The Circle of Willis
Key Point
An understanding of the variant anatomy and flow dynamics of the Circle of Willis is crucial in many of the treatment decisions we make. Rare variations, on occasion, can represent considerable risks for particular patients.
Although a schema of the circle of Willis is easy to remember, there is enormous interindividual variation in the configuration of this arterial ring. Most intracranial saccular aneurysms occur on the circle of Willis or on the major branches close by. Of these, 82% to 85% occur anterior to a transverse line bisecting the posterior communicating arteries, with the single most common site being the region of the anterior communicating artery complex.
Anatomic Relationship to Surrounding Structures
The relationships of the circle of Willis and particularly those of the anterior cerebral artery and anterior communicating artery to surrounding cisternal and neural structures can be more easily envisioned if it is remembered that the optic structures and the infundibulum are subtended anteriorly through the ring of the circle (Figs. 9-1–9-3). The image of the arterial circle as a crab reaching around the optic chiasm with its claws may help to recall this relationship. The optic apparatus enters this ring at an angle that sweeps from posterosuperior to anteroinferior as the optic nerves make their way toward the optic canals. Optic nerve and chiasm displacement or compression with visual field deficits may be frequently seen with aneurysms of the anterior circle of Willis. In fact, Wilbrand’s knee (1), the genu of nasal fibers that loop into the posterior aspect of the contralateral optic nerve before continuing posteriorly in the optic tract, was described in 1915 in the setting of optic nerve compression by an aneurysm (although the existence of this loop is disputed (2,3)). The relationship of the optic chiasm to the anterior communicating artery is then easily remembered, as is the way that it derives perforator feeders from the inferior aspect of the anterior communicating artery and A1 segments. The tight apposition of the optic tracts and the basal forebrain can be easily remembered and underscore that the anterior choroidal artery must travel posteromedially under the optic tract before turning laterally into the choroidal fissure. The optic tracts will therefore receive perforators from the medial and superior aspects of the anterior choroidal artery and posterior communicating artery below.
The posterior communicating artery usually joins the posterior cerebral artery in front of the midbrain by leaving the posteromedial aspect of the internal carotid artery and ascending posteriorly, superiorly, and slightly medially. The oculomotor nerve lying between the posterior cerebral artery and superior cerebellar artery is a fairly constant relationship; therefore, it follows that the posterior communicating artery is usually superior or superomedial to the oculomotor nerve. Aneurysms in the region of the posterior communicating artery may present frequently with III nerve signs.
The position of the mammillary bodies in front of the midbrain allows one to recall that they are suspended above the posterior aspect of the ring of the circle of Willis. The posterior communicating artery sends between four and eight anterior perforating vessels superiorly toward the diencephalon. The sites of origin of these vessels are more frequently in the anterior half of the posterior communicating artery. The largest identifiable perforator in 80% of dissection specimens terminates between the mammillary bodies and the tuber cinereum. Therefore, this vessel is sometimes called the premamillary artery or thalamotuberal artery (4).
Variations in the Circle of Willis
The propensity of the circle of Willis for anomalies and variations is of interest in that the hemodynamic stresses associated with these variations combined with defects in the media at vessel junctions predispose to aneurysm formation (Figs. 9-4 and 9-5). Patients with aneurysms are more likely to have asymmetries or anomalies of the circle of Willis (5). Berry or saccular aneurysms form at a turn or curve in the artery, usually at a bifurcation point (6,7). The impact of hemodynamic stresses on bifurcations in the formation of aneurysms is reflected in the observation that aneurysms most often point in the direction in which flow would have traveled had its course not been deflected by the arterial wall. It is therefore cogent that in the setting of an anterior communicating artery aneurysm with asymmetry of the A1 segments, the aneurysm will point along the line of hemodynamic thrust from the dominant A1 segment.
In this circumstance, the aneurysm will project anteriorly and away from the side of the dominant A1, a relationship that is the case in the majority of such aneurysms. Some degree of A1 asymmetry is found in the majority of anterior communicating artery aneurysms (8). Similarly, the effects of hemodynamic stresses contribute, at least in part, to the formation of aneurysms at the sites of variant vessel origins. Aneurysms are associated with anomalous vessels or in situations with higher than usual rates of flow. Aneurysms are seen more frequently than one would expect by coincidence in the setting of variant carotid–vertebrobasilar anastomoses, arterial feeders of arteriovenous malformations, or after occlusion of cranial vessels by balloon closure, surgical ligation, or disease. For instance, an azygous anomaly of the anterior cerebral artery, seen in less than 1% of brains, is associated with a higher than usual rate of aneurysm formation (9,10,11).
In this circumstance, the aneurysm will project anteriorly and away from the side of the dominant A1, a relationship that is the case in the majority of such aneurysms. Some degree of A1 asymmetry is found in the majority of anterior communicating artery aneurysms (8). Similarly, the effects of hemodynamic stresses contribute, at least in part, to the formation of aneurysms at the sites of variant vessel origins. Aneurysms are associated with anomalous vessels or in situations with higher than usual rates of flow. Aneurysms are seen more frequently than one would expect by coincidence in the setting of variant carotid–vertebrobasilar anastomoses, arterial feeders of arteriovenous malformations, or after occlusion of cranial vessels by balloon closure, surgical ligation, or disease. For instance, an azygous anomaly of the anterior cerebral artery, seen in less than 1% of brains, is associated with a higher than usual rate of aneurysm formation (9,10,11).
Vessel Size
The circle of Willis is formed by a communication between the left and right carotid intracranial circulations at the anterior communicating artery and by bilateral communications between the carotid and vertebrobasilar circulations through the posterior communicating arteries. Because of the large number of embryologic steps involved in the evolution of the circle (12) and of each of its many components, variations and anomalies are common. Of these, the most common are those related to size of individual segments. Variability in the circle of Willis is not chaotic but has an order that conforms to hemodynamic principles, replicated in mathematical models (13,14). Therefore, for instance, an inverse relationship in size between the P1 segment of the posterior cerebral artery and the diameter of the posterior communicating artery is commonly seen. Similarly, the anterior communicating artery tends to be largest in the setting of unilateral hypoplasia of an A1 segment. Hillen et al. (15,16) demonstrated that the relationship curve of flow versus vessel diameter is steepest in the 0.5- to 2-mm range. This implies that compensatory hemodynamic effects can be predicted when a component of the circle of Willis is less than 2 mm in diameter.
A “typical” circle of Willis—in which all components are robust in diameter and symmetric—is unusual, being present
on macroscopic examination in only 21% of autopsy specimens (17). When bilateral small posterior communicating arteries are allowed as part of the normal symmetric configuration, only 30% would be “typical” (18); or, if asymmetry of vessel size is allowed with all vessels in the polygon measuring 1 mm or more, the proportion of “normals” is approximately 52% (19). A fetal-type posterior communicating artery, that is, a posterior communicating artery larger than the P1 segment of the posterior cerebral artery or one supplying the bulk of flow to that territory, is seen in 22% to 26% of cases. A 15% incidence of a more definitive fetal posterior cerebral artery is seen (19), whereas a hypoplastic posterior communicating artery is seen in 32% (4,20). When there is a fetal-type posterior communicating artery present, the P1 segment of the posterior cerebral artery is often longer and more tortuous. In these instances, the course of the fetal posterior communicating artery is more directly posterior off the internal carotid artery or posterolateral compared with the usual posteromedial course of the nonfetal posterior communicating artery. This alters the relationship of the posterior communicating artery to the oculomotor nerve. Instead of running superomedial to the oculomotor nerve, the fetal posterior communicating artery takes a more superior or superolateral course.
on macroscopic examination in only 21% of autopsy specimens (17). When bilateral small posterior communicating arteries are allowed as part of the normal symmetric configuration, only 30% would be “typical” (18); or, if asymmetry of vessel size is allowed with all vessels in the polygon measuring 1 mm or more, the proportion of “normals” is approximately 52% (19). A fetal-type posterior communicating artery, that is, a posterior communicating artery larger than the P1 segment of the posterior cerebral artery or one supplying the bulk of flow to that territory, is seen in 22% to 26% of cases. A 15% incidence of a more definitive fetal posterior cerebral artery is seen (19), whereas a hypoplastic posterior communicating artery is seen in 32% (4,20). When there is a fetal-type posterior communicating artery present, the P1 segment of the posterior cerebral artery is often longer and more tortuous. In these instances, the course of the fetal posterior communicating artery is more directly posterior off the internal carotid artery or posterolateral compared with the usual posteromedial course of the nonfetal posterior communicating artery. This alters the relationship of the posterior communicating artery to the oculomotor nerve. Instead of running superomedial to the oculomotor nerve, the fetal posterior communicating artery takes a more superior or superolateral course.
Complete absence of the posterior communicating artery is rare during dissection, 0.6% or less (19), although it is more common during angiographic interpretation not to be
able to see any evidence of a hemodynamically significant posterior communicating artery.
able to see any evidence of a hemodynamically significant posterior communicating artery.
Alone or in combination with other variations of the circle, a hypoplastic A1 segment may be seen in 12% of autopsy specimens, a hypoplastic P1 segment in 18%, and a hypoplastic anterior communicating artery in 20% (17).
Other Anomalies
Anomalies other than those related to vessel size fall into three general categories: Unusual vessels, duplications of normally present vessels, and origins of normally present vessels from unusual sites.
The anterior communicating artery complex is probably the single most common site in the circle of Willis for anomalous configurations. Duplications or fenestrations of this complex are seen in 9% to 40% of dissections (8,19). Alpers et al. (5,19) found a total incidence of duplications of vessels in the polygon of 19% (Figs. 9-6 and 9-7). A more unusual variant of the anterior communicating artery complex is the superior anterior communicating artery, which is occasionally seen more superiorly joining the A2 segments of the distal anterior cerebral arteries.
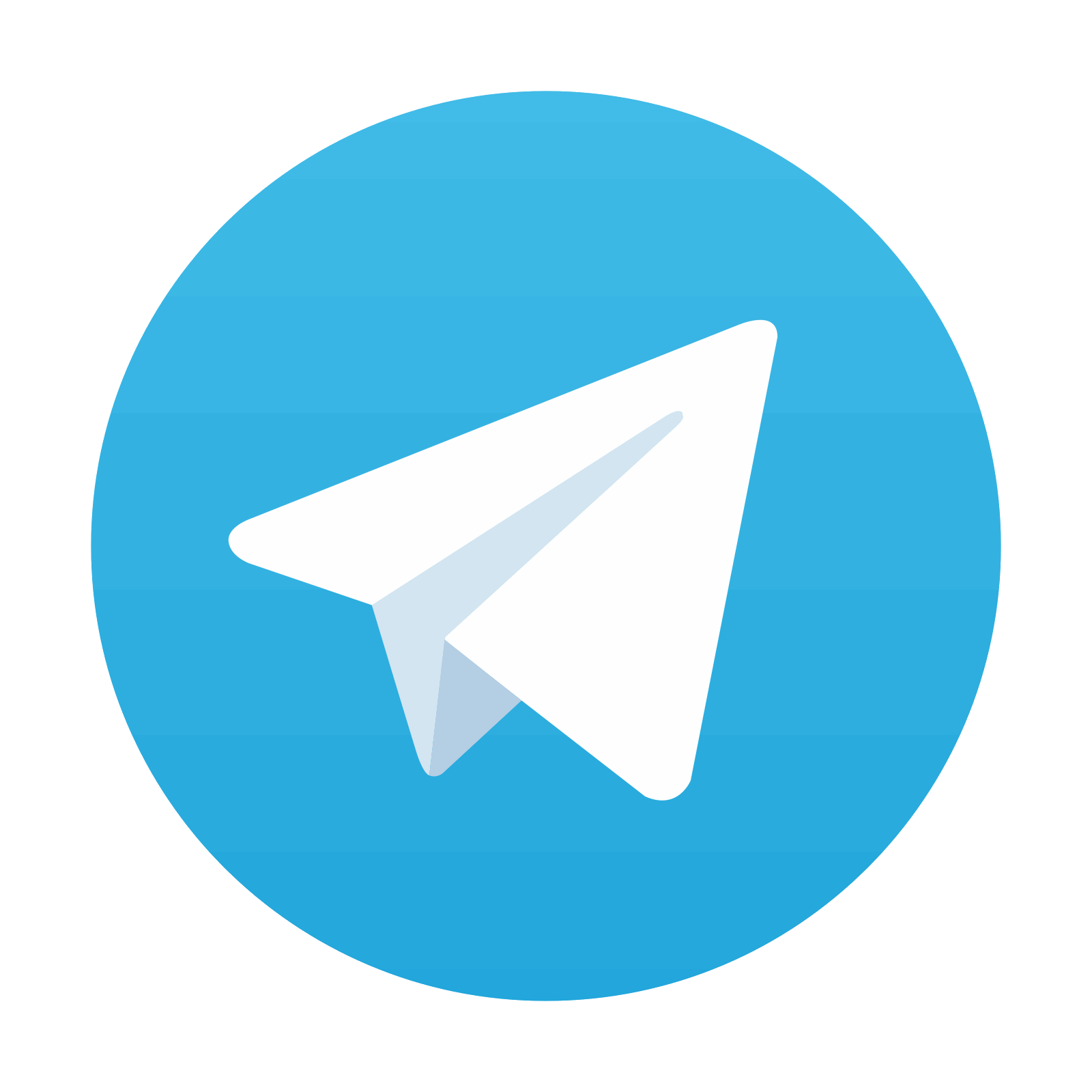
Stay updated, free articles. Join our Telegram channel

Full access? Get Clinical Tree
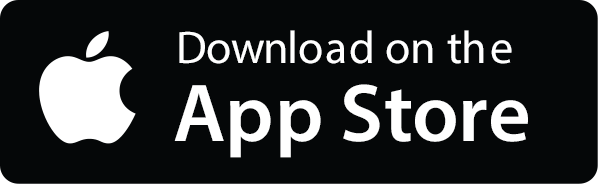
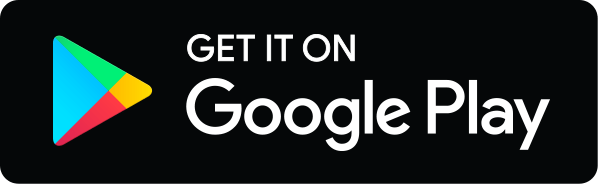