Spinal Cord Injury: The Role of Steroids
Ian A. Madom
Darrel S. Brodke
Over the past two decades, extensive basic science and clinical research have focused on treating the secondary injury cascade of acute spinal cord injury (SCI). This includes targeting factors that affect the spinal cord in the hours to weeks after an injury. It stands to reason that an intervention that reduces vasogenic edema, lessens neuron membrane excitability, eliminates free radicals, and inhibits the inflammatory response while improving cord blood flow may result in better patient outcomes after an SCI. It is these properties and early successes in animal trials that have led to the use of methylprednisolone sodium succinate (MPSS) in the setting of acute SCI. The clinical use of steroids in SCI patients rapidly grew after three large clinical trials supported their use for these patients and thus became part of the standard protocol for SCI treatment (1, 2 and 3). However, this remains controversial, as MPSS used in high doses carries many potential risks and the reported benefits are not clear after a critical review of the supporting literature. Additionally, it has been shown that these types of agents to be of limited benefit to patient outcomes. This chapter focuses on the use of MPSS, which is only one of the many potential treatments aimed at the secondary cascade that ensues following SCI. It reviews the early preclinical data that led to large clinical trials and how these data may not support the early conclusions regarding the utility of MPSS for SCI.
PROPERTIES OF STEROIDS AND EFFECT ON SCI
There are a variety of mechanisms leading to the initial injury, as well as its progression (Fig. 132.1). The treatment of the primary injury involves aggressive prevention of hypotension and hypoperfusion of the spinal cord via intravascular volume and blood pressure support. A significant amount of energy has been directed to considerations regarding secondary mechanisms that occur in the acute to subacute time frame after injury. One such intervention is the use of glucocorticoids, which work broadly to inhibit inflammation by suppressing cell-mediated immunity. Steroids, such as MPSS, cause a downregulation of key proinflammatory mediators such as IL-1 and IL-6. In addition, they decrease production of T cells and decrease the lysosomal activity of neutrophils and monocytes.
Steroids have been shown to positively affect SCI through a variety of mechanisms. Ducker and Hamit (4) first reported in 1969 that reducing local edema, either by use of glucocorticoids or local hypothermia, could reduce further insult to spinal cord tissue by interrupting the “selfdestructive pathophysiologic response” and facilitate functional recovery. Black and Markowitz later demonstrated similar effects of steroids (dexamethasone) and local hypothermia in monkeys. Only treatment with steroids, however, reached statistical significance (5). Later studies demonstrated that glucocorticoids act more specifically and can reverse intracellular accumulation of calcium and loss of potassium, with the understanding that both ions play a critical role in neuron and axon function (6). Lewin et al. (7) demonstrated that cats treated with dexamethasone, both before and after an induced SCI, showed a statistically significant decrease in potassium loss from spinal cord tissue compared to untreated animals. This effect was observed without a noticeable change in edema of the surrounding tissue. They also demonstrated a high correlation between animal function and potassium content of the cord tissue. Young and Flamm (8) subsequently demonstrated that MPSS facilitated calcium ion recovery by contused spinal cord tissue and help prevent loss of evoked potentials in these animals. Cats treated with highdose (30 mg/kg) MPSS after an induced SCI had similar losses of calcium immediately after injury compared to controls and were no different in their recovery of calcium ions in the first 2 hours. However, in contrast to untreated cats, animals treated with high-dose MPSS continued to recover Ca2+ in the next 2 to 3 hours reaching a steady level by 4 hours after injury.
Young and Flamm (8) were also able to show that steroid administration has a profound effect on perfusion of spinal cord tissue. Animals treated with high-dose MPSS had higher cord blood flow than controls 1 to 4 hours
after injury and had higher blood flow compared to preinjury levels in the first hour after SCI. Anderson et al. (9) and Hall et al. (10), showing similar effects on microvascular perfusion and spinal cord blood flow, respectively, have further supported this mechanism of steroids on spinal cord tissue. However, these studies administered MPSS 1 to 1.5 hours after SCI. Furthermore, a variety of doses were used, including different study groups of 15, 30,and 60 mg/kg; it appears from both studies that a dose around 30 mg/kg is required in order to realize the reported effects.
after injury and had higher blood flow compared to preinjury levels in the first hour after SCI. Anderson et al. (9) and Hall et al. (10), showing similar effects on microvascular perfusion and spinal cord blood flow, respectively, have further supported this mechanism of steroids on spinal cord tissue. However, these studies administered MPSS 1 to 1.5 hours after SCI. Furthermore, a variety of doses were used, including different study groups of 15, 30,and 60 mg/kg; it appears from both studies that a dose around 30 mg/kg is required in order to realize the reported effects.
Hall has also published on other important mechanisms of glucocorticoids such as the inhibition of prostaglandin E2 and thromboxane A2 (6,11). MPSS decreases membrane lipid hydrolysis, thus decreasing the release of these factors. Along with Braughler and Hall (12), they reported the enhancement of sodium/potassium-ATPase activity by preventing its destruction, thus maintaining neuronal resting membrane potentials. More importantly, however, was their discussion of free-radical formation and resultant lipid peroxidation. Neuronal tissue holds a much higher content of membrane lipid (40%) compared to nonneuronal tissue (5% to 10%) adding to its sensitivity to trauma. After spinal cord tissue has been injured, increased unsaturated free radicals are formed from free fatty acids of neuronal membranes and myelin. Decreased oxygen delivery to spinal cord tissue also promotes dissociated electron transport chain components, resulting in the production additional oxygen free radicals. Accumulation of these free radicals results in formation of lipid peroxidases that ultimately continue a self-destructive process of the spinal cord tissue. Hall and Braughler (13) were able to show in their work on cat models that treatment with MPSS (with either a 30 or 60 mg/kg dose) reduced the level of lipid peroxidation of spinal cord tissue.
CLINICAL STUDIES AND NASCIS I, II, AND III
Nearly a decade of research on SCI animal models, demonstrating a variety of beneficial mechanisms, supported the large clinical studies on MPSS in the setting of acute SCI. This medication became the focus of clinical studies due to its properties of passing more easily through cell membranes and its superior antioxidant properties, discussed above. Three large randomized series, the National Acute SCI Studies (NASCIS) I, II, and III, investigated the efficacy of MPSS and other agents in treating SCI.
NASCIS I, published in 1984, set out to compare highdose versus low-dose MPSS in patients with SCI (1). This was a double-blind, randomized trial with 330 patients with two treatment groups, one treated with 1,000 mg IV bolus dose followed by 250 mg every 6 hours for 10 days and the other treated with 100 mg IV bolus followed by 25 mg every 6 hours for 10 days. Follow-up data were reported at 6 weeks and 6 months. Placebo control was not included as it was thought that denying a patient a presumed benefit with steroids did not meet ethical standards.
Results of NASCIS I showed that high-dose steroid therapy after SCI with prolonged treatment resulted in greater complications, including death and infection. Though not statistically significant, death occurred in 12.5% of those receiving high-dose MPSS, compared to 8.4% receiving low-dose MPSS. In contrast, there was a significantly greater chance of wound infections on high-dose MPSS (RR 3.6, p = 0.01). There was not statistically significant difference between the high doses and standard-dose protocols with respect to neurologic recovery. This study brought into question the use of steroids after SCI and illustrated the risks of high-dose therapy without any benefit compared to low-dose (standard) therapy. The results conflict with the results of animal studies, which may be accounted for by the mechanism producing SCI in such studies. Most animal models produced SCI by direct impact on the spinal cord, whereas in human SCI, factors of rotation and translation are involved. Toward the end of the study, animal data were reported that demonstrated a theoretical threshold of 15 to 30 mg/kg of MPSS to detect a clinical benefit; the doses administered in this study only reached 1.43 and 14.3 mg/kg.
Additionally, the study had a large dropout rate, that is, 22% at 6 weeks (only 9.5% of these had died) and 46% at 6 months (only 11.5% had died). While the dropout of patients reportedly was equal between the two treatment groups, the large number of patients unavailable for follow-up is a problematic aspect of this report. Finally, the authors’ discussion of their results acknowledges the need for a placebo-controlled trial to truly investigate the efficacy of MPSS after SCI.
A follow-up study to this first report, NASCIS II, has been the central paper around which many current practices and controversies surround (2,14). This multicenter, double-blind, placebo-controlled trial included 487 patients with varying SCI severity, investigating the efficacy of MPSS versus naloxone versus placebo. All patients
presented and were diagnosed within 12 hours of their injury. MPSS was given to 162 patients as a 30 mg/kg bolus and continued at 5.4 mg/kg/h for the following 23 hours, naloxone was administered to 154 patients as a 5.4 mg/kg bolus followed by 4.0 mg/kg/h for the next 23 hours, and 171 patients were given placebo. Sixty percent of those randomized had complete injuries, and the average time from injury to bolus dose in all patients was 3 hours (Table 132.1).
presented and were diagnosed within 12 hours of their injury. MPSS was given to 162 patients as a 30 mg/kg bolus and continued at 5.4 mg/kg/h for the following 23 hours, naloxone was administered to 154 patients as a 5.4 mg/kg bolus followed by 4.0 mg/kg/h for the next 23 hours, and 171 patients were given placebo. Sixty percent of those randomized had complete injuries, and the average time from injury to bolus dose in all patients was 3 hours (Table 132.1).
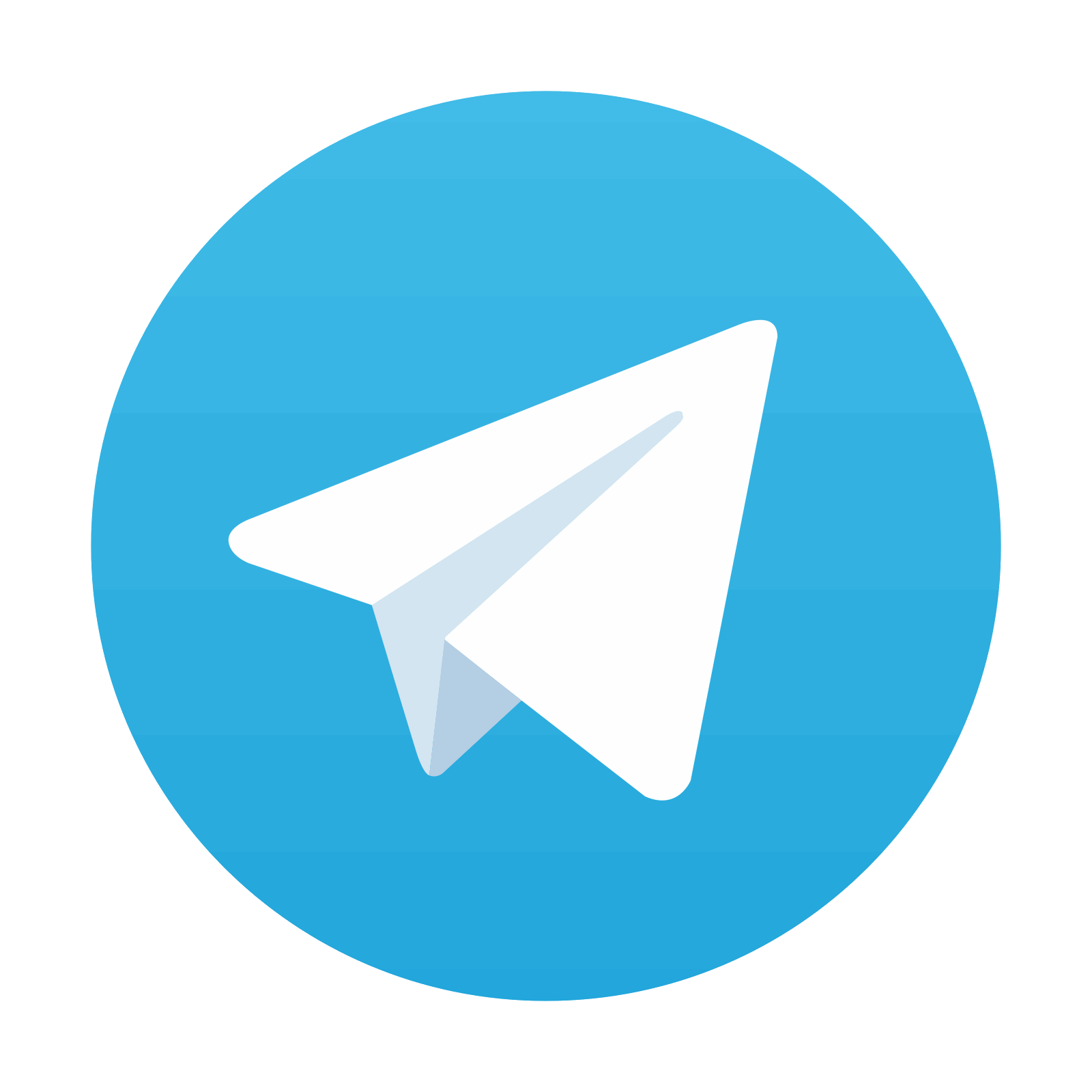
Stay updated, free articles. Join our Telegram channel

Full access? Get Clinical Tree
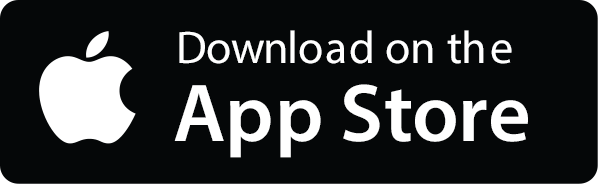
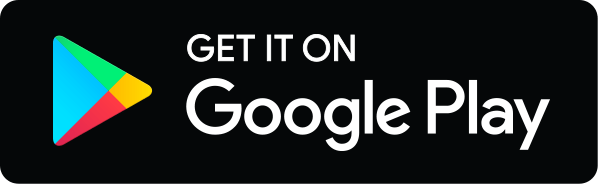