Sleep and Dreaming
Sleep Consists of Alternating REM and Non-REM Periods
Sleep Obeys Circadian and Ultradian Rhythms
The Circadian Rhythm Clock Is Based on a Cyclic Production of Nuclear Transcription Factors
The Ultradian Rhythm of Sleep Is Controlled by the Brain Stem
Sleep-Related Activity in the EEG Is Generated Through Local and Long-Range Circuits
The Characteristics of Sleep Vary Greatly Between Species
Sleep Disorders Have Behavioral, Psychological, and Neurological Causes
Excessive Daytime Sleepiness Is Indicative of Disrupted Sleep
The Disruption of Breathing During Sleep Apnea Results in Fragmentation of Sleep
Narcolepsy Is Characterized by Abnormal Activation of Sleep Mechanisms
Restless Leg Syndrome and Periodic Leg Movements Disrupt Sleep
Parasomnias Include Sleep Walking, Sleep Talking, and Night Terrors
SLEEP IS A REMARKABLE STATE. It consumes fully a third of our lives—approximately 25 years in the average lifetime—yet most of us know little about this daily excursion into our inner world. Perhaps even more surprising, we are still hard pressed to give a raison d’etre for sleep.
The exact functions of sleep and of dreaming, one of the more spectacular components of sleep, have been debated over the ages and are still not known. Do dreams reveal some inner psychological functioning of unconscious mental processes, as Sigmund Freud first suggested, or are they merely the consequence of random firing of neurons in the brain? The psychic content of dreams has been a rich subject of speculation throughout history. Plato, anticipating Sigmund Freud, thought that all of us have a “terrible, fierce, and lawless blood of desires, which it seems are revealed in our sleep,” whereas Aristotle believed that dreams are merely afterthoughts of the day’s activities and experiences.
Although we have only limited understanding of the functions of sleep, our insight into its mechanisms has increased greatly over the past 50 years. We have moved away from the intuitively appealing but incorrect notion that sleep is a period of relative inactivity and rest—one that occurs reflexively in response to reduction of sensory input—to the current view that sleep is a highly organized state generated by the cooperative interplay of many behavioral and neural components. Although in many respects the biological function of sleep remains a mystery, we have begun to understand the cellular and molecular processes underlying sleep.
Sleep Consists of Alternating REM and Non-REM Periods
Sleep affects all of our bodily and mental functions, from the regulation of hormonal levels to muscle tone, from the regulation of respiration rate to the content of our thought processes. Given these important behavioral changes, it is not surprising that the brain’s overall electrical activity changes significantly with sleep. During wakefulness the electroencephalogram (EEG) shows relatively low-voltage, high-frequency, fast activity, reflecting an active cerebral cortex busy with perception and cognition. Slow synchronized oscillatory activity is either at a minimum or occurs only transiently in small groups of neurons (Figure 51-1). Relaxing or closing the eyes may produce alpha waves, especially over the visual cortex, indicating relatively synchronous rhythmic activity in the underlying cortical networks.
To describe sleep quantitatively and to distinguish its stages, sleep researchers routinely use three measures: brain activity measured by the EEG (see Chapter 50), eye movements recorded by the electro-oculogram (EOG), and muscle tone measured by the electromyogram (EMG). These three measures are used because of their reliability, ease of recording, and discriminatory power.
Non-REM Sleep Has Four Stages
Based on these measures, sleep is subdivided into five stages. Stages 1 to 4 comprise non-rapid eye movement (REM) sleep, while stage 5 is REM sleep. Stage 1 sleep is the transition between waking and sleep, the period in which sleep is thought to be imminent but not yet developed. During stage 1 the EEG exhibits a decrease in the high-frequency activity that characterizes the waking state. Stage 2, the first true stage of sleep, is marked in the EEG by the onset of spindle waves, 7-15 Hz oscillations over a period of 1-2 seconds that resemble a spindle of thread, and K-complexes (Figure 51-1). Spindle waves and K-complexes reflect slow, synchronized oscillations of neuronal and synaptic activity within the thalamus and cerebral cortex. This state is the result of the relaxation and general hyperpolarization of neurons and neuronal networks that follow gradual inactivation of the brain mechanisms of arousal. During stage 2 sleep muscle tone decreases, and the eyes slowly roll back and forth. Respiration becomes more regular and slows (Figure 51-2), and body temperature begins to fall.
Stage 3 sleep is heralded in the EEG by the appearance of a significant fraction of delta wave oscillations (0.5-4 Hz). These signal a further reduction in arousal processes in the brain and increased synchronization of cortical and thalamic activity. A predominance of delta waves (> 50% of the time in the EEG) indicates that the person is in stage 4 sleep, the deepest stage. During stages 3 and 4 respiration continues to be slow and regular, heart rate slows, muscles relax, and temperature slowly drifts downward (Figure 51-2).
Figure 51-2 Physiological changes during sleep. Sleep consists of several cycles between stages 1 and 4 and rapid eye movement (REM) sleep. As sleep deepens, eye movements become less prominent; movements of the head, heart rate, and respiration all decrease. As the level of sleep lightens, these trends reverse. During REM sleep heart rate and respiration increase, as does penile erection. Neck movements can occur just before and after an episode of REM sleep, whereas during REM sleep muscle tone is very low. (EOG, electro-oculogram; EMG, electromyogram.) (Adapted, with permission, from Purves et al. 2004.)
The progression from waking to stage 4 sleep at the beginning of the night typically occurs relatively quickly—over the first 30 minutes (Figure 51-1). After approximately 30 minutes in stage 4 sleep the sleeper ascends quickly through all four stages of sleep. Instead of waking, however, the sleeper now enters a unique stage known as rapid eye movement or REM sleep, a period in which dreams can become vivid, even bizarre.
Rapid eye movement (REM) sleep was discovered in 1953 when Eugene Aserinsky and Nathaniel Kleitman first recorded the EEG and EOG of sleeping adults and observed that during sleep these tests showed changes in activity approximately four to five times per night (changing to a state of higher frequency and lower amplitude similar to that of the waking state). During this “activated” period the eyes dart back and forth, thus giving this stage its name. Sleepers roused from REM sleep report experiencing vivid dreams approximately 80% to 95% of the time.
Interestingly, REM sleep is associated with an almost complete loss of muscle tone, owing to inhibition of the spinal motor neurons by descending pathways. Apparently the motor neurons in the brain stem that control eye movements are not inhibited, because the eyes move during REM sleep. During REM sleep temperature regulation is at a low point, and body temperature begins to fall further still.
Although sleep can be segregated into five stages, the REM stage is so distinctive that sleep is often separated into two phases, referred to simply as REM and non-REM (or NREM) sleep. The cycle of sleep—the non-REM sequence from stage 1 to stage 4 and the reverse sequence followed by a brief period of REM sleep—occurs repeatedly during the night, in a pattern that is best described as a damped oscillation. As the night progresses, the depth of non-REM sleep decreases and the duration spent in REM sleep increases (Figure 51-1). As a result of this pattern, and the fact that it is necessary to wake up to remember your dreams, the dreams that take place in the morning are the ones most often remembered.
Figure 51-1 The electrical activity of the brain is distinctive during wakefulness and each of the five stages of sleep.
A. Electrodes are placed systematically on the scalp for recording the electroencephalogram (see Figure 50-1). Each trace of the electroencephalogram (EEG) is actually the difference between neighboring electrodes, a technique that removes noise. When the subject is awake with eyes open, the EEG exhibits low-voltage, higher-frequency activities. Stage 1 sleep is characterized by a slight slowing of frequencies, whereas stages 2 and 3 sleep are characterized by spindle waves and K-complexes as well as increases in other slow rhythms. During stage 4 sleep slow rhythms become very prominent. During rapid eye movement (REM) sleep is similar to that during waking.
B. Over the course of the night several cycles of deepening and lightening of sleep occur as a damped oscillation, known as the ultradian rhythm. Upon falling asleep, non-REM sleep deepens, followed by REM sleep, and then a period of lightened non REM sleep. Each cycle is approximately 90 minutes. (Adapted, with permission, from Purves et al. 1997 and 2004.)
REM and Non-REM Dreams Are Different
In adults REM sleep occupies approximately 25% of the total sleep time. Most people would be surprised to find that they had vivid dreams for approximately 2 hours every night. Indeed, the inability to remember dreams (unless awakened) is one of the great mysteries of sleep.
Analysis of dreams and their content have revealed some surprising results. Most people believe that dreams are only occasional and fleeting occurrences of highly bizarre or emotional content, in which the entire scenario is dreamt in an instant. In reality, specific dreams recur with a regular and predictable periodicity, and mostly reflect everyday events. Even the fleeting nature of dreams is a misperception. Events in dreams occur over a period of time that is about as long as they would in real time. Only a small percentage of dreams contain bizarre and fantastic elements.
Dreams occur in both REM and non-REM sleep but the characteristics of REM and non-REM dreams differ. REM dreams are relatively long, primarily visual, somewhat emotional, and usually not connected to the immediate events of the everyday life of the dreamer. These resemble what Freud referred to as the latent content of the dream. Non-REM dreams are shorter, less visual, less emotional, more conceptual, and usually related to the current life of the dreamer. They resemble what Freud referred to as the day’s residues, or the manifest content of the dream. This conceptual, “thought-like” mentation can occupy up to 50% of non-REM sleep.
What sensory modalities are experienced during dreams in REM sleep? Vision is preeminent, occurring in all dreams (except, of course, in the congenitally blind), whereas auditory events occur in approximately 65%, vestibular 8%, and temperature 4%; tactile, olfactory, and gustatory experiences are rare (only 1% each). The emotional content of dreams varies from anxiety (14%), to surprise (9%), joy (7%), sadness (5%), and shame (2%). Men experience penile erections, and women experience the physiological counterparts of sexual arousal during REM sleep. The regularity of penile erection during REM sleep (Figure 51-2) can help determine if impotence in a patient has a psychological or physiological origin. The emotional content is organized at several levels of the central nervous system but is, for the most part, unrelated to the specific content of the dream.
Sleep Obeys Circadian and Ultradian Rhythms
Sleep is a circadian behavior that is composed of cyclical or ultradian stages. In all species the period of sleep is dictated by the rotation of the earth and time clues (German, zeitgebers) that indicate the day-night cycle. The principal zeitgeber is the daily rising of the sun. It was once thought that the circadian rhythms of plants and animals are strongly or completely driven by the rising and setting of the sun. However, in 1729 the Swiss astronomer Jean-Jacques d’Ortous de Mairan observed that even in the dark the leaves of plants move according to a diurnal cycle. Since then endogenous rhythms of about 24 hours (ie, circadian rhythms) have been found to be prevalent throughout the plant and animal kingdoms. Circadian rhythms seem to have evolved so as to maximize the use of the day-night cycle by anticipating the rising and setting of the sun, even without clear signals from sunlight or other zeitgebers.
The features of the circadian rhythm of sleep in human beings, in the absence of zeitgebers, has been documented by a few brave individuals willing to live in isolation in specially constructed rooms in labs or caves. These rooms contain no clues as to the time of day in the outside world—no clocks, windows, radios, televisions, e-mail, Internet, or sounds that would tell
subjects the time in the outside world. Under these conditions subjects were allowed to sleep and be active whenever their body dictated. Interestingly, the circadian rhythm persists, but its duration varies among subjects and is typically slightly longer (eg, 25 hours) than that of the normal day-night cycle, causing each person in isolation to slowly drift out of phase with the outside environment. Reexposure to the external day-night cycle rapidly resets the sleep-wake cycles (Figure 51-3A).
Figure 51-3 The endogenous circadian rhythm of sleep in humans.
A. A human volunteer isolated in an underground bunker is initially exposed to a normal day-night cycle and exhibits a circadian period of wakefulness (white bar) that is synchronized with the day-night cycle. However, following removal of external cues (after day 3) the volunteer’s circadian cycle lengthens from 24 hours to approximately 26 hours (days 4-21). Because the endogenous cycle of the volunteer is longer than that of the normal day-night cycle, the period of wakefulness slowly drifts out of phase. Following reintroduction of environmental cues to the normal day-night cycle, the subject’s periods of wakefulness once again become synchronized to the day-night cycle. (Adapted, with permission, from Aschoff 1965.)
B. The suprachiasmatic nucleus in the hypothalamus is the master circadian clock of the nervous system. (Adapted, with permission, from Purves et al. 1997.)
The sleep-wake cycle is not the only circadian rhythm in the body. Many other physiological processes also exhibit daily maximums and minimums. The level of arousal, the ability to do cognitive tasks such as math problems, body temperature, hormone release, and kidney function all follow endogenous 24-hour rhythms that normally are synchronized to the day-night cycle.
How do sleep and other circadian rhythms become synchronized to the day-night cycle? A small number of retinal ganglion cells (see Chapter 26) respond directly to light and project to the suprachiasmatic nucleus in the hypothalamus (Figure 51-3B). It is this pathway, described in detail later, that synchronizes the circadian rhythmicity with the day-night cycle. Although rare, some people with tumors or lesions of the hypothalamus lose the circadian rhythmicity of their sleep-wake cycle and therefore sleep for short periods throughout the day. Other people, equally rare, have a normal circadian rhythm in their sleep-wake cycle but are unable to synchronize it with the day-night cycle of their environment. The phase relationship between their own endogenous need to sleep, and the time of day, changes continually. Perhaps in these people the neural path conveying light information to the suprachiasmatic nucleus is defective.
Jet lag demonstrates other properties of our circadian rhythm. Travel to a distant time zone disrupts the synchrony of one’s circadian rhythm with the day-night cycle. One may temporarily experience excessive sleepiness during the day, hunger at the wrong times, cold despite being in a warm environment, and awakenings in the middle of the night despite sleep deprivation. This feeling of being “out of phase” is common and has a physiological basis. Flying west is generally easier than flying east, presumably because our endogenous rhythm is approximately 25 hours. When flying west we take advantage of this long rhythm by staying up late and sleeping in. Indeed, many individuals practice this on a weekly basis—staying up late and sleeping in on the weekend. The jarring experience of the “Monday morning blues” results from a rapid shift in the circadian cycle and perhaps a bit of sleep deprivation thrown in as well.
Sleepiness—the drive to sleep—depends on several factors. Two of the strongest are the time since the last full period of sleep and the circadian rhythm. We therefore can think of the drive to sleep as two separate processes: a “sleep deficit” that slowly builds up without sleep and dissipates with sleep, and a circadian rhythmicity in arousal. The difference between these processes reflects the sleep drive. After a full night of sleep the sleep deficit should be very low. In the morning our level of arousal slowly increases as does our sleep deficit. Because sleep deficit and alertness increase together, the difference between these two processes—the drive to sleep—is small and therefore weak. Toward evening, however, our sleep deficit continues to increase, and the circadian rhythm of arousal begins to wane, resulting in a larger and larger sleep drive (Figure 51-4A). Sleeping a full night once again removes the sleep debt, and the process starts over.
Figure 51-4 Hypothetical model of the circadian rhythmicity of the sleep drive. The difference between two interacting circadian processes—alertness and sleep deficit—determines sleep drive. (Adapted, with permission, from Daan et al. 1984.)
A. During a normal sleep-wake cycle the drive to sleep (yellow) accumulates during the day and declines during sleep (blue) such that the desire to sleep is greatest at bedtime and least on wakening.
B. Missing a night of sleep results in a continual build up of the sleep deficit (curve S) while the endogenous circadian rhythm of arousal (curve C) persists. As a result, over the period of wakefulness the sleep drive (the difference between S and C curves) varies. It is greatest in the middle of the night of missed sleep as well as at normal bedtime on the second evening.
If a night of sleep is missed, one is likely to find that at approximately 3-4 a.m. it is nearly impossible to stay awake. This is because the sleep deficit is high and continues to increase, while the circadian rhythm in arousal (and other body functions such as temperature) is at a low point. Thus the sleep drive is high (Figure 51-4B). Sleep once again restores the balance, relieving this built-up sleep pressure.
The Circadian Rhythm Clock Is Based on a Cyclic Production of Nuclear Transcription Factors
The search for the neural basis of circadian rhythmicity of sleep has led to one site, the suprachiasmatic nucleus (Figure 51-3B), so named because of its location just above the optic chiasm, the place where the optic nerves cross underneath the hypothalamus.
The firing frequency of neurons in the suprachiasmatic nucleus follows an endogenous circadian rhythm (see Figure 51-6C). Thus the 20,000 neurons in the suprachiasmatic nucleus make up the master clock for circadian rhythms. They are the critical pacemaker for organizing sleep into a circadian pattern. The circadian activity of these neurons is entrained by environmental stimuli such as light, as measured by release of the transmitter vasopressin (see Figure 51-6C). Lesioning this nucleus in animals causes complete loss of the circadian rhythmicity of sleep-wake cycles. Animals with these lesions continue to have a normal daily amount of sleep, but sleep occurs randomly throughout the day and night. Transplantation of the suprachiasmatic nucleus from another animal can restore a circadian sleep rhythm, with the host animal adopting the sleep-wake cycle of the donor.
The suprachiasmatic nucleus is organized into distinct functional groups and acts as the controller for the rhythmic oscillations of clocks located in other organs in the body. These “peripheral” clocks are capable of maintaining their own circadian rhythms for only a few days without input from the suprachiasmatic nucleus.
Like a grandfather clock, the biological clocks that control circadian rhythms have many parts: A complex set of transcription factors, proteins, kinases, phosphatases, and regulatory molecules that have been remarkably conserved throughout the evolution of species. A number of molecules that play key roles in the clock mechanism have been identified over the last 20 years, largely from experiments in the fruit fly Drosophila and the mouse. The essence of the clock mechanism in the suprachiasmatic nucleus, as well as in other organs, is a pair of transcriptional feedback loops; one forms the core circadian mechanism whereas a second forms a modulatory loop that stabilizes the circadian rhythm. Such interlocking feedback loops are quite similar in flies and mammals (see Chapter 3).
At the center of the two loops are two transcriptional activators, CLOCK and BMAL1. These transcription factors bind to each other and form a heterodimer that enhances transcription of the mouse gene per (mPer1-3) and cryptochrome genes (mCry1-2), thereby increasing the cytoplasmic concentrations of the PER and CRY proteins. PER and CRY form heterodimers, reenter the nucleus, and inhibit CLOCK and BMAL1, thus repressing PER and CRY transcription. This sequence creates the core circadian mechanism in which Bmal1 RNA reaches a peak 12 hours out of phase with mPer and mCry RNA. CLOCK and BMAL1 are also at the center of the modulatory loop that modifies the levels of CLOCK/BMAL1 heterodimers. Mutations in genes in the stabilizing loop do not disrupt circadian rhythms to the same degree as those in the core loop.
Once the central clock in the suprachiasmatic nucleus generates the rhythm and the environmental light-dark cycle synchronizes it, this information must be communicated to various systems through humoral or electrical signals. To achieve this, the rhythmic molecular signal is transduced into electrical activity in suprachiasmatic nucleus neurons and then transmitted to other brain regions through action potentials.
A number of areas in the hypothalamus receive input from the suprachiasmatic nucleus and play a role in integrating the output of the suprachiasmatic nucleus. For example, a large number of axons from the suprachiasmatic nucleus terminate in the dorsal and ventral subparaventricular zones of the hypothalamus. The dorsal subparaventricular zone appears to project to areas that are important for circadian rhythms of body temperature, whereas the ventral subparaventricular zone and the downstream dorsomedial nucleus of the hypothalamus are important in the sleep-wake cycle. The dorsomedial nucleus may serve as a common final pathway for a number of circadian rhythms as lesions of this nucleus interrupt circadian rhythms for feeding, locomotion, and corticosteroid secretion as well as the sleep-wake cycle.
The Ultradian Rhythm of Sleep Is Controlled by the Brain Stem
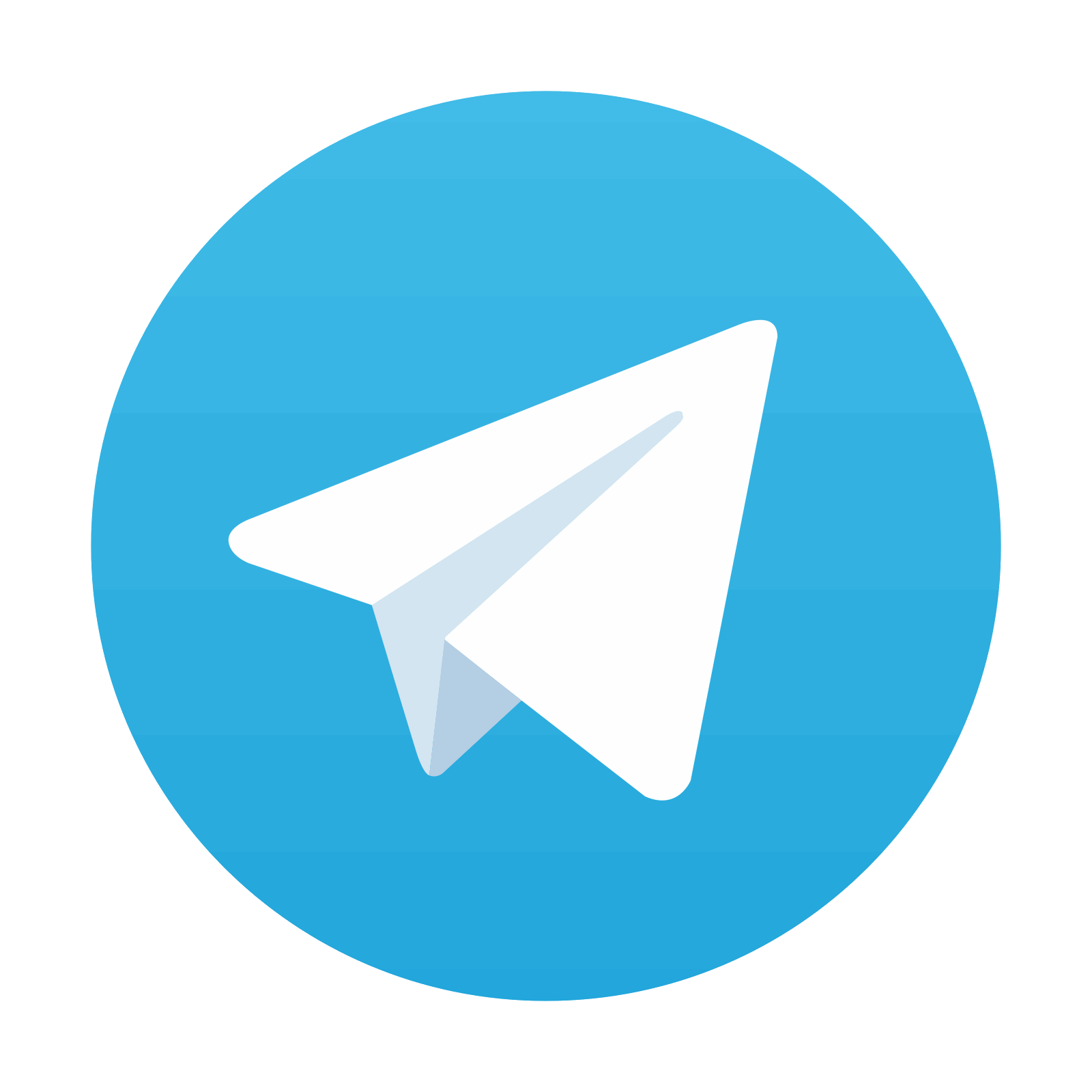
Stay updated, free articles. Join our Telegram channel

Full access? Get Clinical Tree
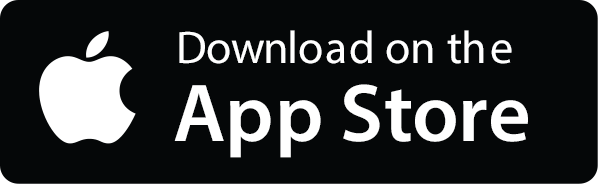
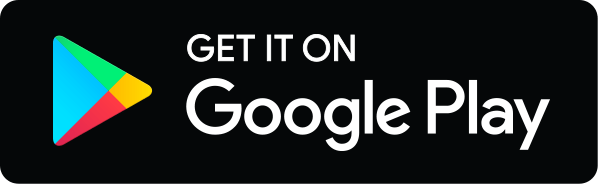