The Autonomic Motor System and the Hypothalamus
The Autonomic Motor System Mediates Homeostasis
The Autonomic System Contains Visceral Motor Neurons That Are Organized into Ganglia
Preganglionic Neurons Are Localized in Three Regions Along the Brain Stem and Spinal Cord
Sympathetic Ganglia Project to Many Targets Throughout the Body
Autonomic Behavior Is the Product of Cooperation Between All Three Autonomic Divisions
The Hypothalamus Integrates Autonomic, Endocrine, and Behavioral Responses
Magnocellular Neuroendocrine Neurons Control the Pituitary Gland Directly
Parvicellular Neuroendocrine Neurons Control the Pituitary Gland Indirectly
WHEN WE ARE FRIGHTENED OUR HEART races, our breathing becomes rapid and shallow, our mouth becomes dry, our muscles tense, our palms become sweaty, and we may want to run. These bodily changes accompanying fear are mediated by the autonomic motor system, which controls heart muscle, smooth muscle, and exocrine glands. The autonomic motor system is controlled by a central neuronal network that includes the hypothalamus.
As we shall learn in this and the next two chapters, the hypothalamus regulates the autonomic circuits so as to recruit appropriate physiological responses for specific emotions and to coordinate these physiological and emotional responses with other aspects of behavior to insure constancy of the internal environment (homeostasis). The hypothalamus contributes to the maintenance of homeostasis by acting on three major systems: the autonomic motor system, the endocrine system, and an ill-defined neural system concerned with motivation.
The autonomic motor system is distinct from the somatic motor system, which controls skeletal muscle. Nevertheless, to produce behaviors the somatic and autonomic motor systems must work together. Whereas neurons in the somatic motor system regulate contractions of striated muscles (see Chapter 34), the autonomic motor system regulates gland cells as well as smooth and cardiac muscle, maintains constant body temperature, and controls eating, drinking, and sexual behavior.
Although the autonomic motor system is largely involuntary, the behaviors controlled by it are tightly integrated with voluntary movements controlled by the somatic motor system. Running, climbing, and lifting are voluntary actions with metabolic requirements and thermoregulatory consequences that are automatically met by the autonomic system through changes in cardiorespiratory drive, cardiac output, regional blood flow, and ventilation. Autonomic behaviors similarly are linked to emotional arousal, stress, motivation, and defensive reactions. Feelings of fear, anger, happiness, and sadness have characteristic autonomic manifestations.
In this chapter we first examine the peripheral components of the autonomic system and then their role in mediating behaviors. We then explore how these “autonomic behaviors” are orchestrated through a central autonomic network in the brain stem and hypothalamus. We conclude by considering the role of the amygdala and specialized areas of cerebral cortex in coordinating autonomic function with motivation, volition, and emotion. The autonomic and hypothalamic mechanisms involved in emotion and motivation are examined in more detail in the next two chapters.
The Autonomic Motor System Mediates Homeostasis
In the middle of the 19th century Claude Bernard in Paris drew attention to the stability of the body’s internal environment, which includes the “fluid that surrounds and bathes all tissues,” during a broad range of behavioral states and external conditions. Bernard wrote: “The internal environment (le milieu interior) is a necessary condition for a free life.” Building on this idea, in the 1930s Walter B. Cannon introduced the concept of homeostasis to describe the mechanisms that maintain within a narrow physiological range the constancy of composition of the bodily fluids, body temperature, blood pressure, and other physiological variables.
As envisioned by Cannon, homeostatic mechanisms are adaptive because they extend the range of human behavior. For example, during exercise healthy people can increase their cardiac output four- to fivefold while maintaining blood pressure within a much narrower range. In the absence of these normal compensatory changes, blood pressure would increase in direct proportion to cardiac output, and the resulting increase in pressure would rupture blood vessels, perturb fluid composition, and alter the balance among the vascular, interstitial, and intracellular compartments. Increases in pressure of that proportion do not normally happen because the increase during exercise is curbed by an increase in diameter of the arteries that supply the working muscles and a resulting reduction of total vascular resistance to blood flow.
All homeostatic behavior, including control of the circulation, arises from neural modulation of the physiological properties of organ systems, mediated by hypothalamic control of the autonomic motor system and the endocrine system. We begin the discussion of these mechanisms by considering the peripheral components: the autonomic ganglia. The circuits of the ganglia connect with the spinal cord and brain stem and mediate simple reflexes that are the components of more complex behaviors.
The Autonomic System Contains Visceral Motor Neurons That Are Organized into Ganglia
Unlike the somatic motor system, in which the motor neurons are located in the ventral spinal cord and brain stem, the cell bodies of autonomic motor neurons are found in enlargements of peripheral nerves called ganglia.1 The autonomic ganglia contain motor neurons that innervate the secretory epithelial cells in glands or smooth and cardiac muscle.
Overall, the nervous system has many more autonomic than somatic motor neurons. In humans the entire spinal cord contains only approximately 120,000 somatic motor nerve cells, whereas the superior cervical ganglion alone contains approximately 900,000 autonomic motor neurons. Although the significance of this difference in numbers is uncertain, it may reflect the great diversity and complexity of autonomically controlled target tissues—the stomach, intestine, bladder, heart, lungs, and vasculature—as compared to the relative uniformity of skeletal muscle controlled by the somatic motor system. Most autonomic ganglia contain far fewer cells. For example, in the lungs and gastrointestinal tract of humans there are many microscopic ganglia, each with only tens to hundreds of neurons. These differences in number of cells are thought to reflect differences in the degree of control and the size of peripheral target fields.
Efforts to understand the principles of organization of autonomic ganglia began in 1880 in England with the work of Walter Gaskell and were later continued by John N. Langley. Their pioneering studies determined how individual autonomic ganglia are functionally regulated by central nerves, and in turn how the different ganglia regulate different peripheral targets. Gaskell and Langley stimulated autonomic nerves and observed the responses of end-organs (eg, vasoconstriction, piloerection, sweating, pupillary constriction). They used nicotine to block signals from individual ganglia to test interactions between ganglia. In the course of these studies Langley proposed that specific chemical substances must be released by neurons of the autonomic ganglia and that these substances act by binding to receptors on the target cells. These ideas set the stage for the later investigations of chemical synaptic transmission. Langley also distinguished the autonomic and somatic motor systems and in so doing created much of our current nomenclature.
Langley divided the autonomic system into three divisions: sympathetic, parasympathetic, and enteric. All neurons in sympathetic and parasympathetic ganglia are controlled by preganglionic neurons whose cell bodies lie in the spinal cord and brain stem. The pre-ganglionic neurons synthesize and release the neurotransmitter acetylcholine (ACh), which acts on nicotinic ACh receptors in postganglionic neurons, producing fast excitatory postsynaptic potentials and initiating action potentials that propagate to synapses with effector cells in end-organs (Figure 47–1). The sympathetic and parasympathetic systems are distinguished by five criteria:
Figure 47-1 Autonomic pathways have three basic cell types. Autonomic motor neurons lie outside the central nervous system in clusters or ganglia and are controlled by preganglionic neurons in the spinal cord and brain stem. Specialized neurons in ganglia regulate specific types of effector cells, such as smooth muscle, gland cells, and cardiac muscle.
1. The segmental organization of their preganglionic neurons in the spinal cord and brain stem
2. The peripheral locations of their ganglia
3. The types and locations of end-organs they innervate
4. The effects they produce on end-organs
5. The neurotransmitters employed by their post-ganglionic neurons
Preganglionic Neurons Are Localized in Three Regions Along the Brain Stem and Spinal Cord
The parasympathetic pathways arise from a cranial nerve zone in the brain stem and a second zone in sacral segments of the spinal cord (Figure 47–2). These parasympathetic zones surround a sympathetic zone that extends continuously in thoracic and lumbar segments of the cord.
The cranial parasympathetic pathways arise from preganglionic neurons in the general visceral motor nuclei of four cranial nerves: the oculomotor nerve (N. III) in the midbrain and the facial (N. VII), glossopharyngeal (N. IX), and vagus (N. X) nerves in the medulla. The cranial parasympathetic nuclei are described in Chapter 45 together with the mixed cranial nerves (such as the facial, glossopharyngeal, and vagus). The spinal parasympathetic pathway originates in preganglionic neurons in sacral segments two to four (S2–S4). The cell bodies of most of these neurons are located in intermediate regions of the gray matter, and their axons project in peripheral nerves through the ventral roots.
The sympathetic preganglionic cell column extends between the cervical and lumbosacral enlargements of the spinal cord, corresponding to the first thoracic segment (T1) and third lumbar segment (L3) in humans (Figure 47–2). Most of the cell bodies of sympathetic preganglionic neurons are located in the intermediolateral cell column, near the lateral margin of the spinal gray matter at the level of the central canal (Figure 47–3). Others are found in the central autonomic area surrounding the central canal and in a band connecting the central area with the intermediolateral cell column. The axons of preganglionic sympathetic neurons project from the spinal cord through the nearest ventral root and then run with small connecting nerves known as rami communicantes before terminating on postganglionic cells in the paravertebral sympathetic chain (Figure 47–3).
Figure 47-2 Sympathetic and parasympathetic divisions of the autonomic motor system. The sympathetic ganglia lie close to the spinal column and supply virtually every tissue in the body. Some tissues, such as skeletal muscle, are regulated only indirectly through their arterial blood supply. The parasympathetic ganglia are found in close apposition with their targets, which do not include the skin or skeletal muscle.
Sympathetic Ganglia Project to Many Targets Throughout the Body
The sympathetic motor system regulates systemic physiological parameters such as blood pressure and body temperature by influencing target cells within virtually every tissue throughout the body (Figure 47–2). This regulation depends on afferent pathways from the spinal cord and from supraspinal structures that control the activity of the preganglionic neurons. Preganglionic neurons form synapses with neurons in paravertebral and prevertebral sympathetic ganglia (Figure 47–3) that in turn form synapses with a variety of end-organs, including blood vessels, heart, bronchial airways, piloerector muscles, and salivary and sweat glands. The preganglionic neurons also synapse on chromaffin cells in the medulla of the adrenal gland (Figure 47–3), which secrete epinephrine (adrenaline) and norepinephrine (noradrenaline) into the circulation as hormones to act on distant targets.
Figure 47-3 The sympathetic outflow is organized into groups of paravertebral and prevertebral ganglia. The axons of preganglionic cells in the spinal cord reach postganglionic neurons by way of ventral roots and the paravertebral sympathetic chain. The axons either form synapses on postganglionic neurons in paravertebral ganglia or project out of the chain into splanchnic nerves. Preganglionic axons in the splanchnic nerves form synapses with postganglionic neurons in prevertebral ganglia and with chromaffin cells in the adrenal medulla.
The paravertebral and prevertebral sympathetic ganglia differ both in location and organization. Paravertebral ganglia are distributed segmentally, extending bilaterally as two chains from the first cervical segment to the last sacral segment. The chains lie lateral to the vertebral column at its ventral margin and generally contain one ganglion per segment (Figures 47–2 and 47–3). Two important exceptions are the superior cervical and stellate ganglia. The superior cervical ganglion is a coalescence of several cervical ganglia and supplies sympathetic innervation to the entire head, including the cerebral vasculature. The stellate ganglion, which innervates the heart and lungs, is a coalescence of ganglia from lower cervical segments and the first thoracic segment. These sympathetic pathways have an orderly somatotopic relation to one another, from their segmental origin in preganglionic neurons to their terminus in peripheral targets.
The prevertebral ganglia are midline structures that lie close to the arteries for which they are named (Figures 47–2 and 47–3). In addition to sending sympathetic signals to visceral organs in the abdomen and pelvis, these ganglia also receive sensory feedback from their end-organs.
Parasympathetic Ganglia Innervate Single Organs
In contrast to sympathetic ganglia, which regulate many targets and lie some distance from their targets close to the spinal cord, parasympathetic ganglia generally innervate single end-organs and lie near to or within the end-organs they regulate (Figure 47–2). In addition, the parasympathetic system does not influence skin or skeletal muscle except in the head, where it regulates vascular beds in the jaw, lip, and tongue.
The cranial and sacral parasympathetic ganglia innervate different targets. The cranial outflow includes four ganglia in the head. The oculomotor (III) nerve projects to the ciliary ganglion, which controls pupillary size and focus by innervating the iris and ciliary muscles. The facial (VII) nerve and a small component of the glossopharyngeal (IX) nerve project to the pterygopalatine (or sphenopalatine) ganglion, which promotes production of tears by the lacrimal glands and mucus by the nasal and palatine glands. Cranial nerve IX and a small component of nerve VII project to the otic ganglion, which innervates the parotid, the largest salivary gland. Nerve VII also projects to the submandibular ganglion, which controls secretion of saliva by the submaxillary and sublingual glands.
The vagus (X) nerve projects broadly to parasympathetic ganglia in the heart, lungs, liver, gall bladder, and pancreas. It also projects to the stomach, small intestine, and more rostral segments of the gastrointestinal tract. The caudal parasympathetic outflow supplies the large intestine, rectum, bladder, and reproductive organs.
The Enteric Ganglia Regulate the Gastrointestinal Tract
The entire gastrointestinal tract, from the esophagus to the rectum—and including the pancreas and gall bladder—is controlled by the system of enteric ganglia. This system, by far the largest and most complex division of the autonomic nervous system, contains as many as 100 million neurons in humans.
The enteric system has been studied most extensively in the small intestine of the guinea pig, where the diversity of enteric neurons and their organization has been analyzed in two interconnected plexuses, small islands of interconnected neurons. The myenteric plexus controls smooth muscle movements of the gastrointestinal tract; the submucous plexus controls mucosal function (Figure 47–4). Working together, this distributed network of ganglia coordinates the orderly peristaltic propulsion of gastrointestinal contents and controls the secretions of the stomach and intestines and other components of digestion. In addition, the enteric system regulates local blood flow and is modulated by external inputs from sympathetic prevertebral ganglia and from parasympathetic components of the vagus nerve.
Figure 47-4 Organization of the enteric plexuses in the guinea pig. The myenteric plexus and submucous plexus lie between the layers of intestinal wall (A and B). At least 14 types of neurons have been identified within the enteric system based on morphology, chemical coding, and functional properties (C). Four sets of motor neurons provide excitatory (+) and inhibitory (–) inputs to two smooth muscle layers. Three additional groups of motor neurons control secretions from the mucosa and produce vasodilation. The network also includes two major classes of intrinsic sensory neurons. (ACh, acetylcholine; ATP, adenosine triphosphate; CCK, cholecystokinin; CGRP, calcitonin gene-related polypeptide; DYN, dynorphin; ENK, enkephalin; GAL, galanin; NPY, neuropeptide Y; NO, nitric oxide; PACAP, pituitary adenylate cyclase-activating peptide; SOM, somatostatin; Tk, tachykinin; VIP, vasoactive intestinal peptide; 5-HT, serotonin.) (Parts A and B adapted, with permission, from Furness and Costa 1980; Part C reproduced, with permission, from Furness et al. 2004.)
Unlike the sympathetic and parasympathetic divisions of the autonomic nervous system, the enteric ganglia contain interneurons and sensory neurons in addition to motor neurons. This intrinsic neural circuitry can maintain the basic functions of the gut even after the splanchnic sympathetic and vagal parasympathetic pathways are cut. Through splanchnic nerves and the vagus nerve the gastrointestinal tract also sends sensory information about the physiological states of the tract to the spinal cord and brain stem.
Both the Pre- and Postsynaptic Neurons of the Autonomic Motor System Use Co-Transmission at Their Synaptic Connections
Synaptic transmission in the peripheral autonomic nervous system was originally thought to be a simple tale of two neurotransmitters, ACh and norepinephrine. According to this idea, all preganglionic neurons in the sympathetic and parasympathetic systems use ACh as their neurotransmitter, binding and exciting ionotropic nicotinic ACh receptors on ganglionic neurons and thus opening nonselective cation channels similar to the nicotinic ACh receptors at the neuromuscular junction. The resulting action potentials propagate to postganglionic synapses with end-organs in the periphery. At these synapses parasympathetic neurons release ACh that activates muscarinic receptors whereas sympathetic neurons release norepinephrine that activates α- and β-adrenergic G protein-coupled receptors. The consequences can be either excitatory or inhibitory, depending on the type of target cell and its receptors (Table 47–1).
In addition to acting on different receptors in different postsynaptic cells, one transmitter can activate two or more receptor types in the same postsynaptic cell. This principle was first discovered in sympathetic ganglia where ACh activates both nicotinic and muscarinic postsynaptic receptors to produce both a fast and slow excitatory postsynaptic potential (EPSP) (Figure 47–5A, and see Chapter 13). In some cases one transmitter can activate both a postsynaptic receptor as well as a receptor on the presynaptic terminals from which the transmitter was released. Such presynaptic responses can either reduce transmitter release (presynaptic inhibition) or enhance it (presynaptic facilitation). This mechanism, also widespread, was discovered at autonomic junctions with blood vessels where different types of α-adrenergic receptors are expressed by some pre- and postsynaptic cells (Figure 47–5B). This specialization of synaptic transmission in sympathetic and parasympathetic neurons leads to functional diversity in the regulation of end-organ function.
Figure 47-5 Synaptic transmission in the peripheral autonomic system.
A. In sympathetic ganglia ACh can activate both nicotinic and muscarinic receptors to produce fast and slow postsynaptic potentials, respectively.
B. At neurovascular junctions norepinephrine can simultaneously activate postsynaptic α1-adrenergic receptors to produce vasoconstriction and presynaptic α2-adrenergic receptors to inhibit further transmitter release.
C. Co-transmission involves the co-activation of more than one type of receptor by more than one transmitter. Parasympathetic postganglionic nerve terminals in the salivary glands release both ACh and vasoactive intestinal peptide (VIP) to control secretion. Autonomic synapses with end-organs sometimes employ more elaborate combinations, activating three or more receptor types.
Today we know that many transmitters are co-released at a single synapse, activating multiple receptor types and contributing to functional diversity. Many autonomic neurons release co-transmitters, often together with ACh or norepinephrine.
The cellular principles of co-transmission have been elucidated in part in a series of studies of paravertebral sympathetic ganglia in the bullfrog. These ganglia contain two major groups of neurons. Secreto-motor B neurons selectively innervate mucous glands in the skin, whereas vasomotor C neurons selectively innervate arteries that supply striated muscle and skin. In addition, each cell group receives input from a distinct group of preganglionic neurons in the spinal cord. These cell types differ from one another in part by expressing different neuropeptides. Preganglionic B cells selectively express calcitonin gene-related peptide (CGRP). Preganglionic C neurons express luteinizing hormone-releasing hormone (LHRH), also known as gonadotropin-releasing hormone (GnRH), and their postganglionic target cells express neuropeptide Y (Figure 47–6A).
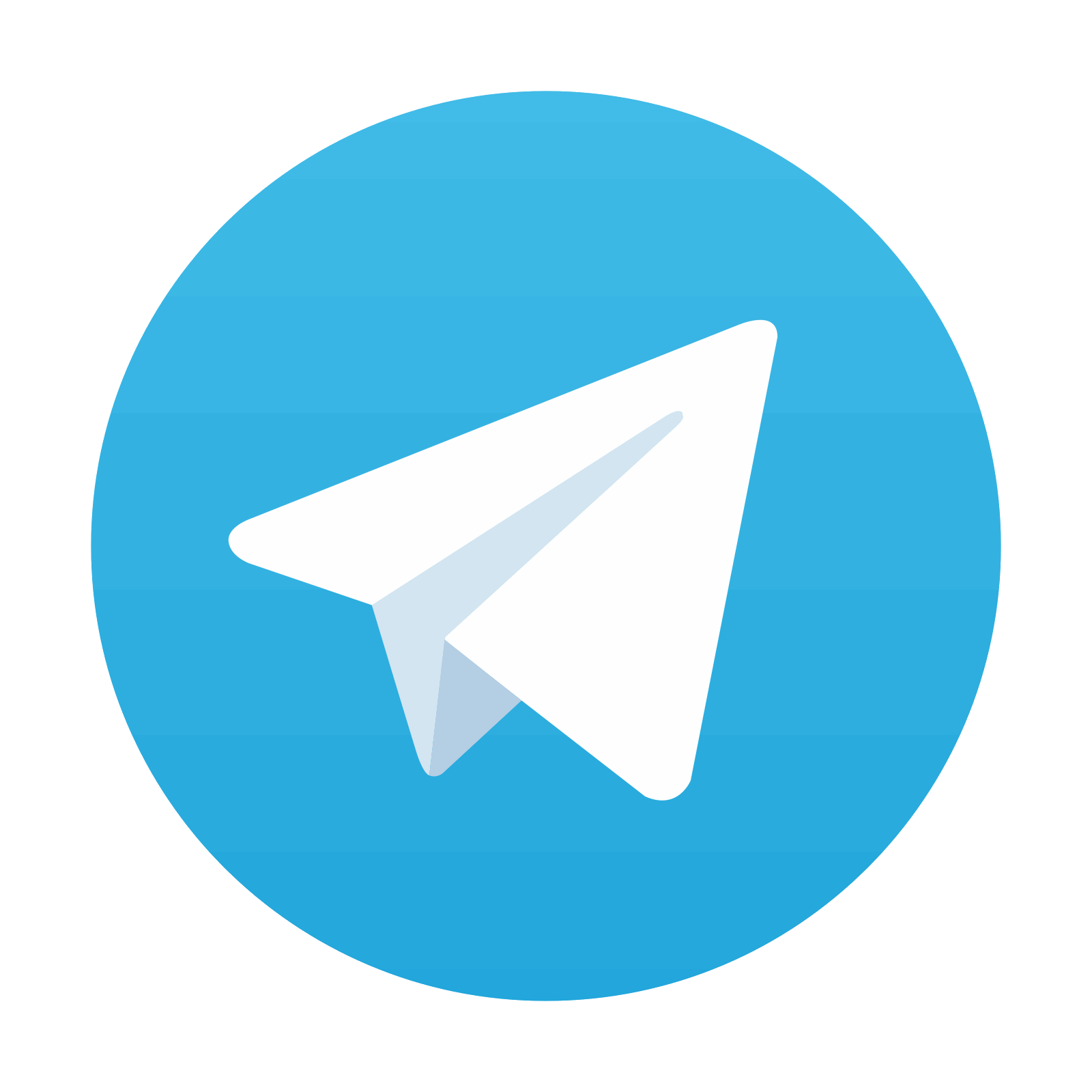
Stay updated, free articles. Join our Telegram channel

Full access? Get Clinical Tree
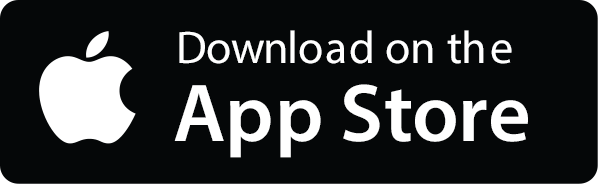
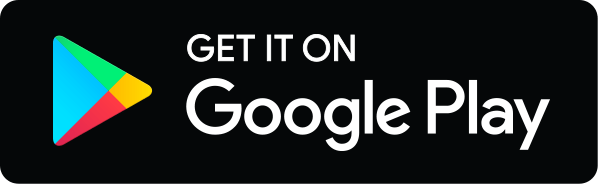