and in virtually all series the problem of shunt infection has been a significant feature. Although infection rates as low as 1.04% have been reported,2 rates between 3 and 15% are more representative of neurosurgical practice.3–9 Among children with shunt infection, a mortality rate of 10% has been reported10 furthermore, seizure disorders,11 cognitive impairment, and reduced academic achievement10,12 are more common in these children than in those who have not had infection. In addition to the clinical consequences of shunt infection, there is an economic burden, given that it is estimated that the hospital cost of treating a shunt infection is $50,000, making it the most costly implant-related infection in the United States.13
Although neurosurgeons have long recognized the importance of reducing shunt infection rates and numerous preventative strategies have been employed in pursuit of this goal, the strategies are institution-based, variably applied, and frequently unsupported by clinical evidence.14 Even in more recent series, between 4 and 12% of shunts become infected within 6 months of placement.15,16 In this chapter, the etiology of shunt infections is examined, and the contemporary understanding of the microbiology and pathogenesis of shunt infection is reviewed. Surgical management of shunt infection is discussed, and guidelines aimed at preventing or reducing shunt infection and its sequelae are presented.
77.1 Etiology and Pathogenesis
Infection results when a shunt becomes contaminated by an organism capable of establishing growth at the operative site, within the cerebrospinal fluid (CSF) and/or on the shunt hardware itself. Contamination may occur (1) at the time of shunt insertion because of contamination of the shunt tubing, CSF, or exposed wound; (2) through the wound during the early postoperative period before full skin integrity is re-established; or (3) after insertion by direct contamination (e.g., following shunt tap or by contiguous spread of abdominal infection).
An etiologic distinction is made between infections that begin as surgical wound infections enhanced by the presence of a biomaterial (external infections) and those that begin with colonization of the shunt itself (internal infections).17 From a clinical perspective, infections may be classified according to their location as superficial incisional, deep incisional (below the fascia, with or without extension to the external part of the shunt), or CSF (infection within the shunt, ventricle, or peritoneum). Unless stated otherwise, we have used the term shunt infection to indicate infection of the CSF.
77.2 Causative Bacteria
The majority of shunt infections are caused by staphylococci (gram-positive cocci). Staphylococci are divided into two groups on the basis of their ability to produce the enzyme coagulase and are easily differentiated in the laboratory by the coagulase (or other equivalent) test. Staphylococcus aureus produces coagulase and is therefore coagulase-positive. Most staphylococci do not produce coagulase, and these comprise the coagulase-negative staphylococci (CoNS). Where the CoNS have been accurately identified in human infection, Staphylococcus epidermidis is the most commonly found species. In almost all reported studies of shunt infection, CoNS are the most common infecting agents, accounting for at least half of cases. S. aureus accounts for a further quarter of cases.18–23 The remaining quarter of cases are due to gram-positive rods, other gram-positive cocci, and gram-negative rods (▶ Table 77.1). Propionibacteria are a not uncommon cause of shunt infection; however, the organisms can be difficult to isolate, and prolonged anaerobic culture is recommended.24
Organism | Shunt infections (%) |
Coagulase negative Staphylococcus | 60 |
Staphylococcus aureus | 18 |
Gran positive rods (C. diptheriae, P. acnes) | 10 |
Other gram positive cocci (Streptococcus, Micrococcus, Enterococcus) | 6 |
Gram negative rods (E.coli, Pseudomonas, Enterobacter) | 6 |
Data from references Kanev et al.1–10 |
The microbiological profile of shunt infections that present a year or more after shunt insertion is different. In these “late” infections, staphylococci are seen less often, and there is a predominance of gram-negative organisms. These infections are less likely to be related to the shunt insertion procedure (hence the lower preponderance of Staphylococcus species), and in as many as three-quarters of cases some additional event, such as peritonitis, hematogenous spread, or bowel perforation, can be identified as the precipitating factor.25
77.2.1 Source of Infecting Bacteria
It is clear that the majority of shunt infections are caused by gram-positive pathogens that are typical of the commensal skin flora, and an association has been demonstrated between perioperative skin bacterial density and the risk for shunt infection.22 Skin preparation (even with alcohol-based agents, which are more effective than aqueous agents) will kill the majority of skin surface organisms. However, some bacteria in the deeper layers of the epidermis and in hair follicles may survive this process, and indeed, in two studies in which wound swabs were taken during shunt operations, positive cultures were obtained in 37% and 58% of cases, respectively.26,27 Whether or not the organisms cultured from operative swabs are exclusively from the patient (as opposed to the surgeon or operative environment), and whether it is these specific organisms that will be responsible for subsequent shunt infection, remain controversial.
Shunt contamination may theoretically occur through the deposition of airborne organisms on shunt components or the wound; however, there is little evidence to assess the contribution of this route, and its role is not thought to be significant. With respect to the surgeon as a source of pathogens, in one study cultures from the surgeon’s gloves at the end of shunt surgery were positive for skin commensal bacteria in 39% of cases.22 In another study, breach of the surgeon’s gloves was identified in one-third of cases, and this was an independent risk factor for shunt infection in that series.28 Although the practices of double gloving, glove changing, and minimal shunt handling during surgery are supported by these findings, the evidence that commensal bacteria from the surgeon are an important source of shunt infection pathogens is weak. The patient’s own flora continue to be the most commonly cited source of shunt-infecting pathogens, although again, supporting evidence is far from conclusive. Seven of nine shunt infections studied by Bayston and Lari were due to organisms that had been cultured preoperatively from the patient,27 although whether the typing methods available at that time can be relied upon to establish that these were identical species might be debated. Shapiro et al found that only 20% of shunt infections could be related to cultures from the skin incision,23 and in a prospective study, Pople et al identified shunt infections in 3 of 30 infants younger than 6 months, although in only one of these were the authors confident that the organism originated in the patient’s flora.22 In the authors’ experience, intraoperative contamination has been clearly demonstrated; however, we were unable to show a clear association between intraoperative bacterial isolates and the organisms responsible for subsequent shunt infection.26 These apparently disparate findings might be explained by the suggestion that the surgical wound is contaminated by multiple strains of skin bacteria yet only a limited number progress to infection. Furthermore, in the days following surgery, as the commensal flora repopulates, the sutured surgical incision likely remains a potential portal of entry for bacteria.
77.2.2 Bacterial Factors
The mechanisms by which bacteria infect shunts have direct implications not only for our understanding of the treatment of shunt infection but also for the development of preventative strategies. Soon after placement of a shunt, there is a local tissue response that results in the deposition of host proteins over the tubing. Certain bacteria have surface-binding proteins or adhesins capable of binding directly to the shunt surface or to host proteins, thus facilitating bacterial adhesion to the catheter; this represents the first step toward shunt infection. It is now known that once bacterial adhesion has taken place, genetically driven metabolic changes occur within the bacteria. The bacteria become capable of producing an extracellular “mucoid” material rich in polysaccharides, glycolipids, and glycoproteins commonly referred to as “bacterial slime,” although now more properly referred to as polysaccharide intercellular adhesin or exopolymer.29 Colonies of bacterial cells are suspended within this exopolymer, and the resulting amalgam of cells and extracellular material, known as a biofilm, progressively coats the surface of the shunt tubing. The biofilm is a complex microenvironment in which there is an interaction between the infecting organism and the inflammatory host response. Additionally cell to cell signaling between bacterial cells results in expression of genes that further enhance their ability to produce exopolymer.29,30 The high failure rate of antibiotic therapy alone as a treatment for shunt infection has been attributed to the effect of the biofilm, which constitutes a physical barrier to the penetration of antibiotics. The reality is likely to be a far more sophisticated developmental strategy on the part of the bacteria. It is now known that early on in the establishment of the biofilm, additional phenotypic changes take place within the bacteria that modify some of the pathways that would previously have been targeted by the antimicrobials, thus further ensuring survival and propagation of the biofilm.31 This is important when treatment strategies are considered because organisms in an established biofilm are unlikely to be eradicated by antibiotics alone; hence the need to remove the shunt as part of the shunt infection treatment strategy.
These preliminary stages of shunt infection—initial bacterial adhesion and early proliferation of bacteria within the biofilm—represent potential targets for preventative strategies, and the recent generation of hydrogel-coated and silver- or antibiotic-impregnated shunts has been developed with this in mind.
Interestingly, the introduction of antimicrobial-impregnated shunts may have changed the demographic of shunt infection, with an apparent reduction in the incidence of CoNS shunt infections (▶ Table 77.2).
Organism | Historical cohort (1993–2003) 1592 shunts Infections = 133 (8.35%) | Bactiseal cohort 2003–2010 499 shunts Infections = 25 (5.10%) |
Coagulase negative staphylococci | 69 | 4 |
Staphylococus aureus | 42 | 10 |
Proprionibacteria | 2 | 4 |
Streptococcal/enterococcal sp. | 10 | 5 |
Gram negative | 5 | 2 |
Organism not isolated | 7 | 1 |
Data from Departments of Neurosurgery and Microbiology, Great Ormond Street Hospital for Children, London, UK. |
S. aureus organisms are additionally more likely to be responsible for incisional infections with or without involvement of the adjacent shunt because they produce a range of toxins and enzymes that allow them to survive the host cellular immune response. Therefore, S. aureus is more often responsible for those infections that begin with wound-related problems, such as wound breakdown, stitch abscess, and CSF leakage.
77.3 Timing of Shunt Infection
Shunt infection develops at a median time of 3 weeks (interquartile range, 1.5 to 5 weeks) after shunt surgery.32 Approximately 70% of shunt infections present within 2 months of shunt surgery, and 80% have presented by 6 months.33 This temporal association supports the assertion that shunt infection is essentially a surgical complication that many would argue is potentially avoidable by appropriate attention to surgical technique.2 The bacterial pathogenesis of acute shunt infection is discussed above. Shunt infections occurring 1 year or more after surgery are considered late infections and comprise up to 12% of cases.10 Both the causative bacteria and the underlying mechanism of shunt infection tend to be different. Two mechanisms of late shunt infection are to be considered. In the first mechanism, infection is likely initiated at the time of shunt insertion but is slow to manifest. Particularly indolent shunt infections, such as those caused by Propionibacterium acnes, typify this mode of infection. The causative organism may have been inoculated into the peritoneum at the time of surgery yet proliferates slowly, resulting in pseudocyst formation or less frequently ascites.34 The presentation in such cases reflects poor CSF absorption, causing abdominal distension or impaired distal drainage. The second mechanism of late shunt infection is due to a post-insertion event, such as appendicitis, erosion of the catheter into the colon, or abdominal surgery. An underlying cause is present in as many as three-quarters of cases, and although the source may not be immediately evident, an exhaustive search should be pursued. Less obvious candidates include hematogenous spread from distal sites, such as the ear, nose, throat, urinary tract, and lungs.25,35 Late infections can occur many years after shunt surgery, and it has been suggested that the risk for such late shunt infection is cumulative over time.25
77.4 Risk Factors for Shunt Infection
Numerous studies have sought to identify risk factors for the development of shunt infection; however, in many single-institution studies, the validity of the findings is compromised by small sample size and additional confounding factors, such as patient selection bias, variations in operative technique, and antibiotic prophylaxis. Inclusion criteria frequently differ between studies; some include only primary insertions, whereas others include revisions, and many studies relate to particular age groups or etiologies of hydrocephalus. Furthermore, some authors report infection rates per procedure rather than per patient, and as a result patient-related risk factors can be easily overlooked. During the following review of risk factors, it is important to take these issues into account because they may shed light on the apparently contradictory findings of some studies.
Pediatric patients with hydrocephalus are an extremely heterogeneous population, and although the following factors are among those that have been repeatedly identified to increase the risk for shunt infection, the risk to an individual patient will commonly reflect a complex interplay of more than one of these elements. Broadly speaking, one can usefully distinguish between risk factors that are intrinsic to the patient (or the patient’s underlying condition) and those that are extrinsic.
77.4.1 Intrinsic Factors
Age
Most studies concur that the risk for shunt infection is greater in children than in adults.36,37 Even after other variables have been accounted for, young age appears to be an independent risk factor for shunt infection.10,32 It is within the infant population that the effect of age is most marked. Kulkarni et al found that premature infants with a gestational age of less than 40 weeks at the time of surgery were at greater risk for shunt infection compared with older infants and children (hazard ratio [HR], 4.72; 95% confidence interval [CI], 1.71 to 13.06).28 In the study of McGirt et al, placement of a ventriculoperitoneal shunt in a premature neonate was associated with an almost fivefold increase in the risk for shunt infection (p < 0.1).38 Immunologic immaturity, greater density of the skin bacterial flora, and poor skin quality are among the reasons cited for the greater vulnerability of this group.22,39
Etiology of Hydrocephalus
The intraventricular hemorrhage of prematurity accounts for a significant proportion of cases of pediatric hydrocephalus, and recent studies continue to demonstrate that infection rates and early revision rates are particularly high in this group.15,40,41 All shunt-related complications tend to be particularly prevalent in patients with myelomeningocele compared with other etiologies, and shunt infection is no exception. Shunt infection rates as high as 25% are reported even in large centers10,42 for this group of patients. Because patients in this group have particularly high rates of shunt revision surgery, the “per patient” risk for shunt infection is also high. This propensity for shunt infection is most likely related to the need for an additional transdural neurosurgical procedure (i.e., closure of the neural tube defect) because the risk for shunt infection tends to decrease in subsequent shunt procedures. Shunt placement before closure of the myelomeningocele, a high level of the myelomeningocele, and a very large size of the ventricles (Evans index > 70) have all been correlated with increased shunt infection rates.43,44 When feasible, shunt placement is best deferred until after the myelomeningocele closure, and even then only after a critical appraisal of the need for shunt placement.45
Number of Previous Shunt Procedures
Even after controlling for other factors, Simon et al showed that the risk for shunt infection following revision surgery was significantly greater than the risk at the time of initial shunt placement (HR, 3.0; 95% CI, 1.9 to 4.7), and that the risk increased as the number of revisions increased (HR, 6.5; 95% CI, 3.6 to 11.4).32 A prior history of shunt infection also increased the odds of subsequent shunt infection.38,46,47 The risk factors for repeated infection are not well understood at present, although the risk is highest in the 6-month period following initial shunt infection, and it is important to distinguish between relapse of an inadequately treated initial infection and a new infection in the same patient.
Other Intrinsic Factors
Other intrinsic factors that have been reported to increase the risk for shunt infection include low birth weight,39,48 and comorbidities, such as chronic respiratory disease and sepsis.18
77.4.2 Extrinsic Factors
Perhaps of more practical relevance are those risk factors that are extrinsic to the patient and diagnosis because these variables, in theory at least, are amenable to modification in an attempt to reduce shunt infection rates.
Cerebrospinal Fluid Leakage and Wound Infection
CSF leakage and wound infection are inextricably linked. CSF leakage through the surgical wound provides a conduit by which bacteria can enter and infect the shunt; moreover, wound infection compromises the integrity of the closure, thus facilitating CSF leakage. Both these surgical complications have consistently been shown to result in increased rates of shunt infection. In the prospective study of risk factors for shunt infection conducted by Kulkarni et al, a postoperative CSF leak was the strongest risk factor (HR, 19.16; 95% CI, 6.96 to 52.91),28 a finding reinforced in a more recent prospective study, in which the incidence of shunt infection was 57.1% in patients with a CSF leak compared with 4.7% in those with no leak.49 The importance of meticulous wound closure and diligent wound care in the postoperative period cannot be overemphasized.
Surgeon Experience
The influence of surgical experience on shunt infection rates has been specifically addressed in a number of studies, and most conclude that more experienced surgeons tend to have lower rates of shunt infection.18,33,50,51 However, surgical grade does not necessarily correlate with shunt infection rates,49 and operative numbers are perhaps a more relevant index. In a study of more than 12,000 shunt procedures performed by 254 surgeons, Cochrane and Kestle observed an infection rate of 7% for surgeons whose shunt procedure numbers were above the 50th percentile and of 9.4% for those whose numbers were below the 50th percentile.51
Operative Factors
Numerous studies have sought to demonstrate a relationship between various operative factors and the development of shunt infection. These include, among others, an increased risk associated with hair shaving,5 intraoperative use of the endoscope,38 excessive numbers of personnel in the operating room,52 position of the case in the operating schedule,2 and increased duration of surgery.20,52 Many of these studies, however, are retrospective single-institution audits and insufficiently powered; thus, the correlations identified may not be causative. One prospective study did identify repeated exposure of the shunt hardware to breached surgical gloves as a significant risk factor.28
Although the role of airborne pathogens in the etiology of shunt infection remains unproven, the use of high-efficiency particulate air (HEPA) filters in the operating environment has been suggested. This has previously been advocated for orthopedic joint implant procedures, although a recent meta-analysis has questioned its role.53 Currently, there is no evidence available on the effects of unidirectional HEPA-filtered laminar air flow and total-body exhaust suits in shunt insertion surgery.
77.5 Clinical Presentation
Shunt infection is almost always secondary to a surgical intervention. In a retrospective study of almost 1,000 patients with shunts younger than 21 years, factors associated with shunt infection were shunt surgery within the preceding 90 days (adjusted odds ratio [OR], 2.4; 95% CI, 1.3 to 4.4) and presentation with fever (adjusted OR, 8.4; 95% CI, 4.3 to 16.3).54 Although shunt infection should always be considered when a child presents unwell or with unexplained symptoms, particularly fever soon after shunt surgery, a full history and clinical examination should be performed in all cases, with the examiner remaining alert to alternative diagnoses, such as upper respiratory tract infection, otitis media, and urinary tract infection (particularly in patients with myelomeningocele). Nonspecific symptoms of headache, vomiting, and lethargy will be present in half of children with shunt infection; thus, there is significant overlap with the symptoms of shunt blockage, and indeed shunt blockage may coexist with shunt infection. Additional clinical features, such as pyrexia and meningism, will increase the index of suspicion of shunt sepsis. Problems of surgical wound healing should be specifically looked for, in particular erythema along the shunt track, wound breakdown, purulent discharge, and CSF leakage, because these are strongly associated with shunt infection.49
Less commonly, the presenting symptoms of shunt infection are related to the distal site of drainage. With ventriculoperitoneal shunts, abdominal pain, nausea, and swelling commonly herald a distal shunt infection. This presentation may occur many months after the shunt procedure (which is an exception to the usual temporal association between surgery and infection), and this likely reflects the low pathogenicity of the infecting organisms. Abdominal swelling may be caused either by the formation of loculi of infected CSF within the peritoneum or by the accumulation of infected ascites throughout the peritoneal cavity (▶ Fig. 77.1a).34
Abdominal ultrasound can usually identify the septa or pseudocyst walls that typify compartmentalized collections of infected CSF and distinguish these from the diffuse free peritoneal fluid of infected ascites. The distinction may have implications for the future options for CSF drainage once the infection has been eradicated. In the case of a loculate infection, simply relocating the peritoneal catheter to a new abdominal site will usually suffice34,55 however, in a patient with a more generalized peritonitis, the absorptive capacity of the peritoneum may have been impaired, and drainage to the atrium or pleural cavity may have to be considered.
Fig. 77.1 (a) Abdominal swelling due to accumulation of infected CSF. (b) Protrusion of distal tubing through the mouth due to erosion of shunt tubing into the upper GI tract. (Courtesy of Dr. A. Leland Albright.) (c) Protrusion of distal tubing through the anus due to migration of distal tubing into the lower GI tract. (Courtesy of Dr. A Leland Albright.)
Distal infection of a ventriculopleural shunt may also result in impaired CSF absorption—in this instance resulting in shortness of breath, pleuritic chest pain, and empyema. Acute ventriculoatrial shunt infection will present with pyrexia secondary to bacteremia; however, a more specific syndrome of “shunt nephritis” may occur in the context of more chronic ventriculoatrial shunt infection.56 This is an immune complex–mediated glomerulonephritis most commonly occurring in association with Staphylococcus epidermidis shunt infection; it presents with rash, arthropathy, hematuria, and renal insufficiency and can progress to renal failure if the underlying infection is not adequately treated.
Unusually, the shunt may erode though the skin, bladder, bowel, or oral cavity. In such circumstances, the tubing must be considered contaminated and the patient at high risk for progression to CSF infection, necessitating removal of the shunt and replacement with external ventricular drainage pending the results of CSF analysis (▶ Fig. 77.1b,c).
77.6 Diagnosis of Shunt Infection
There is no universally agreed-upon definition of shunt-related infection. A distinction should be drawn between superficial wound infections, deep incisional infections (that do not penetrate to the CSF or to the distal compartment of drainage), and “organ space” infections; the latter include classic shunt/CSF infections and isolated peritoneal infections. There is little doubt that incisional infections portend a high risk for subsequent shunt infection, although if identified and treated promptly, they may be adequately managed without recourse to removal of the shunt.
A positive CSF culture is the most definitive diagnostic criterion for shunt infection and whenever possible should be sought before treatment is initiated. However, organisms responsible for shunt infection grow in culture at different rates, and it is worth noting that 25% of CSF cultures from hardware take at least 3 days to become positive.57 Culture for at least 10 days has been recommended, and for some organisms extended culture beyond this time will be required. Bacteriologic culture results often need to be interpreted with caution and in conjunction with microbiological advice because culture results are influenced by the method of collection and processing, the availability of repeated sampling, and the prior use of antibiotics. For example, inadequate attention to aseptic technique at the time of sampling can result in false-positives due to contamination with skin commensal bacteria, and false-negatives may result if empiric antibiotic therapy has been commenced before CSF sampling. Additionally, isolation of the infecting agent can prove difficult, particularly in the case of low-grade pathogens such as propionibacteria in an abdominal pseudocyst, which may require prolonged anaerobic culture.
77.6.1 Diagnostic Studies
Blood investigations usually reveal a raised polymorphonuclear leukocyte count,54 and the serum level of C-reactive protein (S-CRP) will commonly be raised in the presence of shunt infection.58 In one study of children with suspected shunt infection, elevated S-CRP showed 97.1% sensitivity and 73.5% specificity for the presence of shunt infection.58 The negative predictive value of S-CRP in this study was 97.3%, so a normal S-CRP level may obviate the need for shunt tap in borderline cases, at least in the initial phase of investigation. S-CRP is also useful as an additional parameter by which to monitor the response to treatment. Blood cultures are usually not positive in ventriculoperitoneal shunt infections but can be useful in the evaluation of suspected ventriculoatrial shunt infection.
Where wound discharge or other signs of wound infection are present, microbiological swabs should be taken. Additional investigations, including urinalysis and possibly chest X-ray, should be considered in the search for alternative causes of fever.
Although not necessarily of diagnostic value per se in the context of shunt infection, computed tomography or magnetic resonance imaging of the brain should be performed because shunt obstruction may coexist with shunt infection, and in the event that the shunt has to be replaced with an external ventricular drain, knowledge of the ventricular configuration is essential; it may have changed significantly since the initial shunt placement. In patients with complex shunt histories and multiple revisions, X-rays of the shunt system will not only provide evidence of continuity of the shunt system but also serve as a means of looking for lost or retained shunt tubing, which will require removal along with the shunt to eradicate shunt infection.
Samples of CSF for culture should be obtained not only to establish the diagnosis but also to ascertain microbiological sensitivities in order that appropriate antibiotic therapy can be selected. The percutaneous aspiration of CSF from the shunt system with strict aseptic technique is the most usual method and has a high diagnostic sensitivity compared with lumbar puncture or ventricular tap.59 The percutaneous aspiration of shunt reservoirs, although generally safe,60 carries some risk for introducing infection and should therefore be performed only when there is reasonable clinical suspicion of shunt infection and after other causes for the clinical presentation have been excluded. In cases of abdominal CSF collections, ultrasound-guided aspiration of CSF can be performed, although in this instance the likelihood of infection is sufficiently high to justify proceeding with externalization of the shunt, which affords the opportunity to aspirate CSF via the distal tubing at the time of surgery.
77.7 Treatment of Shunt Infection
Like with most aspects of this subject, the optimal treatment of shunt infection is controversial, and each of the treatment strategies advocated has its advantages and disadvantages. There are two principal points of contention— firstly, whether intraventricular as well intravenous antibiotics should be used, and secondly, whether or not the shunt should removed.
77.7.1 Antibiotic Therapy
Intravenous and Intraventricular Antibiotic Therapy
Because CoNS are the most common infecting pathogens, and given the high rates of methicillin resistance of these organisms (60%) in hospital practice, a glycopeptide antibiotic (vancomycin or teicoplanin) is generally considered first-line empiric therapy. However, penetration of the blood–brain barrier by this group of drugs is variable, particularly when there is minimal inflammation, so that intravenous and intrathecal administration is advocated. The dosage should be guided by the regular monitoring of levels to ensure an adequate minimum inhibitory concentration (MIC) while avoiding toxicity. If no intraventricular access is available, intravenous vancomycin should be supplemented with an additional central nervous system–penetrating drug, such as rifampicin or linezolid. Newer agents, such as linezolid, have good CSF penetration and are a suitable alternative for gram-positive infections, particularly in refractory cases or cases with resistance to first-line antimicrobials.61,62 Empiric treatment for a gram-negative infection should be given if one is suspected (if the child is severely systemically unwell, if gram-negative organisms have been seen on CSF examination, or if local epidemiology indicates a high rate of gram-negative infection). An appropriate agent would be a third-generation cephalosporin (if local surveillance data do not show a high rate of extended-spectrum β-lactamase–positive organisms) or a carbapenem, with or without an aminoglycoside. Aminoglycosides also have variable penetration of the blood–brain barrier, so intravenous gentamicin may be supplemented with intrathecal gentamicin when there is a susceptible isolate. Clearly, once an isolate has grown, the antibiotic choice may be modified according to sensitivities.
Factors Influencing the Choice of Antibiotic
The following factors need to be accounted for in choosing an appropriate antibiotic regime:
The likely causative organisms. When possible, the CSF should be sampled before antibiotics are started. Broad-spectrum antibiotic cover can then be commenced pending the results of culture and sensitivity tests. The empiric antibiotic policy will need to reflect the commonly seen shunt-infecting organisms in the neurosurgical institution (▶ Table 77.3). In developing countries, the prevalence of gram-negative shunt infection is such that this might need to be reflected in the choice of empiric therapy—for example, the addition of ceftriaxone, another third-generation cephalosporin, or a carbapenem. (Chloramphenicol can be used if other drugs are not available.)
The clinical condition of the child and local signs of sepsis. In an acutely unwell child, broad-spectrum antibiotics may have to be started before CSF sampling to include empiric cover for sepsis appropriate to the age of the child.
Colonization with known antimicrobial-resistant microbial flora (e.g., methicillin-resistant S. aureus [MRSA], extended-spectrum β-lactamase–producing Enterobacteriaceae).
Whether or not the shunt is to be immediately removed.
Whether intraventricular access will be available.
Local antimicrobial availability.
A history of antibiotic allergy.
Local patterns of antibiotic resistance.
Pediatric neurosurgical units should develop an agreed-upon treatment policy in conjunction with their microbiologists and infectious disease experts. An example of the treatment policy at our institution is shown in ▶ Table 77.3.
Drug | Route of administration | Dose | Terapeutic monitoring | Duration of therapy | |
Vancomycin | Intraventricular (intrathecal) | Preterm | 2 mg daily | CSF levels early each morning; dose only to be given if level < 15 mg/L | Minimum 10 day period following the first negative CSF cultures |
Term–3 months | 2.5 mg daily | ||||
3–6 months | 3 mg daily | ||||
> 6 months | 5 mg daily | ||||
Vancomycin | Intravenous | See GOS vancomycin guideline and note 6 hour dosing is NOT routinely used alongside intraventricular (intrathecal) vancomycin | |||
|
77.7.2 Surgical Management
With respect to the surgical management of shunt infection, three treatment options have been debated: (1) antibiotics, shunt removal, and placement of an extraventricular drain (EVD); (2) antibiotics, shunt removal, and immediate shunt replacement; and (3) antibiotics only.
Antibiotics, Shunt Removal, and Temporary External Ventricular Drainage
This strategy is generally accepted as the current standard of treatment.63–65 Only one randomized study has compared the three treatment options, and although the numbers were small, all infections in the removal-plus-EVD group were successfully treated with the shortest hospital stay.66
A literature review (not exclusively pediatric) comparing the different modalities of treatment of shunt infection identified 11 studies (224 patients) in which the strategy of shunt removal plus EVD had been used.67 The total infection cure rate was 87.7% (95% CI, 83.0 to 91.3), and the mortality rate was 5.7% (95% CI, 3.5 to 9.4). This regime entails at least two surgical procedures and potentially additional surgical incisions because some surgeons like to use separate ventricular sites for the EVD and the definitive shunt. The review concluded that this policy of treatment was superior to the other treatments, assuming that the complication rate of EVD was below 35.8%.
The extirpated infected shunt should be sent for microbiological analysis. It is important that all shunt tubing be removed because retained catheters may harbor bacteria and result in treatment failure (▶ Fig. 77.2).
Fig. 77.2 Retained shunt tubing. 3D CT scan showing retained ventricular catheters. Successful treatment of a shunt infection requires the removal of all catheter tubing.
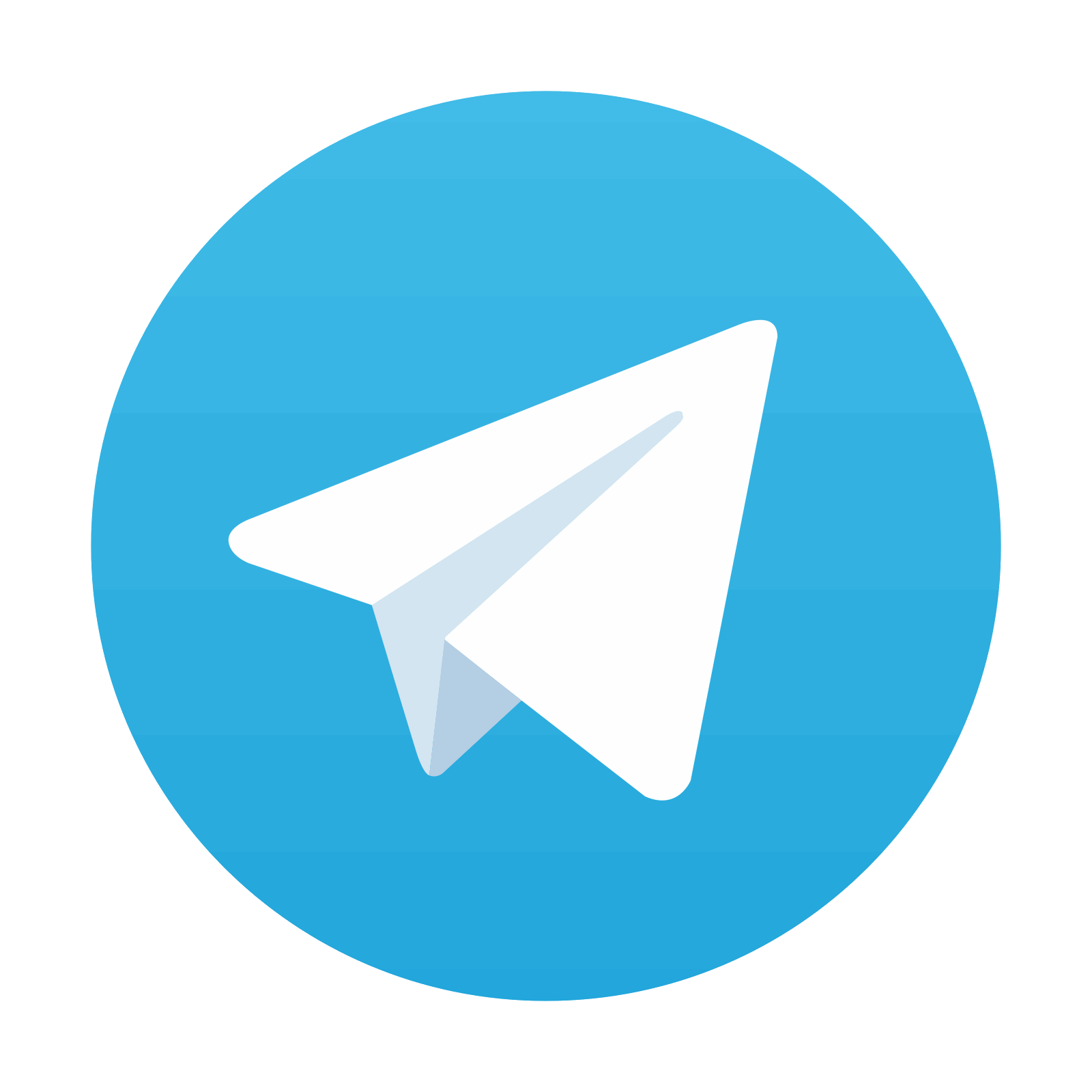