Smell and Taste: The Chemical Senses
A Large Number of Olfactory Receptor Proteins Initiate the Sense of Smell
Different Combinations of Receptors Encode Different Odorants
Olfactory Information Is Transformed Along the Pathway to the Brain
Sensory Inputs in the Olfactory Bulb Are Arranged by Receptor Type
The Olfactory Bulb Transmits Information to the Olfactory Cortex
Output from the Olfactory Cortex Reaches Higher Cortical and Limbic Areas
Odors Elicit Characteristic Innate Behaviors
Invertebrate Olfactory Systems Can Be Used to Study Odor Coding and Behavior
The Anatomy of the Insect Olfactory System Resembles That of Vertebrates
Olfactory Cues Elicit Stereotyped Behaviors and Physiological Responses in the Nematode
The Gustatory System Controls the Sense of Taste
Sensory Neurons Carry Taste Information from the Taste Buds to the Brain
Taste Information Is Transmitted from the Thalamus to the Gustatory Cortex
Perception of Flavor Depends on Gustatory, Olfactory, and Somatosensory Inputs
THROUGH THE SENSES OF SMELL and taste we are able to perceive a staggering number and variety of chemicals in the external world. These chemical senses inform us about the availability of foods and their potential pleasure or danger. Smell and taste also initiate physiological changes required for the digestion and utilization of food. In many animals the olfactory system also serves an important social function by detecting pheromones that elicit innate behavioral or physiological responses.
Although the discriminatory ability of humans is somewhat limited compared with that of many other animals, odor chemists estimate that the human olfactory system may be capable of detecting more than 10,000 different volatile chemicals. Perfumers who are highly trained to discriminate odorants can distinguish as many as 5,000 different types of odorants, and wine tasters can discern more than 100 different components of taste based on combinations of flavor and aroma.
In this chapter we consider how odor and taste stimuli are detected and how they are encoded in patterns of neural signals transmitted to the brain. In recent years much has been learned about the mechanisms underlying chemosensation in a variety of animal species. Certain features of chemosensation have been conserved through evolution, whereas others are specialized adaptations of individual species.
A Large Number of Olfactory Receptor Proteins Initiate the Sense of Smell
Odorants—volatile chemicals that are perceived as odors—are detected by olfactory sensory neurons in the nose. The sensory neurons are embedded in a specialized olfactory epithelium that lines part of nasal cavity, approximately 5 cm2 in area in humans (Figure 32–1), and are interspersed with glia-like supporting cells (Figure 32–2). They are relatively short-lived, with a life span of only 30 to 60 days, and are continuously replaced from a layer of basal stem cells in the epithelium.
Figure 32-1 The olfactory system. Odorants are detected by olfactory sensory neurons in the olfactory epithelium, which lines part of the nasal cavity. The axons of these neurons project to the olfactory bulb where they terminate on mitral and tufted cell relay neurons within glomeruli. The relay neuron axons project to the olfactory cortex where they terminate on the dendrites of pyramidal neurons whose axons project to other brain areas.
Figure 32-2 The olfactory epithelium.
A. The olfactory epithelium contains sensory neurons interspersed with supporting cells as well as a basal layer of stem cells. Cilia extend from the dendrite of each neuron into the mucus lining the nasal cavity. An axon extends from the basal end of each neuron to the olfactory bulb.
B. A scanning electron micrograph of the olfactory epithelium shows the dense mat of sensory cilia at the epithelial surface. Supporting cells (S) are columnar cells that extend the ful depth of the epithelium and have apical microvilli. Interspersed among the supporting cells are an olfactory sensory neuron (O) with its dendrite and cilia and a basal stem cell (B). (Reproduced, with permission, from Morrison and Costanzo 1990.)
The olfactory sensory neuron is a bipolar nerve cell. A single dendrite extends from the apical end to the epithelial surface, where it gives rise to numerous thin cilia that protrude into the mucus that coats the nasal cavity (Figure 32–2). The cilia have receptors that recognize odorants as well as the transduction machinery needed to amplify the sensory signals and transform them into electrical signals in the neuron’s axon, which projects from the basal pole of the neuron to the brain. The axons of olfactory sensory neurons pass through the cribriform plate, a perforated region in the skull above the nasal cavity. The axons then synapse in the olfactory bulb, the first relay in the olfactory pathway (see Figure 32–1).
Mammals Share a Large Family of Odorant Receptors
Odorant receptors are proteins encoded by a multigene family that is evolutionarily conserved and found in all vertebrate species. Humans have approximately 350 different odorant receptors, whereas mice have approximately 1,000. Although odorant receptors belong to the G protein-coupled receptor superfamily, they share sequence motifs not seen in other superfamily members. Significantly, the odorant receptors vary considerably in amino acid sequence (Figure 32–3A).
Figure 32-3 Odorant receptors.
A. Odorant receptors have the seven transmembrane domains characteristic of G protein-coupled receptors. They are related to one another but vary in amino acid sequence (positions of highest variability are shown here as black balls). Humans have approximately 350 different odorant receptors, and mice have approximately 1,000. (Reproduced, with permission, from Buck and Axel 1991.)
B. Binding of an odorant causes the odorant receptor to interact with Gαolf, the α-subunit of a heterotrimeric G protein. This causes the release of a GTP-coupled Gαolf, which stimulates adenylyl cyclase III, leading to an increase in cAMP. The elevated cAMP in turn induces the opening of cyclic nucleotide-gated cation channels, causing cation influx and a change in membrane potential in the ciliary membrane. (cAMP, cyclic adenosine monophosphate; GTP, guanosine triphosphate.)
Like other G protein-coupled receptors, odorant receptors have seven hydrophobic regions that are likely to serve as transmembrane domains (Figure 32–3A). Detailed studies of other G protein-coupled receptors, such as the β-adrenergic receptor, suggest that odorant binding occurs in a pocket in the transmembrane region formed by a combination of the transmembrane domains. The amino acid sequences of odorant receptors are especially variable in several transmembrane domains, providing a possible basis for variability in the odorant binding pocket that could account for the ability of different receptors to recognize structurally diverse ligands.
A second, smaller family of chemosensory receptors is also expressed in the olfactory epithelium. These receptors, called trace amine-associated receptors (TAARs), are G protein-coupled, but their protein sequence is unrelated to that of odorant receptors. They are encoded by a small family of genes present in humans and mice as well as fish. Studies in mice, which have 14 different olfactory TAARs, indicate that TAARs recognize volatile amines, some of which are enriched in the urine of males compared to that of females. It is possible that this small receptor family has a function distinct from that of the other odorant receptors, perhaps one associated with the detection of social cues.
The binding of an odorant to its receptor induces a cascade of intracellular signaling events that depolarize the olfactory sensory neuron (Figure 32–3B). The depolarization spreads passively to the cell body of the olfactory sensory neuron, causing action potentials that are actively conducted in the axon to the olfactory bulb.
Humans and other animals rapidly accommodate to odors, as witnessed by the weakening of an unpleasant odor when it is continuously present. The ability to sense an odorant rapidly recovers when the odorant is temporarily removed. The adaptation to odorants is caused in part by modulation of the cyclic nucleotide-gated ion channel, but the mechanism by which sensitivity is speedily restored is not yet understood.
Different Combinations of Receptors Encode Different Odorants
To be distinguished perceptually, different odorants must cause different signals to be transmitted from the nose to the brain. This is accomplished in two ways. First, each olfactory sensory neuron expresses only one odorant receptor gene and therefore one type of receptor. Second, each receptor recognizes multiple odorants, and conversely each odorant is detected by different types of receptors. Importantly, however, each odorant is detected by a unique constellation of receptors and thus causes a distinctive pattern of signals to be transmitted to the brain.
The combinatorial coding of odorants greatly expands the discriminatory power of the olfactory system. If each odorant were detected by only three different receptors, this strategy could in theory generate millions of different receptor combinations—and an equivalently vast number of different signaling patterns. Interestingly, even odorants with nearly identical structures are recognized by different combinations of receptors (Figure 32–4). The fact that highly related odorants have different receptor codes explains why a slight change in the structure of an odorant can alter its perceived odor. In some cases the result is dramatic, for example changing the perception of a chemical from rose to sour.
Figure 32-4 Each odorant is recognized by a unique combination of receptors. A single odorant receptor can recognize multiple odorants, and one odorant is recognized by a specific combination of different receptors. That is, different odorants are detected by different combinations of receptors. This combinatorial coding of specific odorants explains how mammals can distinguish odorants with similar chemical structures. The data in the figure were obtained by testing mouse olfactory sensory neurons with different odorants and then determining the odorant receptor gene expressed by each responsive neuron. The perceived qualities of these odorants in humans are shown on the right. (Adapted, with permission, from Malnic et al. 1999.)
A change in concentration of an odorant can also change the perceived odor. For example, a low concentration of thio terpineol smells like tropical fruit, a higher concentration smells like grapefruit, and an even higher concentration smells putrid. As the concentration of an odorant is increased, additional receptors with lower affinity for the odorant are recruited into the response and change the receptor code, providing an explanation for the effects of odorant concentration on perception.
Olfactory Information Is Transformed Along the Pathway to the Brain
Odorants Are Encoded in the Nose by Dispersed Neurons
How is a large array of different odorant receptors organized to generate diverse odor perceptions? This question has been investigated in the mouse. Studies in mice have revealed that olfactory information undergoes a series of spatial transformations as it travels from the olfactory epithelium the olfactory epithelium to the olfactory bulb and then to the olfactory cortex.
Different types of odorant receptors are expressed in several coarse zones of the olfactory epithelium of the mouse (Figure 32–5). Each receptor type is expressed in approximately 5,000 neurons that are confined to one zone. (Recall that each neuron expresses only one odorant receptor gene.) Neurons with the same receptor are randomly scattered within the zone so that neurons with different receptors are interspersed. Although one zone may have more receptors for a particular odorant compared to other zones, all zones contain a variety of receptors, so that a specific odorant may be recognized by receptors in several different zones. The evolutionary significance of the zones is unclear, but, as we shall see, the fact that neurons within different epithelial zones project axons to distinct parts of the olfactory bulb suggests that the arrangement of receptors into discrete zones contributes to the establishment of precise information pathways.
Figure 32-5 Organization of sensory inputs in the olfactory epithelium. Sensory neurons in the olfactory epithelium are distributed in discrete areas known as zones, and each odorant receptor gene is expressed by a small subset of neurons within a single zone. Neurons labeled by four different receptor probes are shown here in different zones in sections through the mouse nose. An olfactory marker protein (OMP) probe labels all neurons expressing odorant receptors. (Adapted, with permission, from Ressler, Sullivan, and Buck 1993; adapted, with permission, from Sullivan et al. 1996.)
Because each odorant is detected by an ensemble of neurons that is widely dispersed across the epithelial sheet, some receptors detecting a particular odorant will remain functional when part of the epithelium is damaged by respiratory infection.
Sensory Inputs in the Olfactory Bulb Are Arranged by Receptor Type
The axons of olfactory sensory neurons project to the ipsilateral olfactory bulb, whose rostral end lies just above the olfactory epithelium. The sensory axons terminate on the dendrites of olfactory bulb neurons within bundles of neuropil called glomeruli that are arrayed over the bulb’s surface (Figure 32–6). In each glomerulus the sensory axons make synaptic connections with three types of neurons: mitral and tufted relay neurons, which project axons to the olfactory cortex, and periglomerular interneurons, which encircle the glomerulus.
Figure 32-6 Olfactory bulb interneurons. Within the glomerulus, the dendrites of GABAergic periglomerular cells receive excitatory input from olfactory sensory neurons and have reciprocal synapses with the primary dendrites of mitral and tufted relay neurons, suggesting a possible role in signal modification. The dendrites of GABAergic granule cells deeper in the bulb have reciprocal excitatory-inhibitory synapses with the secondary dendrites of the relay neurons and are thought to provide negative feedback to relay neurons that shapes the odor response. (Adapted, with permission, from Shepherd and Greer 1998.)
The axon of an olfactory sensory neuron terminates in only one glomerulus. Similarly, the primary dendrite of each mitral and tufted relay neuron is confined to a single glomerulus. In each glomerulus the axons of several thousand sensory neurons converge on the dendrites of approximately 40 to 50 relay neurons. This convergence results in approximately a 100-fold decrease in the number of neurons transmitting olfactory signals.
The organization of sensory information in the olfactory bulb is dramatically different from that of the epithelium. Whereas olfactory sensory neurons with the same odorant receptor are randomly scattered in one epithelial zone, their axons converge in a few glomeruli at two spots, one on either side of the olfactory bulb (Figure 32–7). Each glomerulus, and each mitral and tufted relay neuron connected to it, receives input from just one type of odorant receptor. The result is a precise arrangement of inputs from different odorant receptors, which is similar between individuals.
Figure 32-7 Odor responses in the olfactory bulb.
A. The axons from neurons in one epithelial zone with the same odorant receptor type usually converge to two glomeruli, one on each side of the olfactory bulb. Here a probe specific for one odorant receptor gene labeled a glomerulus on the medial side (left) and lateral side (right) of a mouse olfactory bulb. The probe hybridized to receptor messenger RNAs present in sensory axons in these coronal sections. (Adapted from Ressler, Sullivan, and Buck 1994.)
B. This section of a rat olfactory bulb shows the uptake of radiolabeled 2-deoxglucose at multiple foci (red) following exposure of the animal to the odorant methyl benzoate. The labeled foci correspond to numerous glomeruli at different locations in the olfactory bulb. (Reproduced, with permission, from Johnson, Farahbod, and Leon 2005.)
C. The olfactory bulb has a precise map of odorant receptor inputs because each glomerulus is dedicated to only one type of receptor. The maps in the two olfactory bulbs are bilaterally symmetrical and are nearly identical across individuals. The maps on the medial and lateral sides of each bulb are similar, but slightly displaced along the dorsal-ventral and anterior-posterior axes.
Because each odorant is recognized by a unique combination of receptor types, each odorant also activates a particular combination of glomeruli in the olfactory bulb (Figure 32–7B). At the same time, just as an odorant receptor recognizes multiple odorants, a single glomerulus—or a given mitral or tufted cell—is activated by more than one odorant. Closely related odorants can stimulate glomeruli in the same subregion of the bulb, suggesting that the organization of inputs is related to odorant structure. Owing to the nearly stereotyped pattern of receptor inputs in the olfactory bulb, the patterns of glomerular activation elicited by individual odorants are similar in all individuals and are bilaterally symmetrical in the two adjacent bulbs.
This organization of sensory information in the olfactory bulb is likely to be advantageous in two respects. First, the fact that signals from thousands of sensory neurons with the same odorant receptor type always converge on the same few glomeruli and relay neurons in the olfactory bulb may optimize the detection of odorants present at low concentrations. Second, although olfactory sensory neurons with the same receptor type are dispersed and are continually replaced, the arrangement of inputs in the olfactory bulb remains unaltered. As a result, the neural code for an odorant in the brain is maintained over time, assuring that an odorant encountered previously can be recognized years later.
One mystery that remains unsolved is how all the axons of olfactory sensory neurons with the same type of receptor are directed to the same glomeruli. Studies using transgenic mice indicate that the odorant receptor itself somehow determines the target of the axon, but how it does so is unclear.
Sensory information is processed and possibly refined in the olfactory bulb before it is forwarded to the olfactory cortex. Each glomerulus is encircled by periglomerular interneurons that receive excitatory input from sensory axons and form inhibitory dendrodendritic synapses with mitral and tufted cell dendrites in that glomerulus and perhaps adjacent glomeruli. The periglomerular interneurons may therefore have a role in signal modulation. In addition, granule cell interneurons deep in the bulb provide negative feedback onto mitral and tufted cells. The granule cell interneurons are excited by the basal dendrites of mitral and tufted cells and in turn inhibit those postsynaptic relay neurons and others with which they are connected. The lateral inhibition afforded by these connections is thought to dampen signals from glomeruli and relay neurons that respond to an odorant only weakly, thereby sharpening the contrast between important and irrelevant sensory information before its transmission to the cortex.
Other potential sources of signal refinement are the retrograde projections to the olfactory bulb from the olfactory cortex, basal forebrain (horizontal limb of the diagonal band), and midbrain (locus ceruleus and raphe nuclei). These connections may modulate olfactory bulb output according to the physiological state of an animal. When the animal is hungry, for example, some centrifugal projections might heighten the perception of the aroma of foods.
The Olfactory Bulb Transmits Information to the Olfactory Cortex
The axons of the mitral and tufted relay neurons of the olfactory bulb project through the lateral olfactory tract to the olfactory cortex (Figure 32–8 and see Figure 32–1). The olfactory cortex, defined roughly as that portion of the cortex that receives a direct projection from the olfactory bulb, comprises five main areas: (1) the anterior olfactory nucleus, which connects the two olfactory bulbs through a portion of the anterior commissure; (2) the anterior and posterior cortical nuclei of the amygdala; (3) the olfactory tubercle; (4) part of the entorhinal cortex; and (5) the piriform cortex, the largest and considered the major olfactory cortical area.
Figure 32-8 The olfactory cortex. The axons of mitral and tufted relay neurons of the olfactory bulb project through the lateral olfactory tract to the olfactory cortex. The olfactory cortex consists of a number of distinct areas, the largest of which is the piriform cortex. From these areas olfactory information is transmitted directly to other brain areas as well as indirectly via the thalamus. Targets include frontal and orbitofrontal areas of the neocortex, which are thought to be important for odor discrimination, and the amygdala and hypothalamus, which may be involved in emotional and physiological responses to odors. Mitral cells in the accessory olfactory bulb project to specific areas of the amygdala that transmit signals to the hypothalamus.
In the piriform cortex the axons of olfactory bulb mitral and tufted cells leave the lateral olfactory tract to form excitatory glutamatergic synapses with pyramidal neurons, the projection neurons of the cortex. Signal transmission by the pyramidal neurons appears to be modulated by inhibitory inputs from local GABA-ergic interneurons as well as by excitatory inputs from neighboring pyramidal neurons and the piriform cortex of the other hemisphere. The piriform cortex also receives centrifugal inputs from modulatory brain areas, suggesting that its activity may be adjusted according to behavioral state. Finally, the olfactory cortex projects to the olfactory bulb, providing yet another possible means of signal modulation.
As with the olfactory bulb relay neurons, individual pyramidal neurons can be activated by more than one odorant. However, the pyramidal neurons activated by a particular odorant are scattered across the piriform cortex, an arrangement different from that of the olfactory bulb. Mitral cells in different parts of the olfactory bulb can project axons to the same subregion of the piriform cortex, further indicating that the highly organized map of odorant receptor inputs in the olfactory bulb is not recapitulated in the cortex.
Output from the Olfactory Cortex Reaches Higher Cortical and Limbic Areas
Pyramidal neurons in the olfactory cortex transmit information indirectly to the orbitofrontal cortex through the thalamus and directly to the frontal cortex. These pathways to higher cortical areas are thought to be important in odor discrimination. In fact, people with lesions of the orbitofrontal cortex are unable to discriminate odors. Interestingly, recordings in the orbitofrontal cortex suggest that some individual neurons in that area receive multimodal input and can respond, for example, to the smell, sight, and taste of a banana.
Most areas of the olfactory cortex also relay information to the lateral hypothalamus, an area important in appetite. In addition, studies of rodents indicate that one part of the olfactory cortex, the anterior cortical nucleus of the amygdala, transmits information to other areas of the amygdala and to more anterior regions of the hypothalamus, including those involved in reproduction. These limbic areas are thought to mediate the emotional and motivational aspects of smell as well as many of the behavioral and physiological effects of odorants. In animals they may be important in the generation of stereotyped behavioral and physiological responses to odors of predators or to pheromones that are detected in the olfactory epithelium.
Olfactory Acuity Varies in Humans
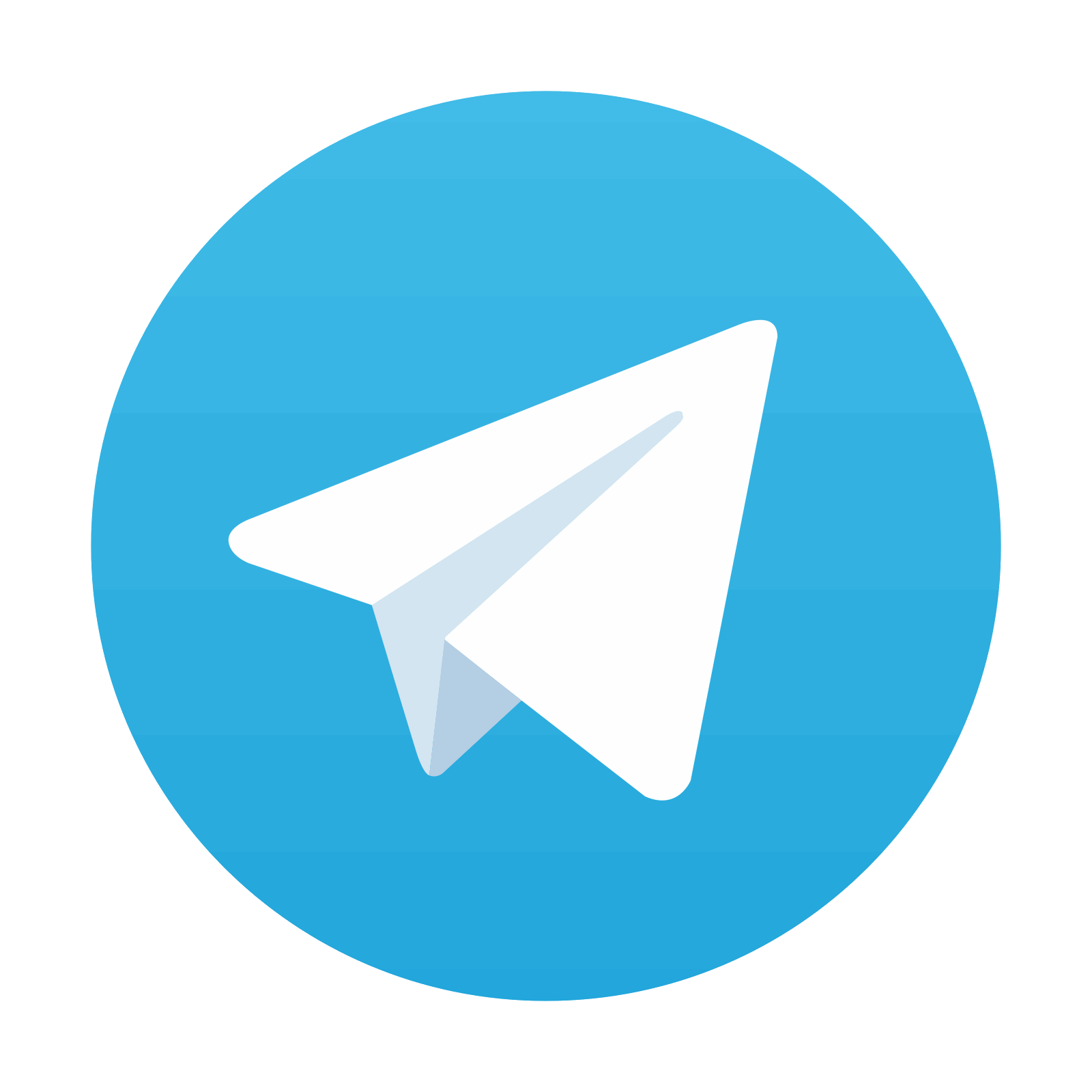
Stay updated, free articles. Join our Telegram channel

Full access? Get Clinical Tree
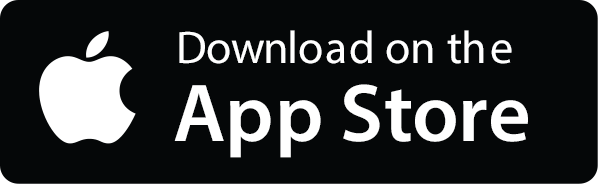
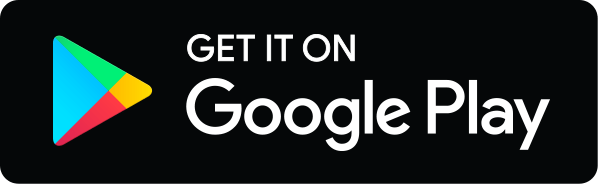