Fig. 10.1
Molecular mechanisms of IDH-associated tumorigenesis. Wild-type IDH1/2 (wtIDH1/2) converts isocitrate (generated through the citric acid (TCA) cycle) into α-KG, producing NADPH in the process. Mutant IDH1/2 (mutIDH1/2) converts isocitrate (generated through the citric acid (TCA) cycle) into α-KG, producing NADPH in the process. Epigenetic modifications result from inhibition of histone lysine demethylases (KDMs) and the 5-methyl cytosine hydroxylase TET2. Inhibition of prolyl-hydroxylation impairs collagen maturation. R-2-HG has been reported to activate the enzyme prolyl-hydroxylase 2 (PHD2) that inhibits hypoxia-inducible factor 1-alpha (HIF-1α) [37], although other studies suggest that it may be inhibited [24]. Mutant IDH may also change the cellular redox environment by altering the ratio of NADPH to NADP+. IDH3 has been omitted from this figure for simplicity
Molecular Alterations: Epigenetic Modifications
Numerous lines of evidence suggest that IDH mutations contribute to widescale epigenetic modifications. One subgroup of the 2-OG dioxygenase family that is potently inhibited by R-2-HG is the jumonji domain containing (Jmjc) family of histone lysine demethylases [26], responsible for the removal of methyl groups from lysine (K) residues of histone tails [27, 28]. Methylation of K residues on the tail of histone 3 (H3) is generally associated with either transcriptionally silenced (H3K9, H3K27, H3K20) or active (H3K4, H3K36, H3K79) regions of chromatin and thus repressed or activated gene expression, respectively [29]. These enzymes are particularly sensitive to inhibition by R-2-HG [26], and knockdown of Jmjd2c (also known as Kdm4c) is sufficient to phenocopy the effects of mutant Idh on adipocyte differentiation in 3T3-L1 cells [20].
R-2-HG also inhibits ten-eleven translocation 2 (TET2) [24]—a 2-OG-dependent dioxygenase that catalyzes the conversion of 5-methylcytosine (5mC) to 5-hydroxy-methylcytosine (5-hmC)—thus effectively reversing gene silencing by DNA methylation [30]. TET2 is itself mutated in myeloid malignancies [31], with mutations occurring in a mutually exclusive fashion to those of IDH [32]. This along with the observation of decreased 5-hmC levels in IDH-mutant AML [32], and the identification of TET2 as a candidate effector of mutant IDH in a short hairpin RNA library screen of 2-OG dioxygenases [21], suggests that this enzyme is an important mediator of the effects of IDH mutation, at least in myeloid malignancies. Indeed, IDH mutations in AML are associated with a hypermethylation phenotype, resulting from increases in global methylcytosine, where widespread DNA methylation results in epigenetic silencing of gene expression [33].
In glioma, mutant IDH establishes a widescale DNA methylation signature [34] known as glioma CpG island methylator phenotype (G-CIMP), observed in the majority of IDH-mutant tumors [20, 35]. In this study, ectopic expression of mutant IDH1 in human astrocytes led to a time-dependent build up of histone methylation at H3K9, H3K27, and H3K36, in addition to increases in global methylcytosine. Treatment of IDH1-mutant glioma cells with a mutant-specific IDH inhibitor decreases histone methylation both in vitro and in vivo, although this is not clearly linked to growth inhibition [36].
Molecular Alterations: Hypoxia and Metabolism
Despite its status as a 2-OG-dependent dioxygenase, prolyl-hydroxylase 2 (PHD2—also referred to as EGLN1) has been reported to be stimulated by mutant IDH [37]. This enzyme is responsible for regulating the expression of hypoxia-inducible factor (HIF)-1α through a post-translational modification involving hydroxylation of a proline residue in HIF’s alpha subunit, leading to targeting by the Von Hippel–Lindau (VHL) ubiquitin ligase complex under normoxic conditions [38, 39]. R-2-HG stimulates the activity of both PHD2 and the functionally similar PHD1 at tumor-relevant concentrations, resulting in a decrease in HIF1α and its target genes [37]. Notably, knockdown of HIF1 α and overexpression of PHD2 in IDH1 R132H -expressing astrocytes, both had transforming effects in soft agar colony formation assays. The same group noted that knockdown of PHD2 abrogated growth factor independence, and restored differentiation ability in IDH1 R132H leukemia cells [21]. However, other reports have suggested that R-2-HG inhibits PHD2-mediated HIF1α modification [24], which has been suggested as an explanation for the observed stabilization of Hif1 a, and increased transcription of downstream target genes, reported in an Idh1 R132H knock-in mouse [40].
Alterations in the TCA cycle resulting from IDH mutations would be expected to disrupt the balance of intracellular redox signaling. Due to impaired oxidative decarboxylation of isocitrate, IDH-mutant tumors exhibit alterations in the NADPH/NADP+ ratio [15, 41]. In addition to scavenging mitochondrial free radicals itself, NADPH is required as a hydrogen anion donor in the generation of the reduced form of glutathione (GSH), which constitutes the cells’ major defense mechanism against reactive oxygen species (ROS) [42]. IDH-mutant cells exhibit both reduced levels of NADPH and a corresponding reduction in GSH [43]. It has also been noted that glioblastoma cells ectopically expressing mutant IDH exhibit elevated levels of ROS, rendering them more sensitive to radiation [44, 45], which has been put forward as an explanation for the favorable therapeutic response [46, 47] and prognosis [48] of mutant IDH [49]. However, elevation of ROS levels has not been observed in transgenic models of mutant Idh [40, 50].
Recent reports suggest that alterations in metabolism and mitochondrial bioenergetics may render IDH-mutant cancer cells more sensitive to cell death. For instance, Tateishi et al. [51] found that mutant IDH1 lowers NAD+ levels, sensitizing cells to further NAD+ depletion that resulted in autophagy and cytotoxicity. While Chan et al. [52] found that R-2-HG-mediated inhibition of the mitochondrial enzyme COX rendered IDH-mutant AML cells sensitive to inhibition of the anti-apoptotic protein BCL-2. These studies raise the interesting notion that IDH mutations might endow tumor cells with an Achille’s heal that could form the basis of targeted therapy, rather than drugging the mutant protein itself.
The Contribution of Mutant IDH to Tumor Formation
Large-scale analyses of clinical data suggest that IDH mutations are an important event in tumorigenesis. Analyzing over 300 gliomas, Watanabe et al. [53] found that in the 51 cases with multiple biopsies, neither acquisition of a mutation in TP53 nor loss of 1p/19q occurred prior to a mutation in IDH1, highlighting its early occurrence. Whole-exome sequencing of matched biopsy pairs from glioma samples taken at initial diagnosis and at the time of recurrence showed that IDH1 R132H was the only mutation that was consistently present in both the initial and recurrent biopsy specimen [54]. In leukemia patients, IDH2 mutations were observed to occur in the absence of NPM1 c mutations in both mature and progenitor cell populations, suggesting that IDH2 mutation might occur as a pre-leukemic event [55].
Numerous studies have explored whether IDH mutations can transform nonmalignant cells of various lineages. Expression of mutant Idh in mouse myeloid progenitor 32D cells and primary mouse bone marrow cells impaired hematopoietic differentiation and increased stem/progenitor cell marker expression, suggesting a pro-leukemogenic effect [56]. A more recent study suggested that retrovirally mediated expression of mutant Idh2 R140Q in murine primary hematopoietic bone marrow stem and progenitor cell populations was sufficient to induce myeloproliferative-like neoplasms, T-cell lymphoma, or B-cell lymphoma, when transplanted into irradiated mice [57]. However, these hematological malignancies occurred at low penetrance and with long latency, suggesting that they did not arise solely due to mutant Idh2 expression. Expression of mutant Idh2 in a non-transformed mesenchymal multipotent mouse cell line (C3H10T) impaired their differentiation into adipocytic and chondrocytic lineages and resulted in loss of contact inhibition and tumor formation in vivo [58]. In immortalized human astrocytes, expression of mutant IDH, but not wild-type IDH or a catalytically inactive IDH mutant, resulted in anchorage-independent growth [37].
Further insights into the role of mutant IDH in tumor initiation have emerged from experiments with genetically engineered mice (Fig. 10.2). Tamoxifen-induced global expression of Idh2 R140Q or R172K resulted in cardiomyopathy, white matter abnormalities throughout the central nervous system, and muscular dystrophy, while mice engineered to express mutant Idh2 in specific tissues developed carcinomas, albeit with very long latencies [59]. In a hematopoietic tissue-specific model, mice that expressed a doxycycline-inducible Idh2 R140Q allele did not develop leukemia after 1 year of continuous doxycycline treatment [60]. The most common cancer-associated IDH mutation, IDH1 R132H , has also been inserted into the endogenous murine Idh1 locus, and expression of the mutant enzyme subsequently targeted to specific cell populations. Expression of Idh1 R132H in hematopoietic cells resulted in increased numbers of hematopoietic progenitors, but no overt leukemia [61]. Expression of Idh1 R132H in nestin-expressing neural stem cells resulted in perinatal lethality due to cerebral hemorrhage [40]. Expression of Idh1 R132H in GFAP-expressing astrocytes resulted in more subtle defects, including impaired collagen maturation and basement membrane function, likely due to R-2-HG-mediated inhibition of prolyl-/lysyl-hydroxylation of collagen proteins, but did not result in glioma formation. Tamoxifen-inducible expression of mutant Idh1 in chondrocytes resulted in the development of enchondromas—benign cartilage tumors that are precursors to malignant chondrosarcomas [62]. Doxycycline-induced expression of mutant Idh2 (Idh2 R140Q or Idh2 R172K) increased hepatocyte proliferation in a liver injury model, but was not sufficient to induce tumors [63].


Fig. 10.2
Transgenic models of mutant IDH. Transgenic knock-in systems have been used in an attempt to model Idh-mutant AML, glioma, chondrosarcoma, and IHCC, both alone and in combination of other genetic lesions
The current data from knock-in mice suggest that mutant Idh likely cooperates with other genetic or epigenetic events to initiate cancer. Using a mouse transplantation assay, Chaturvedi et al. [64] found that mutant IDH1 alone did not transform hematopoietic cells but greatly accelerated the onset of leukemia in cooperation with HoxA9. Chen et al. [65] applied a mosaic mouse modeling approach in which hematopoietic stem and progenitor cells (HPSC) from Flt3-ITD or Nras G12D mice were transduced with a retroviral vector expressing mutant Idh2 and then assessed for tumorigenic potential following transplantation into syngeneic recipient mice. Idh2 mutants were found to cooperate with Flt3 or Nras alleles to drive leukemia formation. This cooperativity between mutant Idh2 R140Q and other leukemia-relevant pathway alterations (e.g., Flt3-ITD or homeobox proteins HoxA9 and Meis1a) was confirmed in the aforementioned doxycycline-inducible transgenic model [60] and another mosaic mouse modeling approach [66]. Saha et al. [63] showed that Idh2 R172K cooperated with mutant KRAS (Kras G12D ) to induce intrahepatic cholangio-carcinomas.
In sum, these studies demonstrate that mutant IDH cooperates with other oncogenic events to initiate cancer, consistent with the finding that IDH-mutant human cancers typically harbor alterations in multiple other cancer genes. In glioma, for example, IDH mutations are associated with missense mutations in ATRX, TP53, and TERT (diffuse astrocytomas) or codeletion of chromosome arms 1p and 19q (oligodendrogliomas; [48, 67]). In AML patients with a normal cytogenetic profile, IDH mutations are associated with mutations in NPM1, FLT3-ITD, and DNMT3 A [68, 69].
Targeting Mutant IDH
Recent studies have begun to address the question of whether the activity of the mutant IDH enzyme remains important for the growth of IDH-mutant cancers once they are fully established. Studies in experimental cancer models suggest that indeed this is the case with the strongest evidence coming from leukemia models. In TF-1 human erythroleukemia cells, ectopic expression of mutant IDH promotes growth factor independence, a phenotype that can be reversed with a small molecule mutant IDH inhibitor [70, 71]. Ex vivo treatment of freshly isolated IDH-mutant leukemic blasts with a mutant-selective IDH inhibitor induces a cellular differentiation program [70]. Pharmacological inhibition of the mutant IDH enzyme blocks colony formation of human AML cells, but not of normal CD34(+) bone marrow cells [64]. In a genetically engineered leukemia model, pharmacologic or genetic inhibition of mutant Idh2 triggered the differentiation and death of AML cells [65], and doxycycline-induced silencing of mutant Idh2 similarly eliminated Idh2 R140Q /Hoxa9 or Idh2 R140Q /Meis1 a-driven leukemia cells [60].
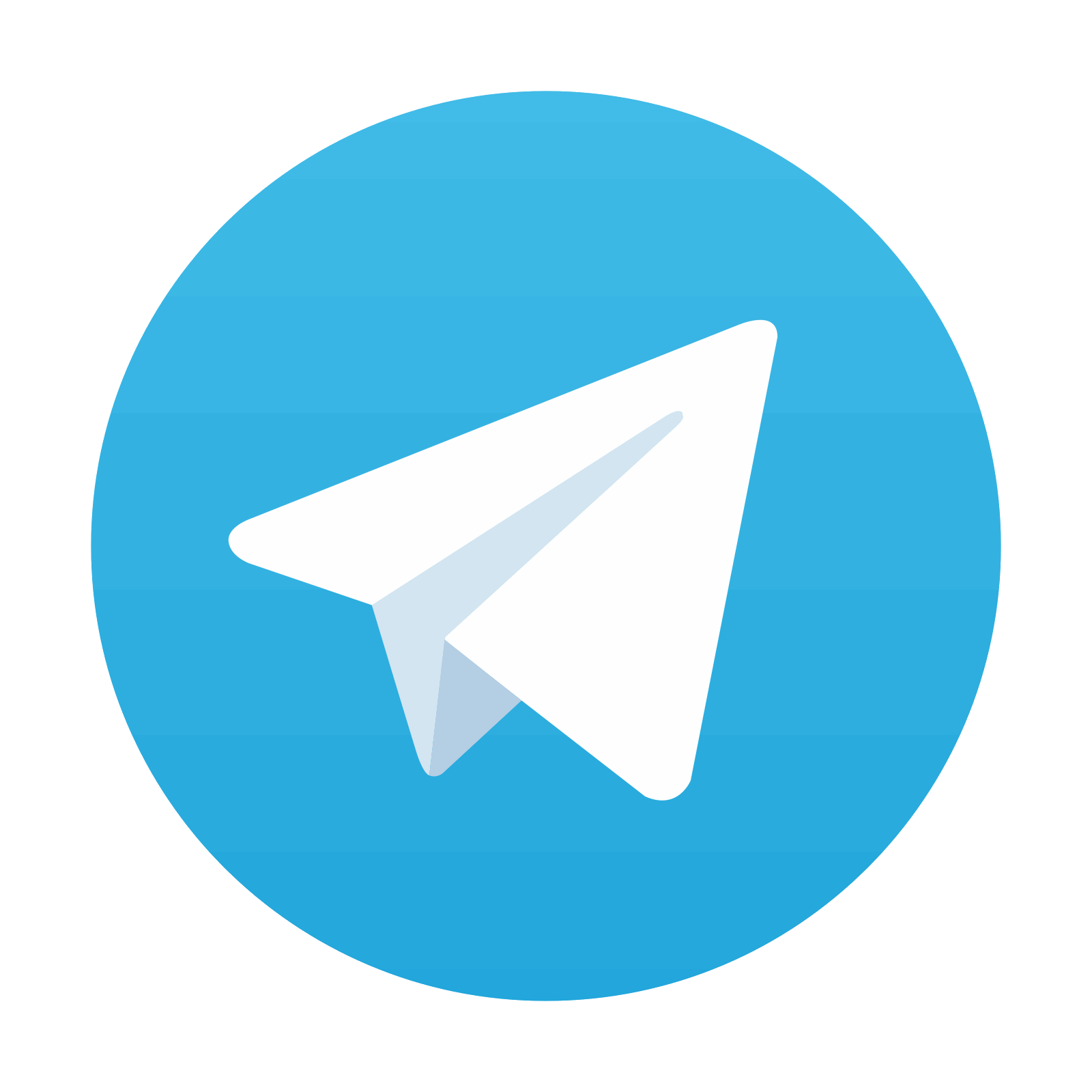
Stay updated, free articles. Join our Telegram channel

Full access? Get Clinical Tree
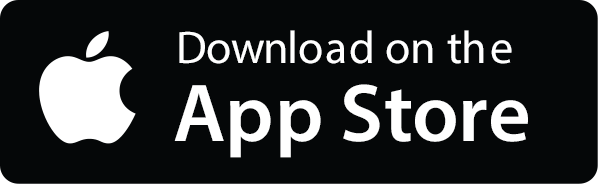
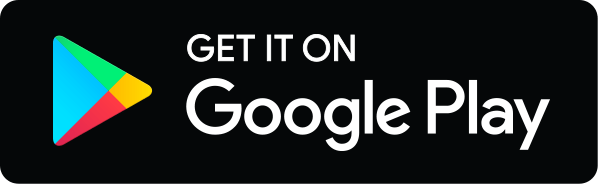