© Springer-Verlag Berlin Heidelberg 2015
Suayib Yalcin and Kjell Öberg (eds.)Neuroendocrine Tumours10.1007/978-3-662-45215-8_3030. Somatostatin Analogs and Interferon in the Treatment of Neuroendocrine Tumors
(1)
Neuroendocrine Tumor Program, H. Lee Moffitt Cancer Center and Research Institute, Tampa, FL 33629, USA
Keywords
Neuroendocrine tumorCarcinoid tumorCarcinoid syndromeInterferonOctreotideLanreotide30.1 Introduction
Biotherapy for neuroendocrine tumors (NET) emerged in the 1980s when somatostatin analogs (SSAs) and interferons (IFNs) were investigated in small series of patients with malignant carcinoid syndrome. While early clinical trials focused on palliation of hormonal symptoms, subsequent studies have been designed to assess the inhibitory effects of SSAs and IFNs on tumor progression. The recognition that biological therapies may be synergistic has led to several randomized studies investigating IFNα in combination with SSAs. This chapter will review the mechanism of action and key clinical trials investigating SSAs and IFNs in advanced, well-differentiated NETs.
30.2 Somatostatin Analogs
Native human somatostatin is a cyclic peptide hormone that exists in two natural forms consisting of 14 and 28 amino acids [1, 2]. It is widely distributed in multiple tissues including the central nervous system, pancreas, and gastrointestinal tract. It has been characterized as a universal endocrine “off switch” due to its inhibitory paracrine, exocrine, and endocrine effects. In the digestive tract, somatostatin inhibits multiple intestinal functions such as bowel motility and absorption [3]. Somatostatin also inhibits secretion of other pancreatic and intestinal hormones including gastrin, glucagon, and serotonin. The physiological actions of somatostatin are mediated through five subtypes of somatostatin receptors (sst1-sst5) belonging to a family of G-protein-coupled receptors with 7 transmembrane domains [4]. Approximately 80 % of well-differentiated NETs express high levels of somatostatin receptors, particularly receptor subtypes 2 and 5 [5].
Native somatostatin is rapidly cleared in the circulation due to enzymatic cleavage sites, limiting its clinical utility [5, 6]. In order to improve somatostatin’s pharmacokinetics, synthetic SSAs have been developed by truncating the polypeptide chain while retaining somatostatin receptor binding sites [7, 8]. Two commercially available analogs, octreotide and lanreotide, are cyclic peptide SSAs which bind with high affinity to sst2 and with moderate affinity to sst3 and sst5. SSAs have had a profound impact on neuroendocrine tumor treatment. They were initially developed as antisecretory agents designed to control symptoms such as flushing and diarrhea associated with the carcinoid syndrome. In recent years, high-level data has emerged demonstrating that SSAs can also control neuroendocrine tumor growth [9–11].
The initial formulation of octreotide was as a subcutaneously administered drug with an elimination half-life of approximately 2 h. A landmark phase II trial evaluated octreotide 150 mcg t.i.d. in 25 patients with malignant carcinoid syndrome [12]. Rapid palliation of flushing and diarrhea was reported in 22 patients (88 %), associated with major reductions of urine 5-HIAA in 18 patients (72 %). Multiple subsequent studies have confirmed that both octreotide and lanreotide are effective for palliation of the carcinoid syndrome [13–15]. A crossover study of 33 patients comparing octreotide to lanreotide demonstrated equivalency in control of flushing and diarrhea as well as improvement in quality of life [13]. Both drugs are also effective at controlling hormonal symptoms associated with pancreatic neuroendocrine tumors such as VIPomas, glucagonomas, and gastrinomas [16–20]. Patients with advanced insulinomas may also respond to SSAs [21]; however, there is potential for exacerbation of hypoglycemia due to inhibition of the counter-regulatory hormone glucagon [22].
During the past decade, a long-acting repeatable (LAR) depot formulation of octreotide (Sandostatin LAR®) has been available, which releases drug slowly by cleavage of a polymer ester linkage and enables monthly intramuscular dosing. A randomized phase III study compared subcutaneous octreotide every 8 h to octreotide LAR 10, 20, or 30 mg every 4 weeks [23]. Control of diarrhea was comparable in each of the four study arms; however, control of flushing was least effective in the 10 mg LAR cohort. Based on this trial, the recommended starting dose of octreotide LAR was set at 20 mg every 4 weeks with subsequent dose titration suggested for symptom control [24]. A second somatostatin analog, lanreotide, was licensed in Europe in 1998 for the treatment of hormonal symptoms-advanced NETs. A long-acting depot formulation of lanreotide (Somatuline Autogel®) has also been developed as a deep subcutaneous injection. It is supplied as a prefilled syringe consisting of a viscous aqueous solution available at doses of 60, 90, and 120 mg every 4 weeks.
Side effects of SSAs are generally mild and include nausea, bloating, and steatorrhea. Most side effects resolve over time. Malabsorptive symptoms caused by SSAs can often be controlled with pancreatic enzyme supplementation. Inhibitory effects of SSAs on gallbladder function increase the long-term risk of gallstones. Therefore, prophylactic cholecystectomy should be considered in patients undergoing abdominal surgery [25]. Other reported side effects include bradycardia, pancreatitis, and hyperglycemia. However, it is important to note that the only randomized trial of octreotide LAR versus placebo did not identify any increase in serious adverse events [26].
30.2.1 Treatment and Prophylaxis of the Carcinoid Crisis
Carcinoid crisis is defined as an episode of severe hypotension caused by an acute release of serotonin and other vasoactive substances into the circulation. It occurs most commonly in the operative setting since triggers include general anesthesia, epinephrine, and physical manipulation of tumors. Patients with carcinoid syndrome or elevated urine 5-HIAA who undergo an invasive procedure should receive a supplementary dose of octreotide 250–500 mcg subcutaneously or intravenously 1 h prior to the procedure [27]. Hypotension occurring in the setting of carcinoid crisis can be managed with bolus intravenous doses of octreotide 500–1,000 mcg until control of symptoms is achieved. Alternatively, continuous intravenous infusion of 50–10 mcg/h may be given after a bolus dose [28].
30.2.2 Antiproliferative Effects of SSAs
In recent years, strong evidence has emerged that SSAs can inhibit the growth of neuroendocrine tumors. Antitumoral activity, also known as the “antiproliferative effect” of SSAs, can occur via direct and indirect mechanisms [6, 9, 29]. Direct mechanisms involve the binding of SSAs to somatostatin receptors on tumor cells leading to activation of intracellular signaling transduction pathways. Multiple in vitro studies using cell lines transfected with somatostatin receptors indicate that all receptor subtypes (sst1-5) can participate in inhibition of cellular proliferation and that specific receptor subtypes (sst2,3) mediate apoptosis [30, 31]. Indirect antiproliferative mechanisms include inhibition of circulating growth factors such as vascular-endothelial growth factor (VEGF) and insulin-like growth factor (IGF) [32], as well as inhibition of tumor angiogenesis through interaction with somatostatin receptors on endothelial cells [33].
30.2.2.1 Direct Antiproliferative Mechanisms
In vitro studies in various cell lines, including breast, pancreatic, and prostate carcinomas, demonstrate that SSA binding to somatostatin receptors activates a subset of phosphotyrosine phosphatases (PTPs), including SHP-1 and SHP-2, leading to cell cycle arrest [34, 35]. In a pituitary adenoma cell line, activation of sst2 arrested cell proliferation via stimulation of SHP-1 [36]. SHP-1 has also been implicated in sst3-dependent apoptosis in transfected Chinese hamster ovary (CHO) cells [37]. The precise effects of SSAs on mitogen-activated protein kinase (MAP-kinase) signaling are uncertain since both inhibition and stimulation of this pathway have been linked to the antiproliferative effects of SSAs. In a glioma cell line, the receptor-like PTP, PTPeta, mediated the antiproliferative effects of somatostatin through inhibition of ERK1/2 [38]. In another study, somatostatin activated MAP-kinase in sst1-expressing CHO cells, thereby stimulating the cyclin-dependent kinase inhibitor p21 and inhibiting cell proliferation [39].
30.2.2.2 Indirect Antiproliferative Mechanisms
Suppression of tumor growth may occur through inhibition of various circulating growth factors, including growth hormone (GH) [40]. Inhibition of GH is thought to be mediated through sst2 and sst5, which are expressed in the anterior pituitary [41]. Octreotide has been shown to suppress circulating levels of IGF-1, both by suppressing pituitary GH and by inhibiting IGF-1 production in the liver [32, 42]. SSAs also appear to have an inhibitory effect on tumor angiogenesis [33, 43]. Octreotide has been shown to inhibit proliferating endothelial cells that express sst2 and sst5 [44]. The primary antiangiogenic mechanism may be suppression of endothelial nitric oxide release [45]. Inhibition of circulating vascular-endothelial growth factor (VEGF) appears to also play a role in suppression of peritumoral vessel growth [46].
30.2.3 Clinical Evidence of the Antiproliferative Effect
Multiple phase II and retrospective studies have investigated the effects of SSAs on tumor progression in patients with GEP-NETs. In general, these studies have documented low rates of objective radiographic response (<5 %) but high rates of disease stabilization [11, 14, 47–50]. One of the first prospective studies to report on the antiproliferative effects of SSAs in GEP-NETs was conducted by the German Sandostatin Study Group [51]. In this study, 103 patients with GEP-NETs were treated with octreotide 200 μg t.i.d. until evidence of radiographic progression. Among patients who had disease progression documented at treatment outset, 37 % experienced disease stability lasting at least 3 months, whereas among patients with stable disease at baseline, disease stability lasting at least 12 months was documented in 54 % of patients. Another phase II clinical trial testing octreotide in 34 patients with progressive metastatic NETs reported disease stabilization rate in 50 % of patients lasting a median of 5 months [49].
The antiproliferative activity of lanreotide was tested in several phase II clinical trials. In a study of 55 patients with GEP-NETs, 7 % of 31 assessable patients achieved a partial response to lanreotide, and 81 % experienced disease stability [17]. In another study of 46 patients with GEP-NETs, 2 patients (4 %) achieved an objective radiographic response, while 19 patients (41 %) had stable disease for a mean duration of 9.5 months [11]. In one study of patients with progressive tumors, participants received either octreotide LAR 30 mg or lanreotide SR 60 mg. Among 31 assessable patients, 14 (45 %) achieved disease stability [52]. In multivariate analysis, tumor control appeared to be significantly higher in small bowel NETs compared to pancreatic NETs. Extrahepatic metastases were associated with a poorer prognosis.
Definitive evidence of the antiproliferative effect of SSAs emerged with the PROMID trial, the first randomized placebo-controlled study performed in the field of neuroendocrine tumors [26]. Eighty-five patients with metastatic well-differentiated NETs originating in midgut were randomized to receive either octreotide LAR 30 mg or placebo. Patients with severe uncontrolled carcinoid syndrome were excluded. The primary endpoint was time-to-tumor progression. Median time-to-tumor progression (TTP) was 14.3 months in the octreotide LAR 30 mg group versus 6.0 months in the placebo group (P = 0.000072). Serious adverse events were nearly evenly balanced (11 patients in the octreotide LAR 30 mg arm and 10 patients in the placebo arm). On multivariate analysis, patients with resected primary tumor and low hepatic tumor burden (<10 %) appeared to benefit most significantly from octreotide treatment versus placebo. The small number of deaths in each treatment arm and the high rate of post-trial crossover precluded any analysis of differences in overall survival. Based on the PROMID data, octreotide LAR therapy is now considered an appropriate first-line treatment for patients with metastatic midgut NETs that are not surgically resectable, regardless of presence or absence of the carcinoid syndrome.
Non-midgut NETs tend to progress more aggressively than midgut NETs and are less likely to express somatostatin receptors. It is still uncertain whether SSAs are appropriate for use as antiproliferative agents in non-midgut NETs. The CLARINET study (clinical trial on nonfunctioning enteropancreatic endocrine tumors) is intended to address this question by randomizing patients with hormonally nonfunctioning GEP-NETs to treatment with depot-lanreotide versus placebo. The target enrollment of 200 patients has been achieved, and results are pending [53].
30.2.4 Above-Label Doses of SSAs
While the highest dose of octreotide LAR tested prospectively in patients with metastatic NET has been 30 mg every 4 weeks, higher doses and/or frequencies are often prescribed for patients with suboptimal control of hormonal syndromes. Data supporting this practice derive primarily from retrospective studies. In a longitudinal database conducted by the National Comprehensive Cancer Network (NCCN) encompassing 7 cancer centers, 40 % of carcinoid tumor patients and 23 % of pancreatic NET patients who received SSAs underwent dose escalations beyond 30 mg every 4 weeks [54]. The most common dose/frequency combinations were 40 mg every 4 weeks and 40 mg every 3 weeks. In one retrospective institutional study, 100 patients out of a total of 338 with metastatic midgut NETs (30 %) received octreotide LAR at doses and/or frequency above the standard label dose of 30 mg every 4 weeks [55]. Indications for dose increase were worsening carcinoid syndrome (60 patients), radiographic progression of tumors (33 patients), and rising urine 5-HIAA (6 patients). Among patients whose doses were increased for refractory carcinoid syndrome, 34 patients (62 %) experienced improvement in diarrhea, and 28 patients (56 %) experienced improvements in flushing. A single prospective trial investigated octreotide LAR 30 mg every 3 weeks among 28 patients with disease progression on 30 mg every 4 weeks [56]. Complete and partial control of clinical symptoms were observed in 40 and 60 % of treated patients, respectively.
30.2.5 Novel Somatostatin Analogs
Pasireotide is a novel somatostatin analog which binds avidly to four of the five somatostatin receptors (sst1,2,3 and sst5) [57]. Compared with octreotide, pasireotide has a 40-, 30-, and 5-fold higher binding affinity for sst5, sst1, and sst3, but a 2.5-fold lower affinity for sst2. An open-label trial evaluated the activity of short-acting subcutaneous pasireotide in 45 patients with carcinoid syndrome whose symptoms (flushing and diarrhea) were suboptimally controlled with octreotide LAR [58]. In this trial, 27 % of patients experienced some degree of improvement in their symptoms. However, a randomized phase III trial of long-acting intramuscular pasireotide vs. octreotide LAR in patients with refractory carcinoid syndrome was halted at interim analysis for futility [59]. The main toxicity observed with pasireotide is hyperglycemia, caused by suppression of insulin secretion which is mediated through the binding of pasireotide to sst5. In the phase III trial of pasireotide vs. octreotide, Grade 3 or 4 hyperglycemic events occurred in 11 % of patients in the pasireotide arm vs. 0 % in the octreotide arm. Clinical trials are currently testing the antiproliferative effects of pasireotide in advanced GEP-NETs.
30.3 Interferon
Interferon-α (IFNα) was first introduced to treatment of midgut NETs in 1983 when a small study reported symptom palliation in 6 patients with carcinoid syndrome treated at doses of three to six million units daily [60]. Subsequent single-arm studies have reported objective response radiographic rates in the range of 5–10 %, with higher rates of disease stabilization and palliation of hormonal symptoms [10, 61, 62]. More recently, randomized studies have offered evidence for the antiproliferative activity of interferon combined with somatostatin analogs.
30.3.1 Mechanisms of Action
Interferons are a complex array of cytokines with antiviral and antitumoral properties. They are divided into several classes. Type I interferons consist of IFNα (leukocyte interferon) and IFNβ (fibroblast interferon) which bind to a unique interferon receptor [63]. IFNs activate STAT (signal transducer and activator of transcription) complexes including the Janus-kinase-STAT (JAK-STAT) signaling pathway leading to signaling cascades involving the tumor cell and the host immune system [64]. Immune-related mechanisms of action include induction of major histocompatibility complex (MHC) type I expression in tumor cells which leads to enhanced immune surveillance. Other effects of IFNs include promotion of dendritic cell proliferation and differentiation and alterations of T-cell CD4:CD8 ratios [65]. Angiogenesis inhibition appears to be another indirect mechanism of action. In a study of neuroendocrine tumor xenografts in nude mice, IFNα treatment reduced microvessel density and suppressed vascular-endothelial growth factor (VEGF) gene expression [66].
IFNs may also exert a direct antiproliferative effect on tumor growth through induction of cell cycle arrest; however, the precise mechanism remains elusive. In one in vitro study of lymphoid cell lines, G0/G1 cell cycle arrest was mediated through induction of the cyclin-dependent kinase inhibitors (CKIs) p21 and p15 and the expression of p27 [67]. However, another in vitro study of neuroendocrine tumor cells demonstrated that IFNα delayed progression from S-phase to G2 due to reduction of cyclin B levels and inhibition of Cdc2 kinase activity [68]. A third study demonstrated that IFNα induced apoptosis through activation of caspases-1, -2, -3, -8, and -9 in a variety of malignant cell lines [69].
30.3.2 Studies of IFNα Monotherapy
An early study of IFNα three to six million units/day reported objective tumor responses in 4 of 36 patients (11 %) with advanced carcinoid tumors and major reductions in urine 5-HIAA in 16 patients (53 %) [70]. Another study of 22 patients with pancreatic NETs reported high rates of symptomatic and biochemical responses [71]. A more recent phase II study of 49 patients with advanced NETs evaluated IFNα-2a at a daily dose of six million units daily for 8 weeks followed by the same dose 3 times weekly thereafter [72]. Among 34 patients with carcinoid tumors, partial radiographic responses were observed in 4 patients (12 %), and major improvements in carcinoid syndrome were reported in 64 % of cases. The most frequently observed side effects were fever, flu-like symptoms, and leukopenia. In the United States, Moertel et al. treated 27 patients with metastatic carcinoid tumors with IFNα at a high dose of 24 million units/m2 [73]. Despite an objective radiographic response rate of 20 % and major urine 5-HIAA reductions in 39 % of patients, the authors concluded that any therapeutic gains seemed to be outweighed by severity of side effects which included fevers/chills, anorexia, weight loss, leukopenia, and liver function abnormalities.
30.3.3 Studies of IFNα Combined with SSAs
Studies evaluating the combination of IFNα and SSAs emerged in the 1990s, based on in vitro data demonstrating that interferons can upregulate the expression of somatostatin receptors. One trial of patients with carcinoid syndrome who had become refractory to octreotide reported symptomatic improvement in 49 % of patients after addition of IFNα [74]. Another study reported disease stabilization in 14 of 21 patients in whom IFNα was added to preexisting octreotide therapy [75]. A more recent trial investigated pegylated IFN (PEGIFN), a long-acting formulation which enables weekly dosing. Of 17 patients who progressed on SSA therapy, inhibition of tumor growth was observed in 13 patients. The side effect profile of PEGIFN appeared to be superior to that of IFNα [76].
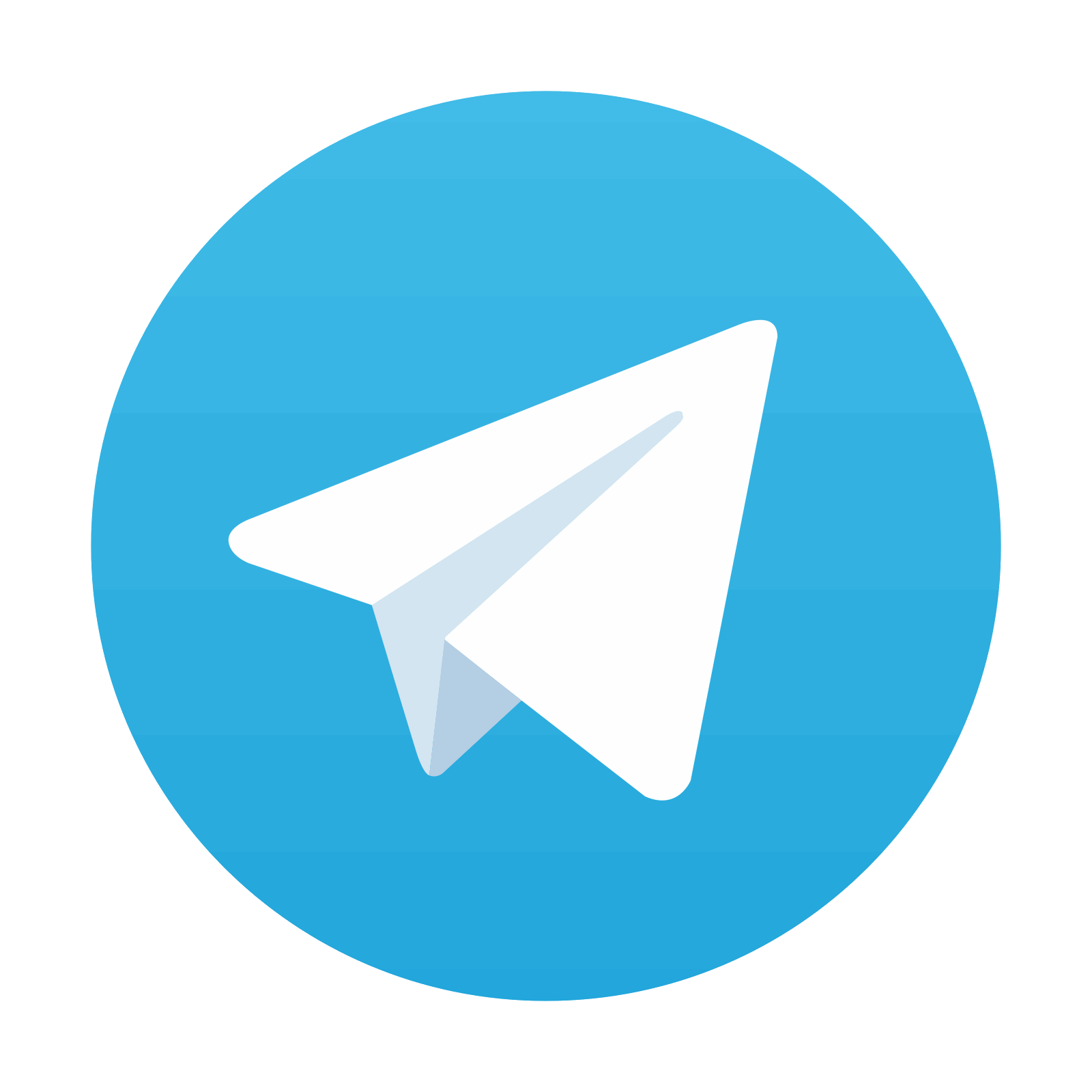
Stay updated, free articles. Join our Telegram channel

Full access? Get Clinical Tree
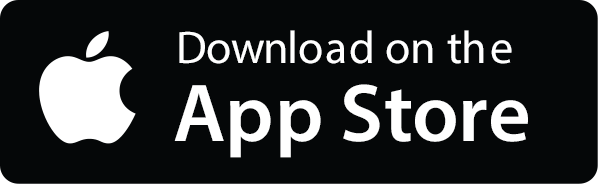
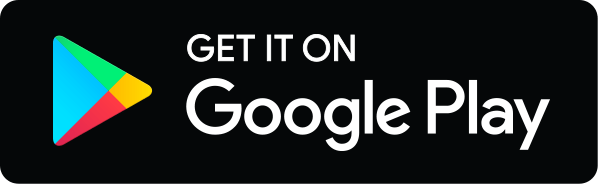