(1)
Department of Behavioral Neurobiology, Institute of Experimental Medicine, Budapest, Hungary
Abstract
Defined in the simplest way, aggression is a behavior that aims at inflicting harm. Inflicting harm, however, is not a simple mechanical act. The target moves, works against offensive actions, and may even counterattack. If aggression consisted exclusively of simple, clearly definable behavioral acts (e.g., biting in animals and hitting in people), its neural control would be similarly simple and would have been discovered a long time ago. However, aggression comprises a series of widely different actions, some of which aim at social recognition, others at avoiding harm, yet others at inflicting harm or expressing dominance, and all these need to be coordinated and adapted to environmental conditions (for instance, the actions of opponents) and temporal changes in these. Based on the findings reviewed in previous chapters, here we evaluate the neural substrates of cognitive and emotional processes that affect aggressiveness as well as the neuronal circuits involved in execution of aggressive acts. The integration of the two levels of aggression control as well as functional alterations of the system is investigated with respect to the emergence of abnormal forms of aggression.
We do not intend in this chapter to reiterate the details (and the references to these details) that were amply presented in previous ones. Here, we attempt at providing a synthesis of the finding in broad terms. For most of the supporting evidence, the readers are kindly directed to the earlier chapters.
6.1 Who’s Flying the Plane and What Kind of Plane?
The first metaphor in this title was borrowed from Siegel and Douard (2011) who addressed a philosophical problem raised by neurochemical models of violence. They asked the question whether aggression is biologically deterministic, in which case perpetrators are not responsible for their acts, or it depends on free will, in which case perpetrators are culpable. In our adaptation of the metaphor, both the paraphrased actors and the question are different. The ones who fly the plane are brain areas, while the plane itself is aggression. The question “who’s flying the plane” may be reformulated less metaphorically: which brain area controls aggression, to what extent, and in which ways? Which of these brain areas or which of their functions go wrong when subjects show abnormal forms of aggression?
The second metaphoric question refers to the nature of aggression. Defined in the simplest way, aggression is a behavior that aims at inflicting harm. Inflicting harm, however, is not a simple mechanical act. The target moves, works against offensive actions, and may even counterattack. If aggression consisted exclusively of simple, clearly definable behavioral acts (e.g., biting in animals and hitting in people), its neural control would be similarly simple and would have been discovered a long time ago. However, aggression comprises a series of widely different actions, some of which aim at social recognition, others at avoiding harm, yet others at inflicting harm or expressing dominance, and all these need to be coordinated and adapted to environmental conditions (for instance, the actions of opponents) and temporal changes in these. Metaphorically speaking, the “plane of aggression” is a rather complex one.
The first section of this chapter is an attempt to corroborate the types of behavioral acts performed during aggression and the types of functions and abilities that particular brain areas possess. Alterations in the system and their possible repercussions for aggressive behavior are discussed in the following chapter.
6.2 Behavior and Brain Function in Animal Aggression
6.2.1 Normal Aggression
In this section we propose that the execution of aggressive acts is initiated by higher-order brain areas, primarily by the prefrontal cortex. This hypothesis challenges the view that aggression is released from, and governed by, mechanisms located in lower-order brain areas (e.g., the hypothalamus and periaqueductal gray) when their inhibition by higher brain structures is suspended. We argue here that the latter two brain areas may indeed put into action neuronal networks that control the execution of fixed action patterns (e.g., the movement sequences of attacks), but they are unable and inappropriate to control aggressive behavior in the context of an ongoing conflict, simply because they have no information on what happens “in the field.” The choice and timing of behaviors is more likely controlled by higher-order brain structures where the necessary information converges. The arguments supporting the model are as follows.
1.
Many of the behavioral acts performed by rodents during aggressive encounters have a fixed choreography, which is innate.1 For example, clinch fights in rats are composed by a sequence of movements which starts with bending over the opponent while the animals are positioned in a right angle relative to each other; continues with snatching with teeth the flank of the victim on the side opposite to the attacker, pulling the victim in the air with teeth; and ends by a blow with hind paws that throw the victim away.2 This behavioral sequence does not depend on social experience; e.g., it is displayed in a highly similar fashion by rats reared in isolation from weaning. Some of the behavioral acts performed during aggressive encounters are composed of simpler movement sequences, but are clearly recognizable as fixed action patterns. This particularity of rodent and more generally animal behavior made the description of ethograms possible.3 Under particular conditions, a fixed action pattern may be present or absent, may be expressed frequently or sporadically, but the basic movement sequences are not altered. If a clinch attack is present, it is always performed in the same way.
2.
It is reasonable to assume that innate and fixed action patterns are controlled by specialized neural networks. The whole sequence described above takes less than a second which leaves no room for planning and cognitive control. Given that clinch attacks seem to be innate and fixed action patterns that are executed extremely quickly, we assume that they are evoked by successive discharges of a tightly interconnected set of neuronal structures. Furthermore, it is reasonable to assume that the network is activated by brain areas from where the movement sequence can be evoked by electrical stimulation. For instance, the stimulation of the mediobasal hypothalamus elicits the whole sequence described above.4
While the hypothalamus is part of the network that evokes clinch fights, it is unlikely that it is able alone and solely to control such complex sequences that include movements of the limbs, body, head, and jaws. It is more likely that the hypothalamic attack area plays an initiating role, while the movements of the sequence are governed by lower brain areas and the spinal cord. This neuronal network likely includes offense-related mechanisms located within the PAG, as particular neurons in this brain area innervate centers, which elicit jaw and limb movements (see Sect. 4.4).
The PAG appears to be important also for another category of behaviors frequently shown during aggressive encounters. Any of the contestants including the intruder may prevail in particular moments of the contest, which makes defense, flight, or immobility necessary for the adversary. These forms of defensive behavior can be elicited by the electrical stimulation of the “defensive” PAG (subregions governing defensive behaviors; see Sect. 4.4); these behaviors appear to be controlled by this brain area in a manner similar to the control of attacks by the hypothalamus.
3.
Aggressive encounters consist of intercalated bouts of offense and defense (moreover social investigation and exploration), the expression and succession of which is determined by the momentary needs and constraints of social interaction rather than by predetermined programs. The mediobasal hypothalamus and the PAG may trigger fixed action patterns, but it is highly unlikely that these brain areas are able to adapt the execution of behaviors to the circumstances. The reason is that they do not receive the information necessary to make decisions on what to do and when. Decisions of this type must come from upstream, from brain areas where environmental information converges and is processed. In addition, the choice of behaviors and their timing needs information on the internal state of the organism and on past experiences and is also governed by emotions. Such information also seems to converge to, and to be processed by, higher-order brain areas. Consequently, decisions regarding the expression and succession of particular action patterns must be taken at levels higher than the mediobasal hypothalamus and the PAG. The most likely brain site of such decisions is the prefrontal cortex. The amygdala may also have a role, but according to current knowledge, the main brain site of decisions is the prefrontal cortex.
4.
At the level of this brain area, the processing of information is achieved by communication with lower brain centers (e.g., sensory fields, thalamus, amygdala) and interactions between its subareas. The net result of this intricate network of information exchange seems to result in decisions that are communicated downwards by those prefrontal neurons that project directly to the executive mechanisms contained by lower-order brain areas. Thus, the organizing role of the prefrontal cortex seems to be performed in two steps. The first step includes the processing of information and decision making which involves extended regions of these brain areas. The second step is the communication of decision via a small number of dedicated neurons.
In brief, we propose that behavior in aggressive conflicts is shaped by a cascade of neuronal events, which start by information processing and decision making at the level of the prefrontal cortex (Fig. 6.1). Decisions are communicated to lower brain areas by subgroups of neurons located within the very same brain area. On their turn, lower-order brain areas initiate the activity of specialized neuronal networks that elicit coordinated muscle contractions that sum up as goal-directed behaviors. The whole network seems to oscillate between different modes of function, as episodes of offense (threats and attacks), episodes of defense (defensiveness, flight, immobility), and episodes of “relaxation” (exploration, grooming, social investigation) alternate frequently. Alternation is determined partly by external circumstances (actions by opponents which trigger shifts from offense to defense and back) partly by internal circumstances (for instance, by fatigue which triggers shifts from agonistic behaviors to “relaxation”). These circumstances are continuously monitored by higher-order brain centers, which switch executive mechanisms on and off depending on the situation.
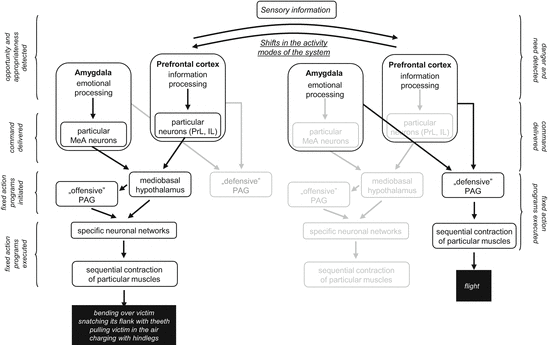
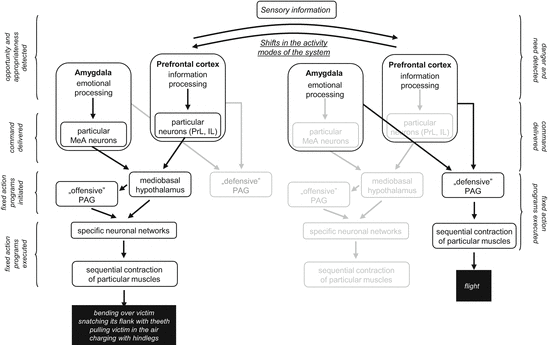
Fig. 6.1
Cascade of neural events leading to clinch attack (left) and flight (right) during one and the same resident/intruder conflict in rats. Note that periods of offense and defense alternate in both contestants, even if their ratio depends on status. The basic idea is that the choice between alternative behaviors and their timing can be established by higher order brain areas (PFC and amygdala) where the neccesary information converge. The mediobasal hypothalamus and PAG are responsible only for “mechanical” execution and organization (see Sect. 6.1). Black area names and arrows, mechanisms momentarily active; grey area names and arrows, mechanisms momentarily inactive; italics, neural and behavioral events; “defensive” and “offensive” PAG, periaqueductal gray mechanisms controlling defense and offense, respectively; MeA, PrL, and IL, medial amygdala, prelimbic and infralimbic neurons that project directly to the mediobasal hypothalamus
6.2.2 Abnormal Aggression
Our first study on the neural background of glucocorticoid dysfunction-induced abnormal aggression brought about a great surprise: brain areas believed to play an important role in rivalry aggression (the medial amygdala, mediobasal hypothalamus, and periaqueductal gray) were activated by aggressive encounters, but the level of activation was not different from that seen in controls. Changes were seen in brain areas where these were unexpected, e.g., the central amygdala.5 We experienced a second surprise when other models of abnormal aggression were analyzed with respect to their neural background. Functional brain alterations similar to those observed in the glucocorticoid dysfunction model were seen in a restricted number of models only. Even our second model of abnormal aggression (postweaning social isolation) proved to have different neural underpinnings as evidenced by the c-Fos technology.
The overall conclusion of the studies published so far is that abnormal aggression may result from a variety of brain alterations, which, however, may be grouped into the following three main categories.
1.
Hypoarousal type models are characterized by downregulated emotional responses to aggression in terms of both glucocorticoid and autonomic changes. In these models, the medial and central amygdala and the mediobasal and the lateral hypothalamus are co-activated; as it regards periaqueductal gray, the center of activation seems to shift ventrally. Similar patterns of emotional and neural responses are typically seen in two types of normal aggression, namely predation and maternal aggression.
The similarities between this subgroup of abnormal aggression models on one side and predatory and maternal aggressions on the other side suggest that those etiological factors that result in the aforementioned pattern of emotional and neural particularities (e.g. abnormal forms of aggression) do not bring about some kind of “unnatural” ways of neural and emotional functioning, but activate mechanisms that are inappropriate to the situation.
2.
Hyperarousal type models are associated with increased emotional responses; either or both autonomic and glucocorticoid responses are increased in this type of models. Interestingly, higher emotionality seems to be restricted to social contexts, because subjects show normal or even reduced emotional responses to nonsocial challenges in some models. No brain areas other than those involved in rivalry aggression are activated, but their activation levels are higher than in controls.
The primacy of emotional and neuronal changes remains to be clarified. High emotions may inherently lead to enhanced activation of brain regions that control behavior during conflict, but it is equally possible that changes in brain function explain both increased emotions and aggression. Either alternative may be supported by the gross spatial co-localization of emotion- and aggression-related mechanisms (e.g., the “amygdala” hosts both). This cannot settle the question of primacy because local (within-area) interactions between the two mechanisms are obscure. Based on the observation that amygdala neurons involved in sexual and aggressive behavior are distinct but spatially intermingled, one can assume that emotion- and aggression-bound mechanisms need to be discerned at neuronal level.
3.
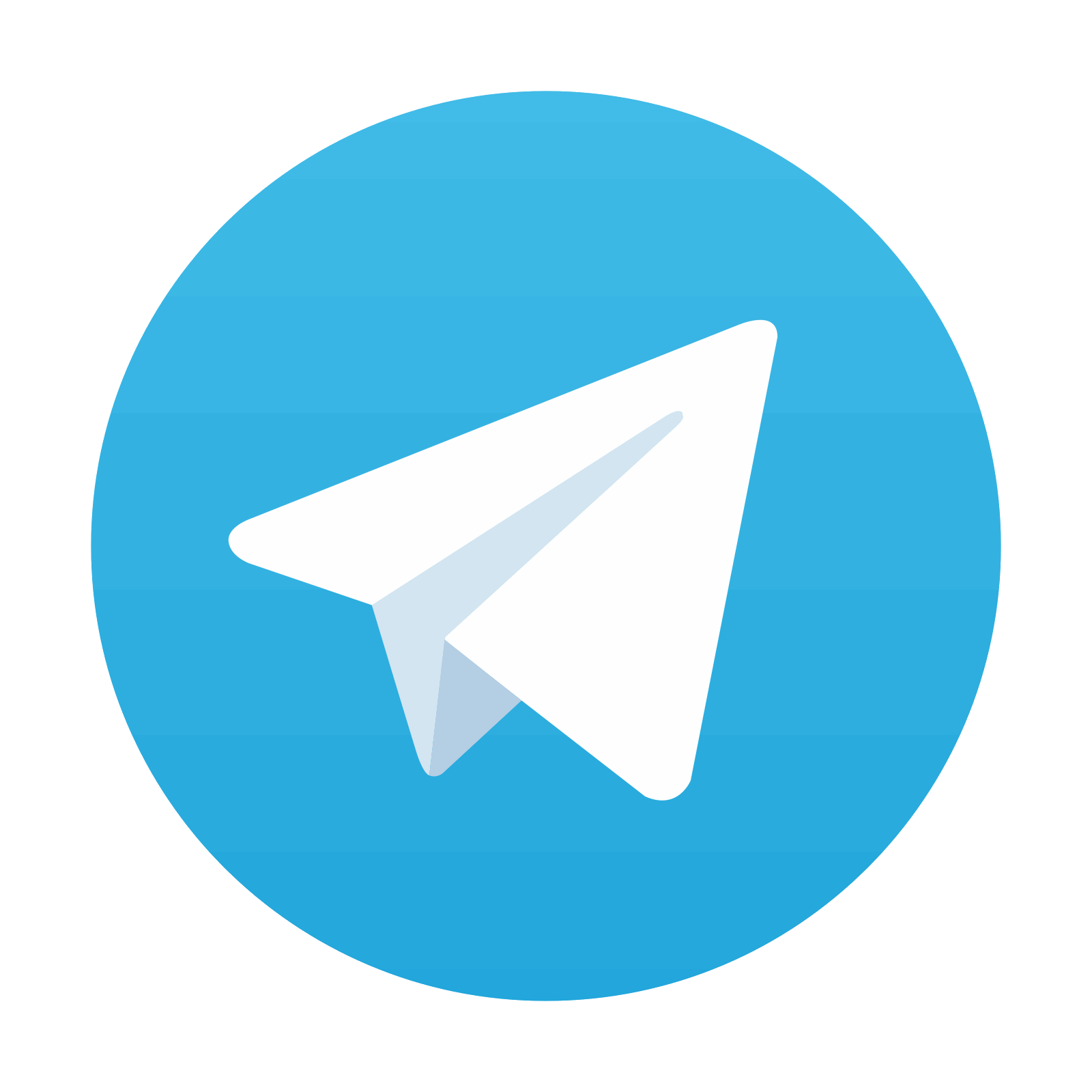
In minor type models, neural and behavioral changes are subtle. The former are usually restricted to neurochemical changes seen in a few brain regions. Some of them were seen in brain areas that were not analyzed in detail here, e.g., the septum. It occurs that vasopressinergic communication is especially affected in these models.
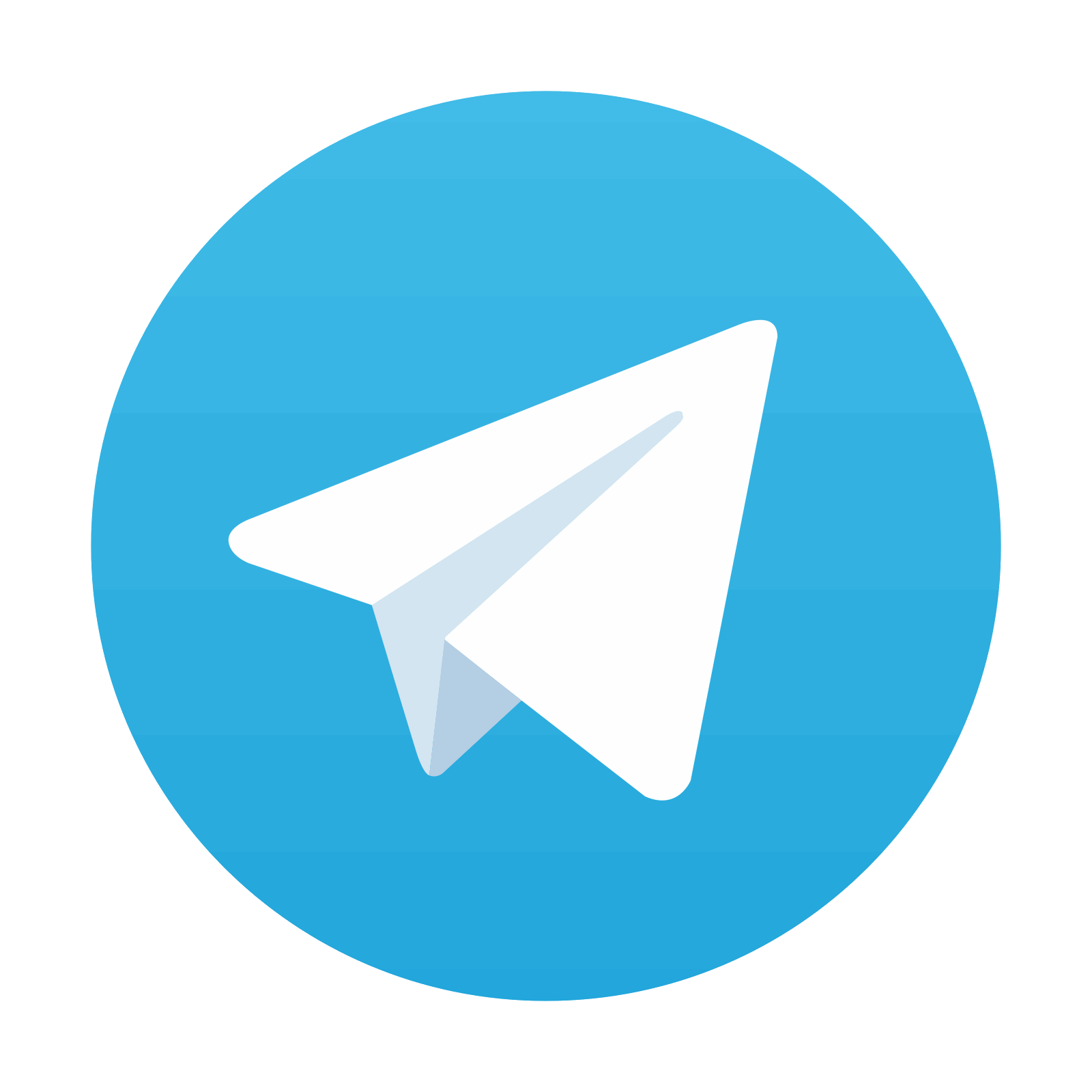
Stay updated, free articles. Join our Telegram channel

Full access? Get Clinical Tree
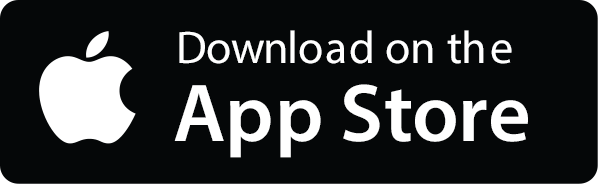
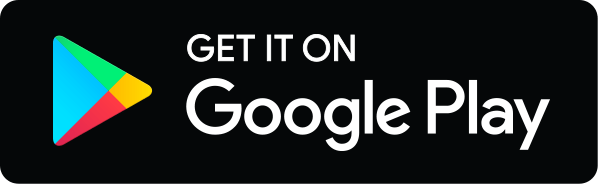
