Aneurysmal subarachnoid hemorrhage (aSAH) is a common and often devastating condition that requires prompt neurosurgical evaluation and intervention. Modern management of aSAH involves a multidisciplinary team of subspecialists, including vascular neurosurgeons, neurocritical care specialists and, frequently, neurointerventional radiologists. This team is responsible for stabilizing the patient on presentation, diagnosing the offending ruptured aneurysm, securing the aneurysm, and managing the patient through a typically prolonged and complicated hospital course. Surgical intervention has remained a definitive treatment for ruptured cerebral aneurysms since the early 1900s. Over the subsequent decades, many innovations in microsurgical technique, adjuvant maneuvers, and intraoperative and perioperative medical therapies have advanced the care of patients with aSAH. This report focuses on the modern surgical management of patients with aSAH. Following a brief historical perspective on the origin of aneurysm surgery, the topics discussed include the timing of surgical intervention after aSAH, commonly used surgical approaches and craniotomies, fenestration of the lamina terminalis, intraoperative neurophysiological monitoring, intraoperative digital subtraction and fluorescent angiography, temporary clipping, deep hypothermic cardiopulmonary bypass, management of acute hydrocephalus, cerebral revascularization, and novel clip configurations and microsurgical techniques. Many of the topics highlighted in this report represent some of the more debated techniques in vascular neurosurgery. The popularity of such techniques is constantly evolving as new studies are performed and data about their utility become available.
Aneurysmal subarachnoid hemorrhage (aSAH) comprises approximately 2% to 5% of all strokes in the United States, affecting about 30,000 people annually, and the worldwide incidence is approximately 10.5 cases per 1000,000 individuals. Despite advances in diagnostic tools, perioperative management, and definitive surgical or endovascular interventions, aSAH remains a devastating condition. Following aSAH, at least 12% of patients die before receiving medical attention, 46% die within 30 days, and many survivors have significant morbidity and require long term assistance. Cognitive dysfunction is common among aSAH survivors, with up to 50% showing deficits and unable to return to work. Because aSAH occurs at a relatively young age and has such a poor prognosis, it is estimated that the loss of productive years from SAH is a significant portion of years lost from ischemic stroke. Outcomes following aSAH are primarily determined by the severity of the initial bleed, early rebleeding, and delayed cerebral ischemia secondary to vasospasm.
Intracranial aneurysm formation and subsequent rupture is a complex multifactorial process that is not well understood. Epidemiology of aSAH is dependent on age, sex, race, and location. Aneurysmal SAH can occur in any age group, but is most common in the fourth to sixth decades. Women have 1.6 times greater risk than men of aSAH and African Americans have 2.1 times the risk of whites. Incidence of aSAH varies greatly among different countries, with Japan (23 to 32 per 100,000) and Finland (22.5 per 100,000) having the highest statistics. Modifiable risk factors include smoking, hypertension, heavy alcohol intake, and use of sympathomimetic agents (eg, cocaine). Nonmodifiable risk factors include a family history of SAH and autosomal-dominant polycystic kidney disease, as well as various uncharacterized genetic susceptibility loci. Familial intracranial aneurysms tend to rupture at earlier ages than sporadic aneurysms.
Aneurysmal SAH is a life-threatening condition that requires prompt medical and surgical attention. This article reviews the surgical management of aSAH, describing frequently used craniotomies and certain additional techniques and surgical maneuvers that are currently debated in the literature.
Historical perspective
Early treatment of cerebral aneurysms involved ligation of the proximal parent artery, a technique named Hunterian ligation after John Hunter (1728–1793), who popularized the technique in peripheral arteries in the mid 1700s. Victor Horsley (1857–1916) was the first person to apply this technique to the cerebral circulation when he performed internal carotid artery (ICA) ligation for a giant intracranial aneurysm in 1885. Norman Dott (1897–1973) subsequently performed the first planned intracranial surgery for treatment of a ruptured cerebral aneurysm in 1931, in which he wrapped the aneurysm with muscle for hemostasis. Dott learned the technique of using muscle pledgets while training under Harvey Cushing (1869–1939), who is thought to be the first surgeon to pack and wrap an unruptured intracranial aneurysm. Dott also pioneered the technique of aneurysm suture ligation in 1933, although this technically challenging maneuver was eventually supplanted by aneurysm clips.
Cushing introduced hemostatic silver vessel clips to neurosurgery in 1911, first using these clips in tumor surgeries to achieve hemostasis on vessels not accessible to suture ligation. Walter Dandy (1886–1946) used a modified Cushing V-shaped silver clip in 1937 to perform the first clipping of an ICA aneurysm. Over the subsequent decades, aneurysm clips underwent many design modifications, particularly with respect to the size and shape of clips suitable for microsurgery and to the materials required for compatibility with magnetic resonance imaging (eg, titanium). In the late 1970s, clipping of ruptured aneurysms was shown to be better than the alternatives of bed rest and carotid artery ligation, and neurosurgical practices shifted toward using clips for routine treatment.
Timing of surgical intervention
Practice regarding the timing of surgery following aneurysm rupture has been historically controversial and has gone through changes over the years. From the 1950s to the mid 1970s, most surgeons advocated delaying surgical intervention for aneurysm clipping at least 1 week until the patient was medically stable. Early surgery was presumed to be more technically demanding due to brain swelling, thought to worsen vasospasm, and was associated with high operative morbidity and worse outcomes. While delayed surgical intervention led to excellent operative results, overall patient outcomes remained poor because of high rates of rebleeding and significant vasospasm-related morbidity and mortality in patients waiting for surgery. Rebleeding is a major concern following aSAH, as mortality from such an event reaches 70%. The risk of rebleeding following aSAH without intervention is up to 40% within 30 days, with the rate greatest within the first 24 hours (4%) versus a daily rate of 1% to 2% for the subsequent 4 weeks. Certain studies have demonstrated higher rebleed rates (15%) within the first day, especially within 2 to 12 hours. Following the 30-day peak period, the risk of rebleed settles out at about 3% per year.
Interest in early surgery increased in the late 1970s as surgical techniques improved, operations became safer, and as medical management failed to significantly reduce rates of vasospasm and rebleeding before surgery. Following favorable initial results from early surgery, primarily from Japanese groups, the International Cooperative Study on the Timing of Aneurysm Surgery sought to better characterize the relationship between outcomes for early (0–3 days) versus late (11–14 days) surgery after aSAH. This study showed that the overall patient outcome of early surgery was equivalent to that of late surgery. Of note, patients in the late surgery group had better surgical outcomes at 6 months than the early surgery group, most likely secondary to natural selection of survivors. However, up to 30% of patients with aSAH do not survive to have surgery at the later time points, and waiting 2 weeks for surgery was associated with a 30% risk of focal ischemic deficit and 12% risk of rebleeding. Thus, the additional risks associated with delayed surgery negated the better surgical outcomes seen in this group, and early surgery became a feasible option. Additional impetus for early surgery is that these patients, having a secured aneurysm, would greatly benefit from any future advances in vasospasm management. It is now common practice that patients are operated on within 48 hrs of presentation if an aneurysm is present.
Timing of surgical intervention
Practice regarding the timing of surgery following aneurysm rupture has been historically controversial and has gone through changes over the years. From the 1950s to the mid 1970s, most surgeons advocated delaying surgical intervention for aneurysm clipping at least 1 week until the patient was medically stable. Early surgery was presumed to be more technically demanding due to brain swelling, thought to worsen vasospasm, and was associated with high operative morbidity and worse outcomes. While delayed surgical intervention led to excellent operative results, overall patient outcomes remained poor because of high rates of rebleeding and significant vasospasm-related morbidity and mortality in patients waiting for surgery. Rebleeding is a major concern following aSAH, as mortality from such an event reaches 70%. The risk of rebleeding following aSAH without intervention is up to 40% within 30 days, with the rate greatest within the first 24 hours (4%) versus a daily rate of 1% to 2% for the subsequent 4 weeks. Certain studies have demonstrated higher rebleed rates (15%) within the first day, especially within 2 to 12 hours. Following the 30-day peak period, the risk of rebleed settles out at about 3% per year.
Interest in early surgery increased in the late 1970s as surgical techniques improved, operations became safer, and as medical management failed to significantly reduce rates of vasospasm and rebleeding before surgery. Following favorable initial results from early surgery, primarily from Japanese groups, the International Cooperative Study on the Timing of Aneurysm Surgery sought to better characterize the relationship between outcomes for early (0–3 days) versus late (11–14 days) surgery after aSAH. This study showed that the overall patient outcome of early surgery was equivalent to that of late surgery. Of note, patients in the late surgery group had better surgical outcomes at 6 months than the early surgery group, most likely secondary to natural selection of survivors. However, up to 30% of patients with aSAH do not survive to have surgery at the later time points, and waiting 2 weeks for surgery was associated with a 30% risk of focal ischemic deficit and 12% risk of rebleeding. Thus, the additional risks associated with delayed surgery negated the better surgical outcomes seen in this group, and early surgery became a feasible option. Additional impetus for early surgery is that these patients, having a secured aneurysm, would greatly benefit from any future advances in vasospasm management. It is now common practice that patients are operated on within 48 hrs of presentation if an aneurysm is present.
Common surgical approaches
Surgery for intracranial aneurysms relies on 2 main principles: gaining access to the aneurysm through a craniotomy and subsequently securing the aneurysm. The basic principles of turning a craniotomy and the microsurgical principles used for the management of acute aSAH (eg, opening of the arachnoid, drainage of cerebral cisterns, use of microinstruments, use of the operating microscope, and brain retraction) have been thoroughly reviewed in numerous articles and textbooks. This section reviews common craniotomies used in most operative cases for acute aSAH. The surgical approach to any given aneurysm depends on a variety of factors, including aneurysm location, morphology, orientation, and neck anatomy. There are 3 main surgical approaches, with possible extensions, required to treat the majority of anterior and posterior circulation aneurysms. Each of these approaches is described briefly below.
Frontosphenotemporal (Pterional) Craniotomy, and the Orbitozygomatic and Subtemporal Extensions
The frontosphenotemporal or pterional craniotomy ( Fig. 1 ) is the workhorse of vascular neurosurgery and can be used for most anterior circulation aneurysms and upper posterior circulation aneurysms. For this approach, the head is placed in a radiolucent skull immobilizer, rotated (up to 60 degrees depending on surgical target), and the neck is extended (approximately 30 degrees) so that the malar eminence is the highest point. The scalp is incised from the root of the zygomatic arch, to the linea temporalis, and anteriorly to the midline just short of the patient’s hairline. The scalp and temporalis muscles are elevated using a subfascial dissection to preserve the frontalis branch of the facial nerve.
In the authors’ practice, the craniotomy is performed using 5 burr holes: keyhole, above the zygomatic root, approximately 1 cm above the temporal squamosa (in line with zygomatic root), intersection of coronal suture with linea temporalis, and frontal bone above frontal sinus and orbit. The burr holes are connected with a Gigli saw (allows for maximum beveling and superior aesthetic results) except for the segment between the keyhole and zygomatic root, which is drilled. Additional squamosal temporal bone is removed with a rongeur to expose the floor of the middle cranial fossa. The greater and lesser wings of the sphenoid are then drilled until the dural flap covering the orbitomeningeal artery is exposed. The dura is opened with a semicircular incision and reflected anterior.
To improve access to the basilar apex and upper clivus regions, the orbitozygomatic and subtemporal extensions (see Fig. 1 ) can be performed following a traditional pterional craniotomy. Since Jane and colleagues first described the supraorbital craniotomy, it has evolved considerably through many adjustments. The traditional orbitozygomatic craniotomy as described by Zabramski and colleagues and the modified orbitozygomatic craniotomies as described by Lemole and colleagues are commonly used today. For the traditional orbitozygomatic craniotomy, the temporal fascia is elevated to expose the zygoma and superior orbital rim, and the periorbita is detached from the orbital roof with the use of an Adson elevator. The orbital and zygomatic osteotomies are then performed using a series of 6 bone cuts involving the orbital roof, lateral orbit, maxillary root, and zygomatic root as previously described. Subtemporal and supraorbital modifications of the traditional orbitozygomatic craniotomy can be performed to better tailor the craniotomy to treat lesions in the temporal fossa or the anterior/middle cranial fossae, respectively. In general, the authors favor the modified orbitozygomatic craniotomy over the full version of this extension.
The subtemporal craniotomy was popularized by Charles Drake in 1961 as an approach to the basilar apex. In brief, the subtemporal approach is performed through a horseshoe-shaped incision starting at the zygoma in front of the ear, extending superiorly and posteriorly along the linea temporalis, and then inferior behind the mastoid. The craniotomy flap is turned using burr holes at the corners of the intended boney exposure and by drilling a trough across the base of the bone flap. Once the bone flap is removed, the inferior edge of the craniotomy is drilled flush with the floor of the middle cranial fossa, and a horseshoe-shaped dural flap is reflected inferiorly. The limitations of this approach include a narrow surgical corridor, the need for significant brain retraction, and difficult access to the contralateral P1 segment and nearby perforators. In the setting of aSAH, a swollen temporal lobe can be problematic when this approach is used.
Most neurosurgeons today use the subtemporal craniotomy as an extension of the frontosphenotemporal craniotomy. The “half and half” approach, originally mentioned by Drake in 1978 and popularized by Batjer and Samson, combines the pterional craniotomy with a subtemporal craniotomy. This combined approach essentially eliminates the disadvantages of a pure subtemporal approach, and it provides good access to the basilar bifurcation, the superior cerebellar artery takeoff, and the P1 segment.
As with all surgical procedures, cosmetically superior reconstruction following craniotomy for aSAH is paramount to prevent disfigurement and negative psychosocial effects on the patient and the family. Patients undergoing pterional craniotomies for aneurysm clipping commonly have depression of the frontozygomatic fossa 6 to 12 months after surgery secondary to atrophy of the temporalis muscle. Such cosmetic defects can be avoided by careful dissection to maintain the neurovascular supply to the temporalis muscle and by a simple use of a frontozygomatic fossa titanium cranioplasty. Raza and colleagues described the use of a frontozygomatic titanium cranioplasty in 194 patients who underwent a pterional craniotomy with average follow-up of 9.5 months. In this series, 93% of patients had excellent cosmetic outcomes with virtually no evidence of surgery, and the remaining 7% had only slight depression of the temporalis fossa. This method and other such techniques should be used when possible to achieve outstanding cosmesis in aSAH patients.
Anterior Parasagittal Craniotomy
The anterior parasagittal craniotomy ( Fig. 2 ) is used for interhemispheric approaches to distal anterior cerebral artery aneurysms as described by Tamargo and colleagues. For this approach, a radiolucent skull immobilizer is placed and then the head is distracted, flexed, and rotated to the contralateral side. Many variations in positioning have been described, with some surgeons preferring more lateral head position to facilitate gravity retraction and increased exposure of the interhemispheric fissure.
The scalp is incised in a bicoronal fashion and the flap is reflected anteriorly. Following identification of the coronal and sagittal sutures, a pentagonal craniotomy is planned with 5 burr holes so that the craniotomy straddles the midline and extends 4 to 5 cm in front and 2 to 3 cm behind the coronal suture. The anterior-posterior position of the craniotomy in relation to the coronal suture can be modified depending on the location of the aneurysm. Two burr holes are placed ipsilateral to the lesion, 2 burr holes directly over the sagittal sinus, and a single burr hole is placed on the contralateral side. The burr holes are connected with a Gigli saw. A semicircular incision is made for the dural flap with its base along the sagittal sinus.
Lateral Suboccipital Craniectomy and the Far-Lateral Transcondylar Extension
Although there are many different described approaches to aneurysms of the posterior circulation, most aneurysms of the vertebral trunk, the mid and lower basilar trunk, and their associated branches (superior cerebellar artery, anterior inferior cerebellar artery, and posterior inferior cerebellar artery) can be approached by a lateral suboccipital craniectomy with or without the far-lateral extension as described ( Fig. 3 ).
For the lateral suboccipital craniectomy, the patient is placed in a skull clamp and is positioned in either the park-bench or lateral position. The incision starts 3 cm behind the posterior margin of the pinna and extends in a sigmoid fashion to the spinous process of C2. After reflection of the suboccipital musculature, the asterion is identified (landmark for junction of transverse and sigmoid sinuses). A craniectomy is then performed that extends from the asterion (supralateral margin), to just above the foramen magnum (inferior), and medially to expose the lateral cerebellum. The dura is opened in a lambdoid incision with respect to the sigmoid and transverse sinuses.
For the far-lateral extension, a “hockey stick” incision is performed with the lateral arm beginning superior and posterior to the ear, descending to the superior nuchal line, crossing medial to the midline, and then descending to the spinous process of C2 or C3. A musculocutaneous flap is then elevated to expose the foramen magnum, the mastoid, and C1 from the arch to the transverse process. The vertebral artery is identified at the point where it enters the dura and then traced to the sulcus arteriosus. The main additions of the far-lateral are removal of the C1 arch, the lip of the foramen magnum, and the posteromedial third of the atlanto-occipital joint. Following completion of the craniectomy, the dura is opened from the transverse sigmoid junction to the arch of C1 and reflected laterally.
Fenestration of the lamina terminalis
Aneurysmal SAH can cause fibrosis of the arachnoid granulations and leptomeninges, leading to altered cerebrospinal fluid (CSF) dynamics and persistent hydrocephalus that requires CSF diversion. Shunt-dependent hydrocephalus occurs in > 20% of patients with aSAH, representing a significant complication. Microsurgical fenestration of the lamina terminalis during aneurysm surgery was proposed in the mid to late 1990s as a means of facilitating CSF dynamics and reducing the incidence of shunt-dependent hydrocephalus in patients with aSAH ; however, subsequent studies have been inconclusive regarding the benefit of this technique. Komotar and colleagues, in a retrospective study of 582 patients with aSAH, demonstrated greater than 80% reduction in shunt-dependent hydrocephalus if the lamina terminalis was fenestrated at the time of surgery. However, a more recent retrospective analysis of 369 patients and a literature review comparing results from 11 different studies failed to find a significant association between lamina terminalis fenestration and decreased shunt dependency. This latest review, in particular, is limited by unmatched cohorts, and all studies of this technique are limited by lack of prospective, randomized data. Pending more definitive studies, this technique is generally favorable and continues to be used by the senior author of this article.
Intraoperative electrophysiological monitoring
Intraoperative neurophysiological monitoring during intracranial aneurysm surgery has become standard practice at the authors’ institution as well as other major medical centers. Intraoperative neurophysiological monitoring is an important adjunct to meticulous surgical inspection and intraoperative angiography to detect cerebral ischemia from temporary clipping, unintentional parent vessel or perforator occlusion, brain manipulation, and retraction injury. Somatosensory evoked potentials (SSEPs), particularly median and posterior tibial nerve SSEPs, are commonly monitored during anterior circulation procedures, whereas dual monitoring with SSEPs and brainstem auditory evoked responses (BAERs) are preferred for posterior circulation aneurysm surgeries.
The rationale for employing electrophysiological monitoring during aneurysm surgery is the significant correlation between alterations in electrical signals and regional cerebral blood flow (rCBF), with transient electrophysiological changes generally corresponding to good outcomes and permanent changes corresponding to postoperative deficits. However, SSEP false-negative rates can reach up to 25% in some studies, and patients with unchanged SSEPs can still have new postoperative motor and other neurologic deficits. Motor deficits with the false-negative SSEP results are commonly attributed to subcortical (internal capsule or brainstem) strokes. Monitoring of motor evoked potentials (MEPs) has been evaluated for efficacy in detecting impending motor deficits. Studies by Neuloh and Schramm, using transcranial electrical stimulation, and Horiuchi and colleagues, using direct cortical stimulation, have demonstrated that MEP deterioration is a more sensitive and reliable predictor of postoperative motor paresis than SSEPs. This technique is promising, but further evaluation in a controlled trial is needed to assess if monitoring with MEPs can reduce morbidity from aneurysm surgery in patients with aSAH.
Electroencephalography (EEG) is also commonly used during surgery for intracranial aneurysm clipping. Prior to temporary clip application, the neuroanesthesia team titrates the brain-protective anesthetic regimen to achieve burst suppression on EEG. Burst suppression helps to decrease metabolic demand so that the cerebral tissue can better tolerate induced ischemia, such as during temporary clipping. EEG is less commonly used to detect ischemia during such surgeries because the airspaces between the dura and arachnoid as a result of the craniotomy and brain relaxation can interfere with scalp EEG recordings. Intraoperative multilobar EEG using subdural electrodes are more sensitive than scalp EEG for detecting ischemic events during aneurysm surgery, but these are not currently in widespread use.
Digital subtraction intraoperative angiography
Egas Moniz (1874–1955) developed cerebral angiography in 1927, but it was not until 1933 when the first angiogram to demonstrate an intracranial aneurysm was performed by Norman Dott. The technology to perform intraoperative angiography was available by the 1960s, however, its routine use did not occur until the 1990s. The interest for intraoperative evaluation of aneurysm clip placement stemmed from reports of routine postoperative angiography that demonstrated unexpected rates of residual aneurysms and major vessel compromise. In such studies, the incidence of residual aneurysms and incidence of parent or branch vessel occlusion were as high as 12% and 19%, respectively, for a possible combined 31% total incidence of unexpected findings. Incompletely treated aneurysms are dangerous as they are prone to regrowth with a 20% to 80% risk of rehemorrhage and 10% to 30% risk of mass effect over 10 to 20 years. These reports, combined with improvements in portable digital subtraction (DS) angiography equipment and practitioner expertise led to more widespread use of intraoperative angiography and subsequent studies evaluating its efficacy.
Various groups have evaluated and demonstrated the efficacy of intraoperative angiography during surgery for aneurysm clipping as an adjunct to methodical surgical technique and clip inspection. In a series of 337 aneurysms by Chiang and colleagues, findings on intraoperative angiography led to clip repositioning in 37 (11%) of aneurysm cases, with 22 (6.5%) being related to residual aneurysm, 10 (3%) parent vessel occlusion, and 5 (1.5%) a combination of residual aneurysm and vessel occlusion. Intraoperative angiography is particularly useful in large (>10 mm) and giant (>25 mm) aneurysms, as these lesions are more likely to be unsatisfactorily clipped and require revision than smaller aneurysms. It is also particularly useful in peri-clinoidal, basilar apex, and anterior communicating region aneurysms. Complications from intraoperative angiography include vessel occlusion, embolic events, and dissection. Chiang and colleagues reported an overall complication rate of 2.6%, with stroke in 1 of 303 (0.3%) operations, whereas Tang and colleagues reported 2 strokes in 517 aneurysms treated (0.4% stroke risk). The false-negative rate of intraoperative angiography ranges from 5% to 8%, but this number is limited by the lack of routine postoperative angiography in such cases. In general, intraoperative angiography is an important tool to evaluate for aneurysm residuals and vessel occlusion, and it is used routinely at the authors’ institution. Drawbacks are that, it is not available in all centers, it is an invasive technique, and it does not provide immediate feedback.
Intraoperative fluorescent angiography
Intraoperative fluorescent angiography has been a recent addition to the neurosurgical armamentarium to assess intraoperative blood flow dynamics, aneurysm sac obliteration, and vessel patency. Angiography using fluorescein sodium has been used by some groups in the treatment of cerebral aneurysms; however, the near-infrared dye indocyanine green (ICG) has emerged as the preferred agent for microsurgical use secondary to superior contrast of vessels during primary and subsequent dye applications.
ICG has been evaluated in several studies of aneurysm clipping, and integration of ICG near-infrared video technology into the surgical microscope has greatly facilitated its application. ICG use is noninvasive, safe, simple, and provides the surgeon with rapid feedback after clip application. ICG provides high resolution imaging of vessel anatomy, arterial and venous blood flow, and incomplete aneurysm clipping. Raabe and colleagues demonstrated that ICG angiography correlated with intraoperative or postoperative DS angiography in 90% of cases, and it provided significant information in 9% of cases, many of which led to clip repositioning. This technique is also unique in that it can visualize perforating arteries with good resolution.
ICG videoangiography is not without limitations and is not suited for all applications. Only vessels within the microscope field can be visualized with ICG. Blood clots (subarachnoid, intramural, or intraluminal), vessel wall calcification, atherosclerotic plaque, and arachnoid scarring can all obscure visualization. For the aforementioned reasons, ICG angiography is not currently a replacement for intraoperative DS angiography, but rather a complement to it.
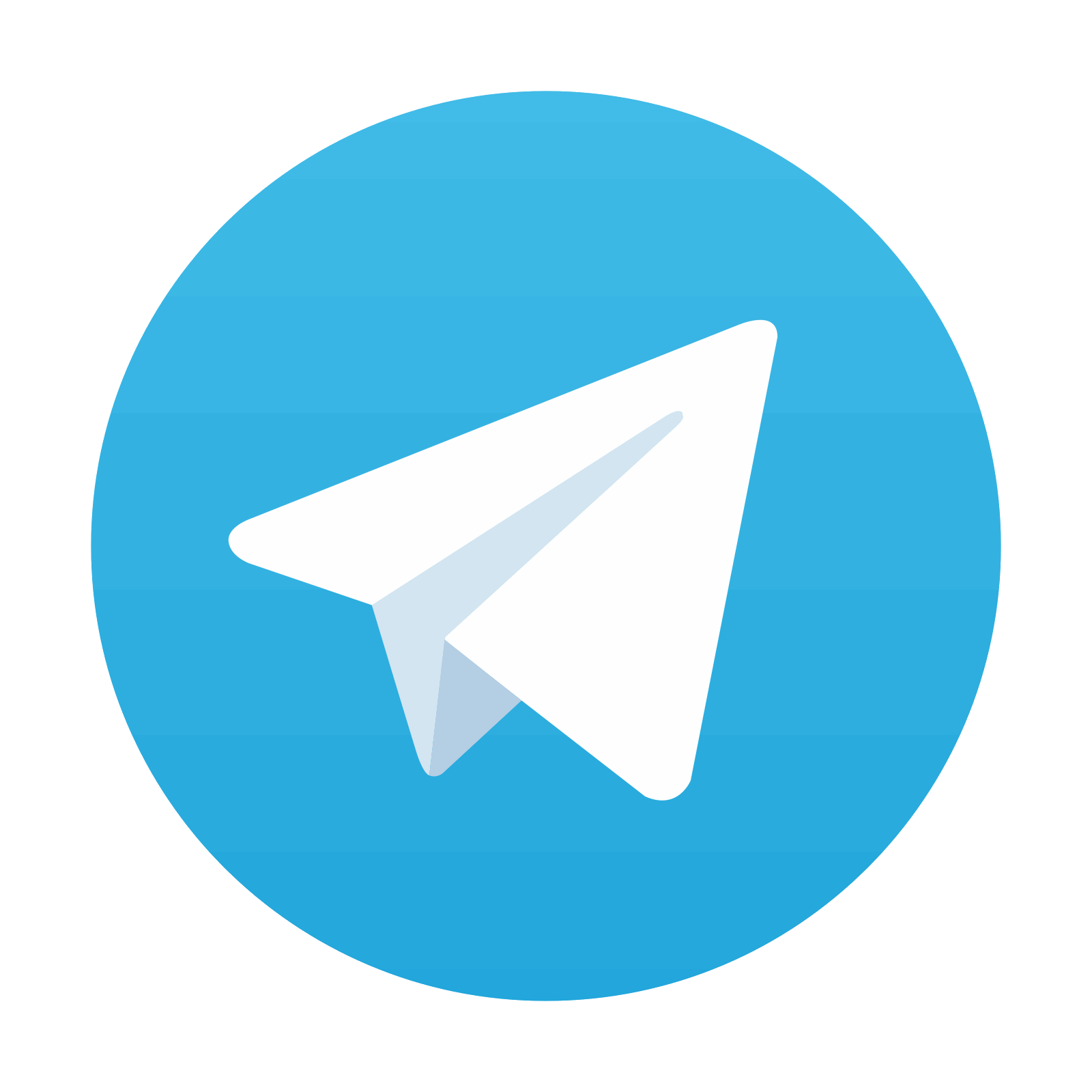
Stay updated, free articles. Join our Telegram channel

Full access? Get Clinical Tree
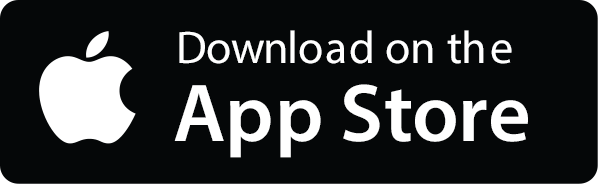
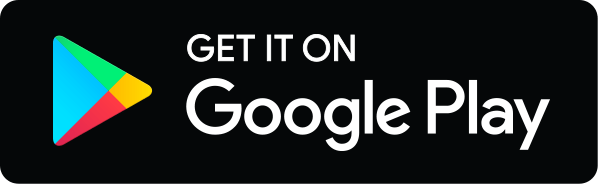