Spinal Cord Injury
Trauma is the leading cause of death and disability in the pediatric population. Spinal cord injuries, despite involving only 1 to 2% of all pediatric trauma cases, can be incredibly debilitating, requiring a lifetime of care and utilization of medical resources.1–7 Spinal cord injury (SCI) affects approximately 2 per 100,000 children per year, and the National Spinal Cord Injury Statistical Center (NSCISC) found in 2010 that 9.86% of patients with SCI were younger than 18 years.8,9 The vast majority of injuries involve the cervical spinal cord, whereas only 5.4 to 34% involve the thoracolumbar spine.1,8,10,11
The pediatric spine is not merely a miniature version of the adult spine. Instead, the developing spinal column is inherently more elastic and mobile. The hypermobility of the pediatric spine provides less protection to the fragile, underlying spinal cord.12 A newborn spine and a 14-year-old spine lie along a biomechanical continuum that becomes less mobile and more rigid as a child transitions into late adolescence and adulthood. The differences between adults and children are not limited to biomechanics; rather, there are clinically important differences in regard to injury pattern, treatment, rehabilitation, and outcomes.
62.1 Epidemiology
The epidemiology of pediatric SCI is difficult to characterize fully because of the limited available data on this patient population.13–15 The extant literature contains few studies that include pediatric patients, typically excluding those treated at adult institutions, who die before arrival at a hospital, or who have birth-related SCI. Potential evidence as to the degree of underreporting in children can be extrapolated from adult data. Most authorities illustrate the increased susceptibility of the pediatric spine to injury. Milby et al, in a pooled analysis of 281,864 adult patients with trauma, found a prevalence of SCI of 3.7% in the adult population.16 One would expect that pediatric patients with trauma would have an incidence of SCI above 1%.
The incidence of SCI is approximately 1.99 to 7.4 per 100,000, and approximately 1,455 children are admitted each year in the United States with SCI.8,17–19 The prevalence within the pediatric population is poorly characterized but can be estimated based on age at injury. The prevalence of SCI in both adults and children is between 223 and 1,009 per million inhabitants, with fewer than 10% of injuries occurring before the age of 18 years.13,14 Lasfargues et al demonstrated the prevalence of SCI in the United States in 1988 to be 176,697, with 11,745 (6.6%) of patients younger than 24 years.20 The NSCISC found in 2010 that 9.86% of patients with SCI were younger than 18 years.9 The same data demonstrated that only 1% of spinal cord injuries occurred in patients younger than 13 years. There is an obvious age distribution, with a mean age of 10.3 to 13.5 years and a median age closer to 16 years.6,21 Younger patients have a lower rate of SCI, and the incidence rapidly increases with age. Although motor vehicle accidents remain the leading cause of SCI across all age groups, the age of the victim is also pertinent to the mechanism of injury. Older persons tend to engage in higher-risk activities, whereas younger people are more susceptible to nonaccidental trauma. The gender distribution tends to be more even in younger patients, with the male-to-female ratio of persons with injuries increasing toward adolescence and adulthood.4
Pediatric SCI is more common in males, with reported male-to-female ratios as high as 3:1.4,8,21–24 The gender distribution is a result of increased risk-taking behavior as children age. Sports-related injuries are rarely seen before the age of 9 years. After 9 years of age, sports-related injuries contribute to approximately 25% of pediatric cases of SCI in the United States, and most of these occur in males.21 Brown et al demonstrated that the numbers of males and females involved in motor vehicle collisions were almost equal, with a male-to-female ratio of 1.2:1, but for sports-related injuries, the ratio was 3.5:1.21
Race also appears to play a role in the epidemiology of pediatric SCI. In the United States, Caucasians have the highest rate and account for the majority of cases of SCI. African Americans exhibit the second highest rate of SCI (1.53 per 100,000), followed by Native Americans (1.00 per 100,000), Hispanics (0.87 per 100,000), and Asians (0.36 per 100,000).8,19
Pediatric SCI is a rare disease entity and as such is not a major international public health concern. Consequently, few geographic data exist outside the developed world. Several interesting cultural trends are apparent from the available literature. Cripps et al performed a global epidemiologic review of SCI and made several observations. In Turkey, a high percentage of SCIs occur after falls from rooftops because children often sleep on the roof in summer.13 Southern Asia, specifically Nepal and Pakistan, have a similarly high incidence of falls from rooftops and trees that result in SCI.13,25 In Greece and Italy, young males are known for engaging in high-risk driving behaviors that place them at increased risk for SCI.7,26 Epidemiologic studies in the pediatric population would be most helpful in terms of directing future research into prevention and management of patients with SCI.
62.2 Biomechanics and Anatomy
The biomechanics of the developing spine and spinal cord have been the subject of much discussion but little research because of the difficulty in conducting the studies in children. Nonetheless, the developmental anatomy and subsequent biomechanical differences in the pediatric spine are felt to play a central role in the understanding of pediatric spine trauma.
Embryology is always a fitting starting point for understanding any anatomical process. The developing fetal spine derives from paraxial mesoderm that is located lateral to the neural tube. The paraxial mesoderm develops into paired somites that then form the axial skeleton and its associated ligaments, tendons, and muscles.27,28 The demarcation of individual vertebrae takes place through a process known as resegmentation, in which the anterior and posterior portions of adjacent somites fuse.27,29,30 Each vertebral body is thus formed from two sclerotomes, unlike the peripheral nervous system. The intervertebral disks are formed at the boundary between the anterior and posterior sclerotomal portions of the somite.28 The development of the spine begins in utero and continues until early adulthood.
Ossification of the atlas and axis is unique compared with that in the remainder of the subaxial spine. The anterior arch of C1 is typically ossified by the age of 1 year, and complete ossification of the posterior arch occurs around the age of 3 years.31 The atlas achieves a normal adult radiographic appearance by the age of 7 years.32 The axis is more complex in that there are a total of six ossification centers (unlike the typical four at other spinal levels); these are involved in the formation of the odontoid process. The odontoid process begins to fuse to the body of C2 between the ages of 3 and 6 years.32 This process is normally completed by the age of 11 years.32 Ossiculum terminale is a condition in which the secondary ossification center at the apical portion of the odontoid does not fuse by the age of 12 years.33 Ossiculum terminale is commonly seen on adult radiographs and usually carries no clinical significance, although several case reports suggest there may be an association with atlantoaxial instability.34 Mortazavi et al noted that the C2 posterior arch fuses in the midline by the age of 3 years and with body between the ages of 3 and 6 years.33
Os odontoideum, a nonunion of the synchrondosis of the odontoid process that is associated with pathologic instability, is clinically distinct from ossiculum terminale. Radiographically, the two can be differentiated by the location of nonunion. Os odontoideum is considered by most authorities to be inherently unstable regardless of findings on dynamic plain films. Klimo et al described three patients with known os odontoideum who were followed nonoperatively and experienced SCI.35 Surgical management is thus recommended to minimize risk for subsequent SCI (see box “▶ Predisposing Conditions for Spinal Cord Injury”).
Predisposing Conditions for Spinal Cord Injury
Congenital cervical stenosis
Down syndrome
Morquio syndrome
Chiari type 1 malformation
Os odontoideum
The subaxial cervical spine from C3 to C7 typically follows a very similar ossification pattern. The ossification of the posterior arch follows the same pattern as C2, and the posterior arch is typically fused with the body by age 6. Complete ossification typically does not occur until age 25.32 Central to an understanding of spinal radiographs in children is the ability to recognize epiphyseal variants, incomplete ossification of synchondroses and apophyses, unique vertebral architecture, pseudosubluxation of C2 on C3, overriding of the anterior atlas in relation to the odontoid in extension, and varied atlantodental intervals.36–40 The pediatric spine is thus unique in terms of stability, injury patterns, clinical and radiographic evaluation, and treatment (▶ Fig. 62.1a–c).
Fig. 62.1 An 18-month-old girl in a forward-facing car seat during a motor vehicle collision. (a,b) The patient presented with an American Spinal Injury Association (ASIA) class A synchondrosis fracture of C2 that was placed in a halo. (c) The patient subsequently underwent internal fixation with Mersilene (Ethicon, Somerville, NJ) tape between C1 and C2.
The stability of the spine is a function of the connecting ligaments, facets and articular capsules, intervertebral disks, and associated musculature. The increased proportional weight of a child’s head with respect the remainder of the body increases the mechanical strain on the cervical spine during extremes of flexion, extension, rotation, and distraction.12,41,42 The neck acts as a fulcrum, and the larger head effectively lengthens the moment arm, thus increasing the force placed upon the underdeveloped cervical spine. In addition to the increased forces acting on the spine, the pediatric cervical diskoligamentous complex has been shown to be considerably more elastic than that in adults.32,38,42–45 The ramifications of increased elasticity are that the spine can bend but not break during trauma. Unfortunately, the spinal cord does not well tolerate stretching and is thus felt to be more susceptible in the pediatric population. The ligaments, joint capsules, and intervertebral disks have different characteristics, as does the bony anatomy of the articulations themselves.
The occipitoatlantoaxial joints are an incredibly complex bony and ligamentous region of the most cephalic portion of the spinal column and are involved in many pediatric SCIs. The occipital condyles, although infrequently fractured, have associated ligamentous structures involved in atlanto-occipital dislocation.46,47 Additionally, the shape of the occipital condyle and the shallow articulation with the lateral mass of C1 put the vertebral artery at risk for injury during hyperextension.48
Pang and Zovickian have written several overviews of the morphometric differences between the adult and pediatric cervical spine that have implications with regard to spinal cord injuries.12,42 They explain that the facet joints are “… shallow and oriented more horizontally than in adults,” and this is felt to increase the potential for unintended translation.12,42 The potential for forward translation is also increased in children by the anterior wedging of vertebral bodies.32,42–44 The uncovertebral joints (joints of Luschka) are stabilizing joints of the subaxial spine that chiefly limit lateral movement.49 Orofino et al studied the uncovertebral joints of 11-week-old fetuses to full-term infants and found no synovial tissue at the future site of the uncinate process, confirming observations in adults that the uncovertebral joints are not true synovial joints.50 Despite not being true synovial joints, unlike the facets, they do provide a mechanical advantage in preventing lateral movement and a degree of rotation.33,51 The uncinate processes and uncovertebral joints are not formed until around the age of 10 years and continue to develop into adulthood.49,52 The incompletely developed subaxial spine contributes to instability and increases the risk for SCI in children.
The diameter of the spinal canal is another important consideration. According to Steel’s “rule of thirds,” which was proposed in 1968, the spinal canal at C1 is filled with (1) odontoid process, (2) spinal cord, and (3) cerebrospinal fluid “dead space” in equal proportions.53–55 Jauregui et al found that Steel’s rule of thirds is maintained throughout childhood, with the average diameter available for the spinal cord in neonates being approximately 12.4 mm.53 The median diameter of the canal at C2 was found by Wang et al to increase from between 12.27 and 12.79 mm at 6 months to between 15.75 and 16.00 mm at 13 years.31 After 13 years of age, the diameter of the spinal canal stabilizes to the adult range. When the smaller canal diameter is coupled with forces that result in hyperextension, the spinal canal diameter can be further decreased by up to 50% simply from the inward buckling of the ligamentum flavum.12,56–58
62.3 Thoracolumbar Injuries
Thoracolumbar fractures most often occur in older children and infrequently lead to SCI (14.6%).11 Dogan et al reviewed 89 patients with thoracolumbar injuries and found motor vehicle accidents to be the most common mechanism.11 The classification of thoracolumbar fractures in the pediatric population is not characterized, but the thoracolumbar injury classification and severity score (TLICS) may be used.59,60 For instance, purely ligamentous injuries (1.1%) without any bony involvement are classified as distracting injuries with interruption of the posterior ligamentous complex. The utility of the TLICS system in children is the consideration of ligamentous involvement and the neurologic examination (see box “▶ Thoracolumbar Injury Classification and Severity Score” and ▶ Fig. 62.2a,b).
Thoracolumbar Injury Classification and Severity Score
Injury morphology
Compression
Burst
Translational/rotational
Distraction
Integrity of posterior ligamentous complex
Intact
Suspected/indeterminate
Injured
Neurologic status
Intact
Nerve root
Complete: American Spinal Injury Association (ASIA) class A
Incomplete
Cauda equina syndrome
Fig. 62.2 An 11-year-old girl unrestrained in a motor vehicle collision presented with an American Spinal Injury Association (ASIA) class A injury at the T7 level. (a) Her thoracolumbar injury classification and severity score (TLICS) was as follows: morphology, 3; posterior ligamentous injury, 3; neurologic injury, 2. (b) The patient underwent decompression, internal fixation, and fusion. She now ambulates with a walker (ASIA class D) and has normal bowel and bladder function.
62.4 Birth Injuries
Birth-related SCI is rare and is estimated to affect approximately 1 in 60,000 live births.61–63 Birth injuries are difficult to diagnose and are a highly litigated form of pediatric SCIs.63–65 The neurologic examination in a neonate is difficult. Normal reflexes can mask an underlying complete cord transection. Upper cervical SCI can present with respiratory insufficiency, hypotonia, and quadriplegia. Lower cervical SCI demonstrates abdominal breathing (due to paralyzed intercostal muscles) in addition to lower extremity hypotonia.63 An important and underappreciated element in the examination of a neonate is the facial reaction to painful stimuli. Reflexive withdrawal of the extremities without grimacing or crying is the hallmark of neonatal SCI. Additionally, Horner syndrome or brachial plexus injury provides evidence of a difficult delivery and potential SCI.
Not all cases of neonatal SCI occur during delivery; multiple reports in the literature document prenatal SCI.63,65–67 The management of birth-related SCI requires ascertaining a diagnosis and evaluating for potential operative intervention. Blount et al described a spontaneous cervical epidural hematoma that mimicked a birth-related SCI and later required surgical decompression.65
62.5 SCIWORA
SCI without radiographic abnormality (SCIWORA) is a subtype of SCI that was initially described by Pang and Wilberger in 1982.68 They described 24 patients with SCI on examination without imaging abnormalities on plain films, computed tomography (CT), or myelography. Magnetic resonance (MR) imaging, a nonradiographic form of imaging, is abnormal in approximately 15 to 65% of patients with SCIWORA.62,69 Thus, the presence of neural or extraneural abnormalities on MR imaging does not exclude the diagnosis, although the semantics of the term remain controversial. For instance, a patient with instability on flexion–extension radiographs does not have SCIWORA, but ligamentous hyperintensity on STIR (short tau inversion recovery) MR imaging suggesting instability would not preclude the diagnosis. Further standardization of the term will limit confusion in the literature and clinical setting. The incidence of SCIWORA in the pediatric population with SCI is 34.8% (range, 5 to 67%).70 As mentioned previously, the spinal column elasticity of younger children leads to a higher rate of SCIWORA.71
The 2012 Guidelines for the Management of Acute Cervical Spine and Spinal Cord Injuries, citing level 3 evidence, recommend that the initial evaluation of a patient with SCIWORA include MR imaging, radiographs of the entire spinal column, and flexion–extension plain films.69 Normal MR imaging portends a good outcome. Bosch et al and Pang independently reported complete recovery in all patients with SCIWORA who had normal MR imaging (n = 50).70,72 There are small case studies of patients with normal MR imaging and deficits persisting beyond 1 year.73 Pang further stratified findings into major hemorrhage, minor hemorrhage, and edema.70 The degree of SCI seen on MR imaging was directly related to outcome.
MR imaging findings can vary depending on patient age and on the level, mechanism, and extent of injury. MR imaging in the acute setting may identify cord signal change, compressive lesions, and/or ligamentous injury. Epidural hematomas, disk herniations, and other compressive lesions resulting in SCIWORA require operative decompression. MR imaging also can demonstrate ligamentous injuries, especially on T2 and STIR sequences, that suggest instability.72,74,75 Mortavazi et al restated the previous recommendations of Pollack et al from 1988 to repeat MR imaging 6 to 9 days after the initial injury.33,76 The quality of MR imaging and the sequences performed have changed significantly since 1988. Nonetheless, in a patient with normal initial MR imaging and a persistent neurologic deficit, repeated imaging is warranted (▶ Fig. 62.3a–c). Flexion–extension lateral radiographs are potential adjuncts to clearing the cervical spine and ruling out instability in SCIWORA. Paraspinous muscle spasms may prevent adequate flexion for several days after injury.77 Pang also recommends somatosensory evoked potential (SSEP) testing in all patients with SCIWORA, although the clinical utility is not well characterized in the literature.
Fig. 62.3 A 2-year-old boy after a motor vehicle collision with a T2 level American Spinal Injury Association (ASIA) class A injury and no discernible spinal column injury. (a) Image obtained less than 24 hours after injury. (b) Image obtained 9 days later demonstrates retraction of the severed cord ends. (c) Two years after injury, late instability had not developed.
The surgical treatment of patients with SCIWORA depends on the presence of compressive pathology and/or instability. The vast majority of patients, however, will not have a surgical indication. Activity restriction thus becomes important to promote recovery and prevent recurrent injury. Pollack et al studied 8 patients (19%) with recurrent SCIWORA and found most surgical indications to occur within the first week (62.5%) and all to occur within 10 weeks.76 As a result of this and similar studies, external immobilization is recommend for up to 12 weeks following injury, and patients are encouraged to avoid “high-risk” activities for 6 months.12,69,76
62.6 Initial Stabilization and Evaluation
The management of pediatric cervical spine injuries begins immediately at the scene of the accident. Education of the general public and emergency medical personnel is a vital adjunct to inpatient care. The first step in the initial evaluation of any trauma is management of the airway, breathing, and circulation. Maintaining adequate oxygenated perfusion to the injured spinal cord is a central tenet in management and should begin as quickly as possible.78 During the primary survey, the cervical spine should be held in in-line immobilization and manipulated as little as possible. A cervical collar of appropriate size should be placed, or the head should be immobilized with rolls. A collar that is too large will not provide adequate immobilization and may place the cervical spine in excessive distraction. Because the head-to-body ratio is larger in children than in adults, patients less than 8 years old should be placed on a back board with either an occipital recess or thoracic bolsters to avoid untoward cervical flexion and maintain neutral alignment.79,80 The proper initial treatment of a child with an unstable cervical spine may prevent secondary SCI.
Following the initial evaluation and stabilization, a more in-depth assessment of the patient must be performed. The clinical history and physical examination are paramount to medical decision making regarding radiographic evaluation and the potential treatment of any injuries. There is no level 1 evidence in pediatric spine trauma, but level 2 and 3 evidence provides some guidance on imaging.
In the absence of a neurologic deficit, patients who are awake, alert, communicative, without midline cervical tenderness, not intoxicated, and without painful distracting injuries typically do not need cervical spine imaging.6,33,69,81 Patients who do not fulfill these criteria, children younger than 3 years who are involved in motor vehicle collisions or falls from more than 10 feet (especially those with axial loads to the head), and suspected victims of nonaccidental trauma should undergo radiographic evaluation of the cervical spine.6,82,83 Visualization of the cervicothoracic junction may necessitate the use of a swimmer’s view if plain radiographs are obtained. In general, children younger than 3 are considered noncommunicative and according to Anderson et al require imaging following trauma.82,84 Viccellio et al performed the largest prospective randomized trial on the evaluation of pediatric cervical injuries. Use of the aforementioned criteria, outlined by the National Emergency X-Radiography Utilization Study (NEXUS), had a sensitivity and negative predictive value of 100% in ruling out pediatric cervical spine injuries.6,85,86 Flexion–extension plain films, CT, and MR imaging are all used adjunctively in the radiologic clearance of a pediatric cervical spine. Clearance of the cervical spine requires that both radiologic and clinical criteria be met.
Children with a neurologic deficit and suspected spinal cord injuries require CT and MR imaging evaluation. MR imaging is especially beneficial in obtunded patients with suspicious imaging and should include T2 and STIR sequences to evaluate for acute ligamentous injury and SCI.33,87,88 Additionally, radiographic evaluation of the remainder of the spine is recommended to avoid missed noncontiguous injuries that may not be clinically apparent because of the presence of a proximal SCI. Firth et al found that 11.8% of children with a spinal injury had a noncontiguous fracture.89
SSEPs and neurogenic evoked potentials are poorly studied in the pediatric trauma population. In patients with incomplete SCIs, they may have some diagnostic utility.69,70,90,91 Pang recommends the routine use of SSEP testing in patients with suspected SCI, especially in the setting of SCIWORA.70 He states that SSEP testing (1) detects subtle abnormalities in the posterior column conduction pathway when the clinical examination is inconclusive, (2) helps detect SCI in head-injured children, (3) is the only feasible assessment in comatose patients or those requiring neuromuscular blockade, and (4) is occasionally useful in distinguishing between brachial plexus, intracranial, and spinal cord injuries.70 Scarrow et al performed a pilot study in which flexion–extension plain films with concomitant SSEP testing were obtained during the clearance of cervical collars in obtunded patients.92 Only 1 of 15 patients studied had flexion–extension films and SSEPs that were both abnormal. This patient’s MR imaging was normal, and his collar was later clinically cleared. Four additional patients had abnormal SSEPs, but the one patient with a SCI had normal SSEPs. Further study is warranted to determine the utility of SSEP testing in the pediatric population.
Concomitant vascular injury may be associated with traumatic cervical SCI. The occurrence of traumatic carotid or vertebral artery dissections in children is very low.93 In the absence of penetrating injuries, CT or conventional angiography is not routinely indicated in the pediatric population.
62.7 Evidence-Based Treatment and Management
A definitive evidence-based approach to the management of pediatric SCIs does not exist. The injuries are rare, varied, and poorly studied in the literature. Nonetheless, these patients deserve high-quality care. The only way to reconcile our predicament is to use the available pediatric literature, make inferences based upon adult data, and draw from experience.
The pharmacologic treatment of spinal cord injuries, specifically the use of methylprednisolone, remains a controversial topic. The only randomized controlled trial to include children was the National Acute Spinal Cord Injury Study (NASCIS) II.94 The results of this trial did not specifically address outcome in the group of 13- to 19-year-old patients who were 14.9 to 15.4% of each of the three treatment arms. The stratification for age and outcome was not statistically significantly different, and the results of the trial have been the subject of considerable controversy. The initial outcome measures of the study were not met, but the authors described an a priori hypothesis that greater clinical improvement could be obtained with earlier initiation of the methylprednisolone treatment protocol.94 Bracken, the lead author of the three NASCIS trials, noted that the treatment effect of NASCIS II was relatively minor.95 The expected motor improvement, based on Japanese, French, and American randomized controlled trials, is unilateral improvement in the motor score of 4.1 points.64,94–97 The score used in these trials consisted of Medical Research Council (MRC) motor grades 0 through 5 in 14 motor groups (total score, 140 per patient). According to the post hoc analysis, a patient could be expected to have unilateral improvement of approximately one complete motor level.95 The effect is thus not nearly as definitive as the conclusions in the original articles or in the January 2012 Cochrane Review written by the same author.64,94,95,98–100 The latest (2008) adult guidelines of the Consortium for Spinal Cord Medicine, an organization to which the American Association of Neurological Surgeons and Congress of Neurological Surgeons belong, do not recommend starting steroids and actually recommend stopping steroids, if they were started at another facility, as soon as possible to avoid adverse complications.101
Wang et al studied 30 cases of SCI in children and found methylprednisolone administration to be unrelated to neurologic improvement (p = 0.31).102 It is important to note that only 8 children received steroids, and 5 (62.5%) had some neurologic improvement.102 The 2002 guidelines for the management of pediatric cervical spine and spinal cord injuries and the 2012 guidelines for the management of acute cervical spine and spinal cord injuries cite insufficient data to comment on steroid use in children.69,81 A well-powered and designed multicenter randomized controlled trial is necessary before methylprednisolone can be recommended for SCIs in the pediatric population.
Perfusion to the injured spinal cord is important during the management of acute injuries. The denervation of sympathetics, in addition to any systemic injuries sustained, may result in distributive hypovolemia, hypotension, and even neurogenic shock. Maintenance of an age-appropriate physiologic mean arterial blood pressures (MAP) is recommended in the treatment of any patient with trauma.103 Augmentation of the blood pressure has been advocated in patients with SCI to prevent secondary injury from hypoperfusion.78,104–108 The two principal studies for MAP management in adults both used methylprednisolone according to the NASCIS II recommendations, thus introducing a potential confounder.78,109 The extent of augmentation, method of hemodynamic monitoring, and duration of treatment are not characterized in the pediatric population.
The management of pediatric SCI should include placement of an arterial line and maintenance of an age-appropriate MAP. Treatment with vasopressors to elevate the blood pressure above the mean for age and height warrants further study before definitive recommendations can be made. An appropriate course of treatment would be to maintain the MAP at the 95th percentile for age and height over a 5- to 7-day period. Based on normative data from Haque and Zaritsky, assuming that both children were in the 50th percentile for height, a 1-year-old would be maintained at an MAP of 56 mm Hg, and a 16-year-old would be managed at an MAP of 85 mm Hg.103 The successful hemodynamic support of pediatric SCI depends on a multidisciplinary critical care team in close coordination with neurosurgery.
Operative decision making in pediatric SCI revolves around instability and spinal cord or nerve root compression. The timing of surgery is determined by the degree of instability and the presence of irreducible dislocations and incomplete injuries with or without progressive neurologic deterioration. The actual timing of decompression has been the subject of medical controversy and has far-reaching medicolegal implications. Fehlings et al demonstrated in the Surgical Timing in Acute Spinal Cord Injury Study (STASCIS) that the odds ratio for achieving an improvement of two grades on the American Spinal Injury Association (ASIA) Impairment Scale is 2.83 (95% confidence interval, 1.10–7.28) for SCI patients undergoing decompression within 24 versus 48 hours.110 The study included individuals past the age of 16, and there are no studies specifically looking at early decompression in children. Dimar et al reviewed 11 articles from 1990 to 2009 comparing early and late decompression.111 The medical literature demonstrated consistently shorter hospital and intensive care unit stays, fewer days on mechanical ventilators, fewer hospital-acquired cases of pneumonia and urinary tract infection, and no increase in operative complications with early versus late surgery.111
62.8 Rehabilitation and Outcomes
The early involvement of a multidisciplinary team is vitally important in the care of pediatric patients with SCI because patients are surviving longer. The overall mortality rate is between 2.5 and 28%.4,21,89,102,112,113 The wide range of mortality rates represents sampling error due to small population size, a high rate of concurrent traumatic brain injury in certain populations, and inclusion of deaths before admission. Hamilton and Myles reported a 28% mortality rate, with 89% of deaths occurring at the scene of the accident.113,114 They cited a pediatric-to-adult mortality ratio of 2.5:1. The cervical spine injuries of younger children tend to be more rostral, resulting in a higher mortality rate. Children involved in motor vehicle collisions have the highest overall mortality rates and the poorest outcomes.21,113 Public health initiatives to keep children properly restrained in motor vehicles are vitally important, as 87% of children who died of SCI were unrestrained.113 Every illustrative case within this chapter is from a motor vehicle collision in which the child was improperly restrained.
Brown et al found that the median age for nonsurvivors was 5.3 years, with 14 of 19 children (74%) sustaining a high cervical SCI.21 Eleraky et al demonstrated in a similarly sized study that children younger than 10 years sustained more spinal cord and upper cervical spine injuries (▶ Fig. 62.4). Despite a higher initial mortality rate than that in adults, children with SCI tend to have a more favorable long-term prognosis than adults with similar injuries.112
Fig. 62.4 Cervical spine injuries by age and location.
(Data from Eleraky et al.112 Illustration by Joseph H. Miller.)
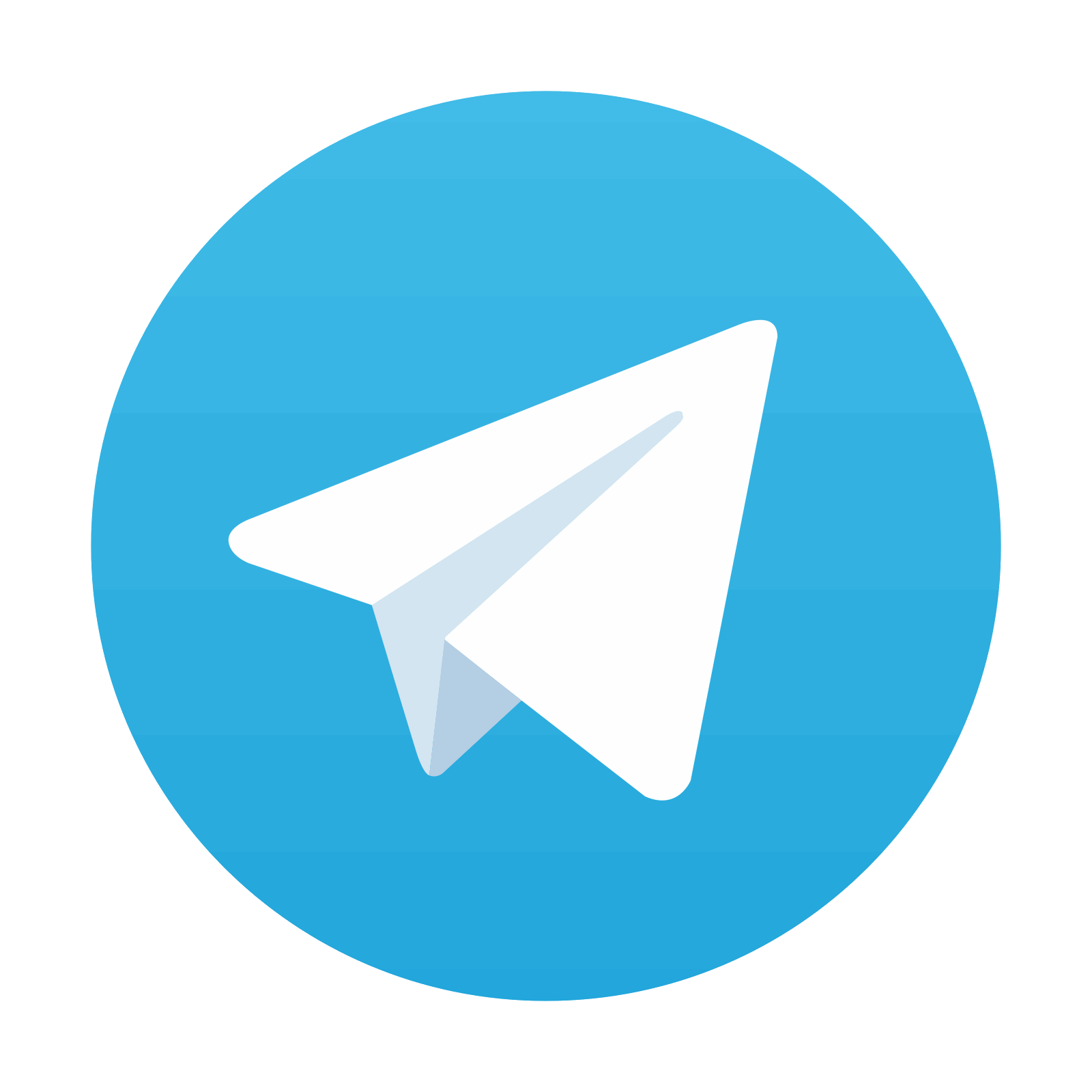